
Electrochemical behavior and polishing properties of silicon wafer in alkaline slurry with abrasive CeO2
SONG Xiao-lan(宋晓岚)1, XU Da-yu(徐大余)1, ZHANG Xiao-wei(张晓伟)1, SHI Xun-da(史训达)2, JIANG Nan(江 楠)1, QIU Guan-zhou(邱冠周)1
1. Department of Inorganic Materials, School of Resources Processing and Bioengineering,
Central South University, Changsha 410083, China;
2. Silicon Wafer Manufacture Department, Grinm Semiconductor Materials Co., Ltd.,Beijing 100088, China
Received 22 December 2006; accepted 22 August 2007
Abstract: The electrochemical behavior of silicon wafer in alkaline slurry with nano-sized CeO2 abrasive was investigated. The variations of corrosion potential (φcorr) and corrosion current density (Jcorr) of the P-type (100) silicon wafer with the slurry pH value and the concentration of abrasive CeO2 were studied by polarization curve technologies. The dependence of the polishing rate on the pH and the concentration of CeO2 in slurries during chemical mechanical polishing(CMP) were also studied. It is discovered that there is a large change of φcorr and Jcorr when slurry pH is altered and the Jcorr reaches the maximum (1.306 μA/cm2) at pH 10.5 when the material removal rate(MRR) comes to the fastest value. The Jcorr increases gradually from 0.994 μA/cm2 with 1% CeO2 to 1.304 μA/cm2 with 3% CeO2 and reaches a plateau with the further increase of CeO2 concentration. There is a considerable MRR in the slurry with 3% CeO2 at pH 10.5. The coherence between Jcorr and MRR elucidates that the research on the electrochemical behavior of silicon wafers in the alkaline slurry could offer theoretic guidance on silicon polishing rate and ensure to adjust optimal components of slurry.
Key words: Chemical mechanical polishing(CMP); material removal rate(MRR); electrochemical characteristics; slurry; abrasive CeO2
1 Introduction
Chemical mechanical polishing(CMP) was firstly put forward in 1991 by IBM and replaced the traditional method gradually, such as single chemical polishing and single mechanical polishing. CMP not only made a better surface, but also provided higher polishing flatness and was commonly recognized as the only and best method of achieving global planarization[1-5].
Colloid SiO2 aqueous suspension was the most widely used slurry in semiconductor industry, but the polishing rate of silica colloid was lower compared with other polishing oxides. Abrasive CeO2 polishing had high rate and it was widely adopted in the glass polishing, but its usage in CMP of silicon wafer was little found in literatures and the researches were few[6-9]. So in this work the polishing properties of silicon wafer in slurry with abrasive CeO2 are investigated and the research on the electrochemical behavior of silicon wafers in the slurry, which could offer theoretic guidance on the silicon corrosion, was conducted as well[10-11]. Through these studies we could realize the factors that influence corrosion rates of silicon wafers more clearly, and we could quantitatively elucidate how to control the polishing parameters, which was very important to improve polishing quality.
2 Experimental
2.1 Chemicals and samples
Chemicals included nano-sized CeO2 abrasive (average diameter 20 nm). The synthesis method of nano-sized CeO2 was described previously[12] and the TEM photograph of nano-sized CeO2 powders is shown in Fig.1. Surfactant (NaPO3)6, β-Hydroxyethyl Diamine (≥99%), CH3COOH (99.5%), HF, NH4F and deionised water were utilized. All of the chemicals were in analytically pure grade. The alkaline slurry was obtained by the following steps: Firstly, confect 225 mg/L solution of (NaPO3)6 (When CeO2 suspension contained 225 mg/L surfactant (NaPO3)6, the CeO2 particles came to the best dispersion in solution[13]); Secondly, add certain quality of abrasive CeO2 to the former solution and then disperse the slurry by ultrasonic wave (frequency 100 kHz) for 20 min; Finally, adjust the pH of slurry with β-Hydroxyethyl Diamine or CH3COOH.
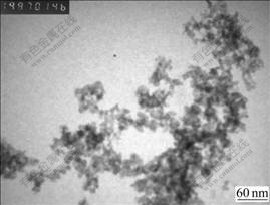
Fig.1 TEM photograph of nano-sized CeO2 powders
In the experiment, samples were czochralski grown, boron-doped P-Si (100) wafer (1-5 Ω?cm, orientation tolerance ±0.5?). The silicon working electrode prepared for electrochemical experiments was mounted in a cylindrical of Teflon holder and the exterior surface was limited with an “O”-ring with diameter of 1.33 cm.
2.2 Electrochemical measurements
For electorchemical measurements, native oxide needed to be removed before each measurement by soaking the wafer in 1 mol/L HF+2 mol/L NH4F for 1 min followed by rinsing with deionised water. The electrochemical experiments were carried out with the silicon working electrode, a platinum counter electrode and an Ag/AgCl reference electrode with a Luggin probe. The static electrochemical DC polarization measurements were conducted by a Potentiostat/Galvanostat of EG&G Model 273A, and the corrosion software of EG&G Model 352 was adopted for electrochemical calculations. During the potentiodynamic scan, the working electrode potential varied with a scan rate of 1 mV/s from -0.6 V to 0.4 V for measuring the corrosion current density and potential.
2.3 MRR of CMP measurements
The system of polishing experiment adopted is shown in Fig.2. Polishing time was 30 min; rotation rate was 200 r/min; imposed pressure was 0.10 kg/cm2 and temperature of the slurry was (30±1) ℃.
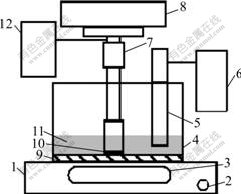
Fig.2 Schematic diagram of polishing experiment system: 1 Pressure sensor; 2 Button of elevator; 3 Display of pressure sensor; 4 Cell; 5 pH-meter; 6 Display of pH-meter; 7 Monitor of rotation rate; 8 Shelf; 9 Polishing pad; 10 Silicon wafer; 11 Slurry; 12 Rotation motor
The material removal rate(MRR) was determined by measuring the thickness of polished silicon as a function of polishing time.
3 Results and discussion
3.1 Dependence of polarization curves of P-Si (100) on pH value
Fig.3 shows the potentiodynamic polarization curves for P-Si (100) silicon tested in the slurries with 3% (mass fraction) CeO2 at various pH values. The corrosion current densities and the potentials of Fig.3 determined by the corrosion software of EG&G Model 352 are plotted in Fig.4. As can be seen from Fig.4, the corrosion potential decreased firstly with the increase of pH, and then increased to the maximum of 1.306 μA/cm2 at pH of 10.5; with the further increase of pH, the current density decreased to 1.175 μA/cm2 at pH of 11.5. This implied that the dissolution rate came to the fastest value in the slurry with pH 10.5.
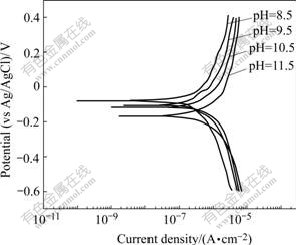
Fig.3 Potentiodynamic polarization curves of P-Si (100) in slurries with 3% CeO2 at various pH values
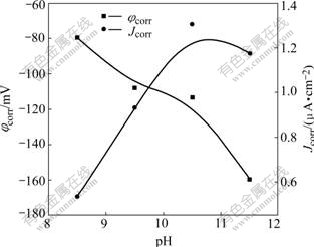
Fig.4 Variation of corrosion potential φcorr and corrosion current density Jcorr of P-Si (100) in slurries with 3% CeO2 as function of pH
The etching process of silicon was described as follows: two hydrogen molecules were released for each silicon atom dissolved and Si(OH)4 was regarded the primary reaction product[14-15]. The overall reaction was[16-17]
Si+4H2O
2H2+Si(OH)4
soluble silicate (1)
The process involved the hydroxide ion catalysed reaction of silicon and water to provide hydrogen and silicates. Namely, the dissolution rate was related to water and hydroxide ion concentration. GLEMBOCKI et al[18] proposed a model to explain the existence of dissolution rate peak based on the assumption that both free water and hydroxide ions were etching species. The reaction rate equation could be expressed as
(2)
According to the model, water was composed of free water and bound water. Free water and hydroxide ions were the major particles that participated in the chemical reaction. As pH increased, the OH- concentration increased, while H2Ofree concentration decreased. So these two competing effects made the peak in the corrosion rate.
3.2 Dependence of CMP material removal rate on pH
As shown in Fig.5, a pronounced increase of MRR with the increase of pH was observed, and then MRR reached a plateau at pH 10.5 and finally kept approximately horizontal. The sharp increase of MRR could be ascribed to hydroxide ion concentration increase and resulted in corrosion rate enhancement as the foregoing analysis. On the other hand, pH had an important influence on CeO2 particles dispersion in solution. Fig.6 shows the Zeta potential of particle surface at various pH values. A minimum negative Zeta potential around pH 10.5 indicated that there was a superior dispersion of abrasive CeO2 in alkaline solution with pH 10.5. Stable dispersion of abrasive CeO2 in slurry helped to improve material removal rate and polishing silicon surface quality. Subsequently, the plateau state in the removal rate was due to limited mechanical effect. As it is well known, chemical mechanical polishing consists of two aspects: chemical corrosion effect and mechanical abrasion effect. When the chemical corrosion effect surpasses the mechanical abrasion effect, MRR depends on the latter. Only under fit cooperation between them can it be expected to reach an optimal MRR level[19].
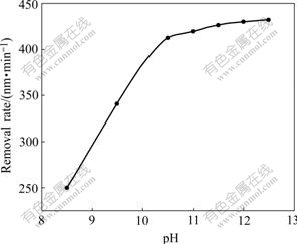
Fig.5 Dependence of CMP material removal rate on pH
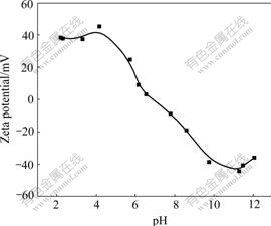
Fig.6 Dependence of Zeta potential of CeO2 particle surface on pH
3.3 Dependence of polarization curves of P-Si (100) silicon on concentration of CeO2
Fig.7 presents the potentiodynamic polarization curves for P-Si (100) in the slurries with different concentrations of abrasive CeO2 at pH 10.5. As can be seen from Fig.7, the shape of the polarization curves with different concentrations was very similar. From Fig.8, the corrosion potential ranged from -67.8 mV with 1% CeO2 to -163.7 mV with 4% CeO2, indicating concentration of CeO2 had some influence on the film thickness. The corrosion current density increased gradually from 0.994 μA/cm2 with 1% CeO2 to 1.304 μA/cm2 with 3% CeO2; then with the further increase of concentration, the current density leveled off to 1.313 μA/cm2.
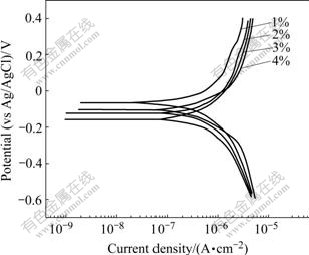
Fig.7 Potentiodynamic polarization curves of P-Si (100) in slurries with different concentrations of abrasive CeO2 at pH 10.5
Fig.8 Variation of corrosion potential and corrosion current density of P-Si (100) in slurries at pH 10.5 as function of CeO2 abrasive concentration
As well known, amphoteric oxide CeO2 would have a hydration in slurry:
CeO2+H2O→Ce(OH)4 (3)
Because of the complexation of CeO2, the corrosion product
could be transformed into [Ce(SiO3)3]2- and the equation could be written as
Ce(OH)4+
→[Ce(SiO3)3]2-+H2O (4)
which resulted in accelerated removal of
from silicon surface and promoted corrosion rate and corrosion current density. When CeO2 concentration reached 3%, because of sufficient complexation, the corrosion current density leveled off.
3.4 Dependence of CMP material removal rate on concentration of CeO2
The abrasive, during the polishing process, affected the mechanical abrasion of the corrosion layer. As shown in Fig.9, with increase of abrasive concentration, MRR rose from 225 nm/min with 1% CeO2 to 406 nm/min with 3% CeO2 and 412 nm/min with 4% CeO2. The functional relation of MRR of silicon in CMP process with the concentration of CeO2 in slurries at pH 10.5 presented the same tendency as that of the corrosion current density with the concentration of CeO2 indicated in Fig.8 under the same condition. This indicates that abrasive CeO2 accelerates reaction product silicates departure from silicon plane by complexation of Ce(OH)4.
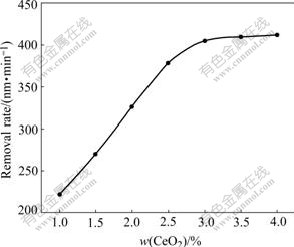
Fig.9 Dependence of CMP material removal rate on concentration of CeO2 in slurries at pH 10.5
4 Conclusions
1) The results of polarization curves reveal that pH of the slurry has a pronounced influence on the silicon wafer corrosion. The current density increases with the increase of pH and reaches the maximum (1.306 μA/cm2) at pH 10.5, then with the further increase of pH, the current density decreases to 1.175 μA/cm2 at pH 11.5. This implies that the corrosion rate comes to the fastest value in the slurry at pH 10.5.
2) The corrosion current density increases gradually from 0.994 μA/cm2 with 1% CeO2 to 1.304 μA/cm2 with 3% CeO2, and then with the further increase of concentration, the current density levels off. This implies that there is an optimal dissolution rate in the slurry with 3% CeO2 at pH 10.5.
3) In the MRR of CMP experiment, a marked increase of MRR with the increase of pH is observed and MRR reaches a plateau (412 nm/min) at pH 10.5. Dependence of MRR on concentration of CeO2 is the same as that on pH and reaches considerable value of 406 nm/min in the slurry with 3% CeO2.
Acknowledgements
This work was carried out with the support of the Instrumental Analyzing & Testing Centre in School of Resources Processing and Bioengineering, Central South University, China. We thank Mrs. NIE for her help and useful advice.
References
[1] MALIK F, HASAN M. Manufacturability of the CMP process [J]. Thin Solid Films, 1995, 270(1/2): 612-615.
[2] PARKER J. Next-generation abrasive particles for CMP [J]. Solid State Technology, 2004, 47(12): 30-32.
[3] GERFRIED Z. Advanced CMP technology and applications [J]. Proc Electrochem Soc, 2004, 11: 235-243.
[4] LUO J F, DORNFELD D A. Material removal mechanism in chemical mechanical polishing: Theory and modelling [J]. IEEE Transaction on Semiconductor Manufacturing, 2001, 14(2): 112-133.
[5] CHIU Jian-bin, YU Cheng-ching, SHEN Shih-haur. Application of soft landing to the process control of chemical mechanical polishing [J]. Microelectronic Engineering, 2003, 65(3): 345-356.
[6] PARK S W, KIM C B, KIM S Y. Design of experimental optimization for ULSI CMP process applications [J]. Microelectronic Engineering, 2003, 66(1/4): 488-495.
[7] COOK L M. Chemical process in glass polishing [J]. Journal of Non-crystalline Solids, 1990, 120(1/2): 152-171.
[8] XU Jin, LUO Jian-bin, LU Xin-chun, ZHANG Chao-hui, PAN Guo-shun. Progress in material removal mechanisms of surface polishing with ultra precision [J]. Chinese Science Bulletin, 2004, 49(16): 1687-1693.
[9] CHEN Hue-ying, CHANG Hung-yi. Synthesis of nanocrystalline cerium oxide particles by the precipitation method [J]. Ceramics International, 2005, 31(6): 795-802.
[10] SONG Yan-yan, ZHANG Yu, XIA Xing-hua. Influence of formaldehyde on the electrochemistry and chemical etching of silicon in alkaline solutions [J]. Acta Chimical Sinica, 2004, 62(15): 1415-1418. (in Chinese)
[11] HE Han-wei, HU Yue-hua, HUANG Ke-long. Corrosion electrochemical mechanism of chemical mechanical polishing of copper in K3[Fe(CN)6] solution [J]. Trans Nonferrous Met Soc China, 2002, 12(1): 178-182.
[12] SONG Xiao-lan, QIU Guan-zhou, QU Peng, YANG Zhen-hua, WU Xue-lan, WANG Hai-bo. Synthesis and performance of CeO2 nanocrystallines by precipitation method [J]. Journal of Hunan University (Natural Science), 2004, 31(6): 13-17. (in Chinese)
[13] SONG Xiao-lan, QIU Guan-zhou, YANG Zhen-hua, QU Peng. Dispersion behaviors of CeO2 nanoparticles in water suspension [J]. Chinese Journal of Rare Metals, 2005, 29(2): 167-172. (in Chinese)
[14] ALLONGUE P, COSTA-KIELING V, GERISCHER H. Etching of silicon in NaOH solutions (II): Electrochemical studies of n-Si (111) and (100) and mechanism of the dissolution [J]. Journal of the Electrochemical Society, 1993, 140(4): 1018-1026.
[15] BRESSERS P M M C, PLAKMAN M, KELLY J J. Etching and electrochemistry of silicon in acidic bromine solutions [J]. Journal of Electroanalytical Chemistry, 1996, 406(1/2): 131-137.
[16] GLEMBOCKI O J, PALIK E D. Hydration model for the molarity dependence of the etch rate of Si in aqueous alkali hydroxides [J]. Journal of the Electrochemical Society, 1991, 138(4): 1055-1063.
[17] PALIK E D, BERMUDEZ V M, GLEMBOCKI O J. Ellipsometric study of orientation-dependent etching of silicon in aqueous KOH [J]. Journal of the Electrochemical Society, 1985, 132(4): 871-884.
[18] GLEMBOCKI O J, PALIK E D, DE GUEL G R, KENDALL D L. Hydration model for the molarity dependence of the etch rate of Si in aqueous alkali hydroxides [J]. Journal of the Electrochemical Society, 1991, 138(4): 1055-1063.
[19] QIN K, MOUDGIL B, PARK C W. A chemical mechanical polishing model incorporating both the chemical and mechanical effects [J]. Thin Solid Films, 2004, 446(2): 277-286.
Foundation item: Project(2005DFBA028) supported by the International Cooperation of Science and Technology Ministry of China; Project(LA07023) supported by the National Undergraduate Innovative Experiment Plan
Corresponding author: SONG Xiao-lan; Tel: +86-731-8830346; E-mail: xlsong@hnu.cn
(Edited by YANG Bing)