
Electrodeposition behavior and characteristics of
Ni-carbon nanotube composite coatings
Sung-Kyu KIM, Tae-Sung OH
Department of Materials Science and Engineering, Hongik University, Seoul 121-791, Korea
Received 21 April 2010; accepted 10 September 2010
Abstract: Ni-CNT (carbon nanotube) composite coatings were processed by electrodeposition and their hardness and corrosion characteristics were investigated with variations of CNT concentration in an electrolyte solution and electrodeposition current density. With increasing the CNT concentration in the electrodeposition bath and the current density, more CNTs are incorporated into Ni matrix. Hardness values of the Ni-CNT coatings are irrelevant to the CNT concentration in the solution, the current density, and current mode, implying poor adhesion of CNTs to Ni matrix. With increasing the CNT content in the coating, the corrosion resistance of the Ni-CNT composite coating becomes inferior due to the porous microstructure.
Key words: composite coating; carbon nanotube; electrodeposition
1 Introduction
Electrodeposition of composite coatings has been widely investigated for better wear resistance and dispersion strengthening[1-3]. Among various process technologies for composite coatings, electrodeposition has advantages such as cost-effectiveness relative to spray and sputtering processes[4]. Conventionally, ceramic powders such as alumina, silicon carbide, and diamond were used as reinforcements for Ni-based nanocomposite coatings[1, 3, 5-8].
Recently, carbon nanotube (CNT) has been applied as a new reinforcement material for composite coatings due to its excellent mechanical properties and high thermal conductivity[4, 9-11]. As Ni exhibits high wear resistance, good ductility, and ferromagnetism, Ni-CNT composite coatings have potential applications not only for wear-resistance coatings and microelectromechanical systems (MEMS), but also for corrosion-resistance coatings[4, 9-11].
Characteristics of Ni-CNT composite coating depend on the CNT content in the composite coatings, which is in turn related to the electrodeposition current density as well as the CNT concentration in electrolyte solution. In this study, Ni-CNT composite coatings were processed by electrodeposition, and their hardness and corrosion characteristics were investigated with variations of the CNT concentration in an electrolyte solution and the electrodeposition current density.
2 Experimental
Ni-CNT composite coatings were electrodeposited in a sulfate Watts bath with the following compositions: 260 g/L nickel sulfate (NiSO4?6H2O), 45 g/L nickel chloride (NiCl2?6H2O), 15 g/L boric acid (H3BO3), and 0.5 g/L saccharine. To improve CNT dispersion, 2.5 g/L sodium dodecylsulfate (SDS) and 7.5 g/L chexadecyl- phosphocholine (HPC) were added into the electrodeposition solutions[12]. CNTs of 10-15 nm in diameter, produced by CVD, were used to form the Ni-CNT electrodeposition solutions. As the length of the as-received CNTs, about 20 μm, was too long for coelectrodeposition, CNTs were cut to a length less than 5 μm by ball-milling with ZrO2 balls for 24 h at 200 r/min. The mass ratio of CNT to ZrO2 was kept as 1:25 in an alumina jar.
After producing the Ni-CNT electrodeposition solutions with CNT contents of 0, 1, 2, 5, 10 g/L, Ni-CNT composites of 50 μm in thickness were electrodeposited on Cu substrates of 2 cm×2 cm in dimensions at a current density of 40-120 mA/cm2. During electrodeposition of a Ni-CNT composite, the bath was maintained at 40 °C with mechanical stirring of 500 r/min.
Vickers hardness values of the Ni-CNT composite coatings were measured with an applied force of 1 N Corrosion characteristics of the Ni-CNT composite coatings were evaluated in 3.5% NaCl (mass fraction) solution. A Ni-CNT composite coating of 1 cm2 surface area was exposed to 3.5% NaCl solution and its polarization curve was recorded at potentials ranging from -0.25 V to 1.0 V at a sweep rate of 5 mV/s. Morphologies of the Ni-CNT composites were observed by using field emission scanning electron microscopy (FESEM).
3 Results and discussion
Fig.1 shows the FESEM micrographs of the Ni-CNT composites electrodeposited in the electro- deposition solutions with the CNT concentrations of 0-10 g/L at a current density of 40 mA/cm2. The Ni-CNT composites were etched in nitric acid to remove the Ni of the composite surface to facilitate the observation of the CNTs incorporated in the composites. With increasing the CNT concentration in the electro- deposition bath up to 5 g/L, more CNTs were incorporated into Ni matrix. However, microstructure of the Ni-CNT composite became porous with increasing the CNT concentration in the bath beyond 2 g/L.
Fig.2 illustrates the FESEM micrographs of the Ni-CNT composites electrodeposited at current densities of 40-120 mA/cm2 in the electrodeposition solution with the CNT concentration of 10 g/L. With increasing the current density, the CNT concentration in the coating substantially increased due to the fact that the force pulling Ni+2 ions adsorbed on the CNT surface to the cathode became larger at higher current density[13-15].
Vickers hardness values of the Ni-CNT coatings electrodeposited in the solutions with the CNT concentrations of 0-10 g/L are illustrated in Fig.3 as a function of the electrodeposition current density. The hardness value of the Ni-CNT composite coating was not changed much not only with increasing the current density, but also with increasing the CNT concentration in the electrodeposition solution.
The Ni-CNT composite coatings were electrodeposited with a pulse current mode shown in Fig.4, and their hardness values were compared in Fig.5 with those prepared at DC current density of 80 mA/cm2. TAN et al[16] reported that the Ni-CNT coating processed with a pulse current had higher hardness than that prepared with a DC current. However, the results in Fig.5 exhibited no noticeable change in the hardness values of the Ni-CNT composite coatings electrodeposited with the pulse plating, compared with those processed by DC electroplating. As shown in Figs.3 and 5, hardness values of the Ni-CNT composite coatings were irrelevant to variations of the CNT concentration in the solution, the electrodeposition current density, and the current mode, implying poor adhesion of CNTs to Ni matrix in the composite coatings. It has been also reported that the weak CNT-matrix interaction caused the epoxy-CNT composites weaker or barely stronger than the epoxy itself[17-18]. Poor adhesion of CNTs to Ni matrix might be caused by insufficient surface treatment of CNTs[19].
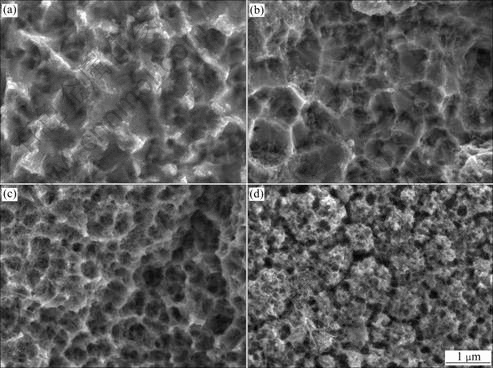
Fig.1 FESEM micrographs of Ni-CNT nanocomposites electrodeposited in solution containing CNT concentration of 1 g/L (a), 2 g/L (b), 5 g/L (c) and 10 g/L (d)
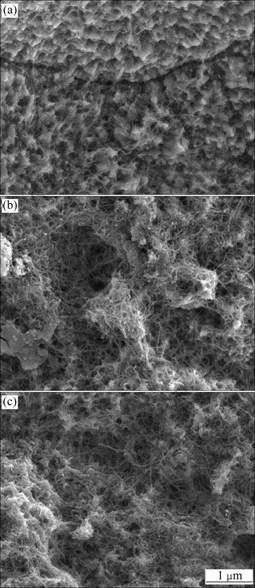
Fig.2 FESEM micrographs of Ni-CNT nanocomposites electro- deposited at current density of 40 mA/cm2 (a), 80 mA/cm2 (b) and 120 mA/cm2 (c)
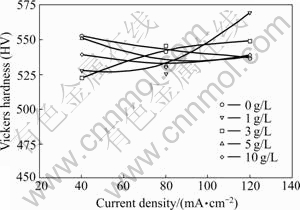
Fig.3 Vickers hardness of Ni-CNT coating as function of electrodeposition current density with different CNT concentrations
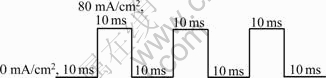
Fig.4 Pulse current mode used for electrodeposition of Ni-CNT composite coating
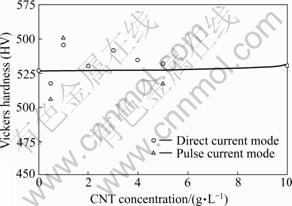
Fig.5 Vickers hardness of Ni-CNT coatings electrodeposited by pulse current mode and DC current mode of 80 mA/cm2, as function of CNT concentration
Fig.6 shows the polarization curves and the corrosion potentials of the Ni-CNT composite coatings. With increasing the CNT content in the coating, the corrosion potential moved towards more negative values, indicating inferior corrosion resistance of the Ni-CNT composite coating with higher CNT content.
To understand the cause for deterioration of the corrosion resistance, corrosion tests were performed for the Ni-CNT coatings electrodeposited at different current densities in the solution containing CNTs of 5 g/L.
As shown in Fig.7, the corrosion potential became more negative with increasing the current density, showing that the Ni-CNT composite electrodeposited at higher current density became less corrosion-resistant. Contrary to our results, it has been reported for Ni-CNT and Zn-CNT coatings that the corrosion resistance was improved with incorporation of CNTs into the coating by filling surface defects such as micro holes and crevices with CNTs[19-20]. The Zn coating has been also reported to become less porous with incorporation of CNTs[19]. As shown in Figs.1 and 2, however, microstructure of the Ni-CNT coatings became porous with increasing the CNT concentration in the solution or the electrodeposition current density. The porous microstructure of the Ni-CNT coatings in this study might be caused by poor adhesion of CNTs to Ni matrix. The more the porous in microstructure was, the more the surface area was exposed to the corroding medium, deteriorating the corrosion characteristics. The adhesion enhancement of CNTs to Ni matrix thus would play an important role for improvement of mechanical and corrosion characteristics of the Ni-CNT composite coating.
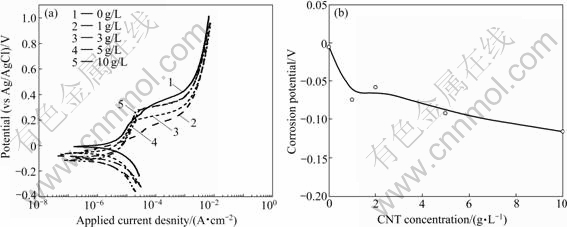
Fig.6 Polarization curves (a) and corrosion potentials (b) of Ni-CNT composite coatings electrodeposited in solutions with different CNT concentrations
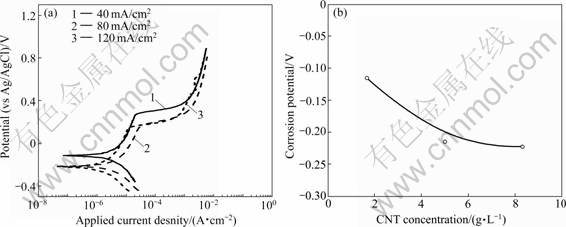
Fig.7 Polarization curves (a) and corrosion potentials (b) of Ni-CNT composite coatings electrodeposited at various current densities
4 Conclusions
1) With increasing the CNT concentration in the electrodeposition bath up to 5 g/L, more CNTs are incorporated into Ni matrix. The CNT concentration in the composite coating substantially increases with increasing the current density from 40 mA/cm2 to 120 mA/cm2.
2) The hardness values of the Ni-CNT coatings are irrelevant to the CNT concentration in the electrodeposition solution, the electrodeposition current density, and current mode, implying poor adhesion of CNTs to Ni matrix.
3) With increasing the CNT content in the coating, the corrosion potential moves towards more negative values, indicating inferior corrosion resistance of the Ni-CNT composite coating with higher CNT content. As the Ni-CNT composite coating becomes more porous with increasing the CNT content, more surface area is exposed to the corroding medium, deteriorating the corrosion resistance of the Ni-CNT coating.
Acknowledgement
This work was supported by the Center for Electronic Packaging Materials of Korea Science Engineering Foundation.
References
[1] ZIMMERMANN A F, PALUMBO G, AUST K T, ERB U. Mechanical properties of nickel silicon carbide nanocomposites [J]. Mater Sci Eng A, 2002, 328: 137-146.
[2] CHOA Y H, YANG J K, KIM B H, JEONG Y K, LEE J S, NAKAYAMA T, SEKINO T, KIIHARA N. Preparation and characterization of metal/ceramic nanoporous nanocomposite powders [J]. J Magn Mater, 2003, 266(1-2): 12-19.
[3] CHANRONG C X, GUO X, LI F, PENF D, PENG G. Preparation of asymmetric Ni/ceramic composite membrane by electroless plating [J]. Colloid Surfaces A, 2001, 179: 229-235.
[4] CHEN X H, CHENG F Q, LI S L, ZHOU L P, LI D Y. Electrodeposited nickel composites containing carbon nanotubes [J]. Surf Coat Technol, 2002, 155(2-3): 274-278.
[5] SHARMA G, YAGAVA R K, SHARMA V K. Characteristics of electrocodeposited Ni-Co-SiC composite coating [J]. Bull Mater Sci, 2006, 29(5): 491-496.
[6] BALARAJU J N, SESHADRI S K. Synthesis and corrosion behavior of electroless Ni-P-Si3N4 composite coatings [J]. J Mater Sci Lett, 1998, 17(15): 1297-1299.
[7] SZCZYGIEL B, KOLODZIEJ M. Composite Ni/Al2O3 coatings and their corrosion resistance [J]. Electrochim Acta, 2005, 50(20): 4188-4195.
[8] TAMAM H B, ZEROUAL L, CHALA A, RAHMANE S, NOUVEAU C. Microhardness and corrosion behavior of Ni-SiC electrodeposited coatings [J]. Plasma Process Polym, 2007, 4: S618-S621
[9] SHEN G R, CHENG Y T, TSAI L N. Synthesis and characterization of Ni-P-CNT’s nanocomposite film for MEMS applications [J]. IEEE Trans Nanotechnol, 2005, 4(5): 539-547.
[10] CHEN X, ZHANG G, CHEN C, ZHOU L, LI S, LI X. Carbon nanotube composite deposits with high hardness and high wear resistance[J]. Adv Eng Mater, 2003, 5(7): 514-518.
[11] CHEN X H, CHEN C S, XIAO H N, LIU H B, ZHOU L P, LI S L, ZHANG G. Dry friction and wear characteristics of nickel/carbon nanotube electroless composite deposits [J]. Tribology Int, 2006, 39(1): 22-28.
[12] JEON Y S, BYUN J Y, OH T S. Electrodeposition and mechanical properties of Ni-carbon nanotube nanocomposite coatings [J]. J Phys Chem Solids, 2008, 69(5-6): 1391-1394.
[13] WU G, LI N, ZHOU D, MITSUO K. Electrodeposited Co-Ni-Al2O3 composite coatings [J]. Surf Coat Technol, 2004, 176: 157-164.
[14] SHRESTHA N K, SAJI T. Non-aqueous composite plating of Ni-ceramic particles using ethanol bath and anti-wear performance of the coatings [J]. Surf Coating Technol, 2004, 186(3): 444-449.
[15] VERRCHEN P M, SHAO I, SEARSON P C. Particle codeposition in nanocomposite films [J]. J Electrochem Soc, 2000, 147: 2572-2575.
[16] TAN J, YU T, XU B, YAO Q. Microstructure and wear resistance of nickel-carbon nanotube composite coating from brush plating technique [J]. Tribology Lett, 2006, 21(2): 107-111.
[17] BIERCUK M J, LIAUNO M C, RADOSAVLJEVIC, HYUN J K, JOHNSON A, FISCHER J E. Carbon nanotube composites for thermal management [J]. Appl Phys Lett, 2002, 80: 2767-2769.
[18] AJAYAN P, SCHADLER L, GIANNARIS C, RUBIO A. Single- walled carbon nanotube-polymer composites: Strength and weakness [J]. Adv Mater, 2000, 12(10): 750-753.
[19] PRAVEEN B M, VENKATESHA T V, NAIK Y A, PRASHANTHA K. Corrosion behavior of Zn-TiO2 composite coating [J]. Surf Coat Technol, 2007, 201: 5836-5842.
[20] CHEN X H, CHEN C S, XIAO H N, CHEN F Q, ZHANG G, YI G J. Corrosion behavior of carbon nanotubes-Ni composite coating [J]. Surf Coat Technol, 2005, 191: 351-356.
(Edited by LI Xiang-qun)
Corresponding author: Tae-Sung OH; Tel: +82-2-320-1655; E-mail: ohts@hongik.ac.kr