J. Cent. South Univ. Technol. (2009) 16: 0247-0252
DOI: 10.1007/s11771-009-0042-2

Electrochemical behavior of wound supercapacitors with propylene carbonate and acetonitrile based nonaqueous electrolytes
ZHANG Zhi-an(张治安), LAI Yan-qing(赖延清), LI Jie(李 劼), LIU Ye-xiang(刘业翔)
(School of Metallurgical Science and Engineering, Central South University, Changsha 410083, China)
Abstract: The activated carbon wound supercapacitors with TEABF4/propylene carbonate (PC) and TEABF4/acetonitrile (AN) electrolytes were prepared. The effects of the electrolyte and temperature on the capacitance behavior were investigated by cyclic voltammetry (CV) and constant current charge-discharge. Compared with the PC-based supercapacitor, the AN-based supercapacitor has higher capacitance and lower equivalent serial resistance (RES) at discharge currents ranging from 5 to 1 000 mA and 25 ℃. Moreover, temperature effects are more prominent for PC-based supercapacitor than for AN-based supercapacitor. When the measurement temperature ranges from 60 ℃ to -40 ℃ the capacitance changes from 5.1 to 2.5 F and RES changes from 135 to 876 m? for the PC-based supercapacior, while the AN-based supercapacitor shows less change in capacitance and RES. Thus AN-based supercapacitor exhibits excellent power characteristics and temperature property.
Key words: supercapacitor; electrolyte; capacitance; equivalent serial resistance; temperature
1 Introduction
Supercapacitors, also called electrochemical double layer capacitors (EDLC), have gained significant attention during recent years and have potential to replace batteries in certain applications or complement batteries or fuel as power boosters. Supercapacitors are charge-storage devices that have a greater power density and longer cycle life compared with batteries, and a higher energy density compared with conventional capacitors. Owing to their unique energy-storage performance, supercapacitors have attracted considerable attention in potential applications including vehicles, such as electric vehicles and trains, electric generation systems, such as solar cells, wind power and portable devices, such as mobile phones, laptop computers and memory devices [1-4].
There are three different types of supercapacitor: carbon/carbon, metal oxide, and electronically conducting polymer [5-7]. Since activated carbon electrode materials enjoy many advantages of excellent electrochemical stable property, raw material abundance, low cost, environment friendness, etc [8-10]. In carbon supercapacitors, the electric charge is stored by double- layer mechanism at the interface of high surface area activated carbon electrode/electrolyte. According to the electrolyte used, two major types of supercapacitors can be distinguished, with aqueous electrolytes and nonaqueous
electrolytes, respectively [11-12]. Supercapacitor with aqueous electrolytes exhibits superior power density at lower energy density, whereas supercapacitor with nonaqueous electrolytes exhibits superior energy density due to the large voltage window of nonaqueous electrolytes. Thus, the choice of a nonaqueous electrolyte solution is of technical importance in the development of high performance supercapacitor. Typical nonaqueous electrolytes used in practical supercapacitor devices today consist of a quaternary ammonium salt, such as tetraethylammonium tetrafluoroborate (TEABF4), in a suitable organic solvent, such as acetonitrile (AN) or propylene carbonate (PC) [13]. However, a systematic comparison between the EDLC performances of the two electrolytes for the same carbon based wound supercapacitor under similar experimental conditions is still lacking.
The main aim of this paper is to study the electrochemical behavior the carbon-based wound supercapacitor in nonaqueous electrolyte and the influence of the solvent characteristics on the region of ideal polarizability of supercapacitor, capacitance and equivalent serial resistance, and rate discharge performance. A direct comparison between two identical supercapacitors built with AN and PC based electrolytes is performed. The electrochemical characteristics of supercapacitors are obtained using AN and PC as solvents for TEABF4 salt.
2 Experimental
2.1 Preparation of carbon-based wound supercapacitors
The active electrode material is an activated carbon with specific surface area of 1.8×103 m2/g, tap density of 0.4 g/cm3. 80% (mass fraction) of activated carbon, 10% of acetylene black, 10% of polyvinylidene fluoride (PVDF) binder were firstly mixed and stirred in the stirred vessel until homogeneous black slurry was obtained, then the slurry was spread out onto a 30 μm-thickness aluminum film current collector. In the vacuum condition, the electrode sheet was dried at 20 ℃ for 12 h. Then, the electrode sheet was pressed by the roller until its thickness was less than 180 μm, and the lead wire was attached to the electrode sheet by spot-welt. Two electrode sheets were separated by a 30 μm-thickness separator, and the electrode sheets and separator were wound and assembled in the wrapping machine. The core packages were put into electrolyte in the inert atmosphere and put onto rubber packing, which were placed into the aluminum case with the size of d12 mm×20 mm, and sealed on the self-made sealer. The image of core and cell of the carbon-based supercapacitor is shown in Fig.1. The core packages were dried for 12 h prior to impregnation with electrolytic solution. The TEABF4 salt was selected owe to usually good solubility in nonaqueous solvents and moderately good conductivity. Two electrolytes were used to perform in this study: 1 mol/L TEABF4/PC and 1 mol/L TEABF4/AN electrolyte. The electrolytes used were stored over molecular sieves before use. Before the measurement, the supercapacitors were kept at the room temperature for 48 h.
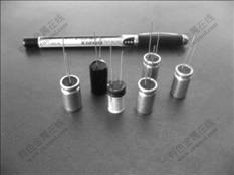
Fig.1 Image of core and cell of supercapacitor
2.2 Electrochemical characterization of supercapacitors
The charge-discharge performance of super- capacitors were measured by a CT2001A-5V rapid sampling battery testing system (LAND, China) at the voltage of 0-2.5 V and different currents. Cyclic voltammetry (CV) measurements were performed at the scan rate ranging from 20 to 200 mV/s using M273A EG&G electrochemical system (Princeton Applied Research, USA). Tests were conducted at temperature between -40 and 60 ℃. Temperature control was provided by a GDH-2005C high-low temperature test chamber (Shanghai JINGHONG, China). Prior to a test the supercapacitor was allowed to equilibrate at a given temperature for 3 h. Equivalent serial resistance (RES) and capacitance(C) of the supercapacitor are determined according to the charge-discharge curves:
RES = ?E/?I (1)
C =It/?E (2)
where I is the discharge current, t is the discharge time, and ?E is the voltage drop in discharge, excluding the portion of IR drop.
3 Results and discussion
3.1 Feature of electrolyte
PC and AN are commonly used as solvents for supercapacitor. The relative permittivity of PC is as high as 64.92 at 25 ℃, and the viscosity is also high (2.530 mPa?s at 25 ℃). However, AN shows low viscosity (0.341 mPa?s at 25 ℃) for its relative low permittivity (35.941 at 25 ℃). Moreover, the boiling point (b.p. 81.60 ℃) of AN is lower than that of PC (b.p. 241 ℃) [14]. Solutions of TEABF4 salt in AN and PC were used as electrolytes for the carbon supercapacitor in this work. Table 1 lists the features of the AN-based electrolyte and PC-based electrolyte. From Table 1, the conductivity of the AN-based electrolyte is relatively high (4.8 S/m at 25 ℃), while the conductivity for PC-based electrolyte is 1.2 S/m. And there is a difference in density between the two electrolytes.
Table 1 Parameters of AN-based and PC-based electrolytes
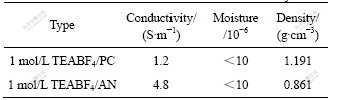
3.2 Cyclic voltammetry characterization
Fig.2 shows the CV curves for the AN-based supercapacitor and the PC-based supercapacitor at different scan rates of 20, 50, 100, 150 and 200 mV/s and 25 ℃, and the voltage window is between 0 and 2.5 V. For the AN-based supercapacitor, the profiles of CV curves show ideal rectangular-like shape at the different scan rates, even the high scan rate of 200 mV/s, and the response current increases linearly with the increase of the scan rate. This shows the typical capacitance behavior, high reversibility and small internal resistance, exhibiting excellent power characteristics. For the PC-based supercapacitor, the profiles of CV curves show ideal rectangular-like shape at low scan rate. At higher scan rate, the distortion effect can be seen in the region of potential switchover. With the increase of the scan rate, distortion effect of the CV curves becomes more obvious [15-16]. The effect depends on the solvent since the electrolyte with PC has lower conductivity and higher viscosity values. In addition, the current of PC-based supercapacitor is smaller than that of AN-based supercapacitor at the same scan rate. As mentioned above, the viscosity for PC is higher than that for AN. With the increase of the scan rate for the PC-based supercapacitor, the electrolyte ionic transport is slow, the interface between carbon and electrotype is not completely immersed, and the surface of activated carbon is partly utilized, resulting in low capacitance. And the phenomena are more obvious at higher scan rate.
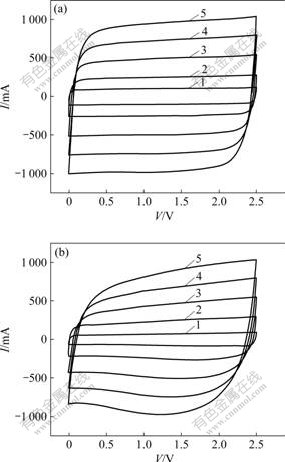
Fig.2 Cyclic voltammgrams for AN-based supercapacitor (a) and PC-based supercapacitor (b) at different scan rates: 1—20 mV/s; 2—50 mV/s; 3—100 mV/s; 4—150 mV/s; 5—200 mV/s
Fig.3 shows the CV curves for the AN-based supercapacitor and PC-based supercapacitor at the scan rate of 20 mV/s and 25 ℃, and the voltage window of CV curve varying from 0 to 2.5 V. It can be seen that the curves show no obvious redox peaks and the current is nearly constant over most of the voltage window range, which indicates that the supercapacitors are charged and discharged mainly by double-layer mechanism at a constant rate over the complete cycle. Moreover, the CV curves show a fairly rectangular mirror image corresponding to a typical capacitive behavior and a rapid current response on voltage reversal at each end voltage, which means a fast and reversible charge-discharge process. Compared with the AN-based supercapacitor and the PC-based supercapacitor, it can be seen that the profile of CV curves is incompletely similar, and the response current of AN-based electrolyte supercapacitor is larger than that of AN-based electrolyte supercapacitor. Moreover, for the PC-based supercapacitor, the response current can slightly increase with the increase of the potential, as the currents are the highest at the largest cell potential during change process. For the AN-based supercapacitor, however, the currents during change and discharge process are nearly constant.
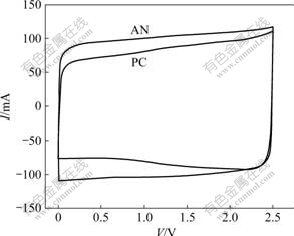
Fig.3 CV curves for AN-based and PC-based supercapacitors at scan rate of 20 mV/s
High electrochemical reversibility is one of the basic requirements for supercapacitor since the reversibility is the predominant factor influencing the power property of supercapacitor. The electrochemical reversibility for the PC-based supercapacitor and the AN-based supercapacitor was investigated by varying the upper potential limit of voltage window. Typical CV curves of the AN-based supercapacitor measured at the scan rate of 50 mV/s and 25 ℃ are shown in Fig.4. Perfect rectangular shape of all CV curves is observed at 50 mV/s. Note that all voltammetric response currents approximately follow the same trace on the reverse scan of all CV curves in Fig.4. These results indicate good electrochemical reversibility of the AN-based supercapacitor, resulting in high-power characteristics of the supercapacitor.
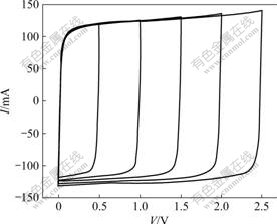
Fig.4 Effect of voltage windows on CV curves for AN-based supercapacitor at scan rate of 50 mV/s
3.3 Charge-discharge performance
It is well known that, for supercapacitor application, capacitance and RES are two important parameters that can influence the performance of the supercapacitor such as the power density and energy density. To measure the capacitance and RES of the AN-based supercapacitor and the PC-based supercapacitor, we also investigated charge-discharge properties at 5, 20, 50, 100, 200, 500, and 1 000 mA, and the maximum voltage of 2.5 V. Fig.5 shows the charge and discharge cycling curves for the PC-based wound supercapacitor at the current of 200 mA and 25 ℃. Capacitance and RES of the supercapacitor at 200 mA were calculated from Fig.5 according to Eqns.(1) and (2). Constant values of the capacitance and resistance were obtained after 10 cycles. Capacitance and RES at different currents were then calculated in the same way. The shape of the cycling curves obtained for the AN-based wound supercapacitor is similar to this one. It can be seen from Fig.5 that that the voltage changes linearly with the change of charge-discharge time on the condition of keeping the current stable, which indicates that the double-layer reaction on the interface between activated carbon and electrolyte is the main reaction of the separation of electrolyte ion. The mirror-like curve indicates the typical capacitance behavior of supercapacitor, which is in accordance with the result observed from the CV curve before.
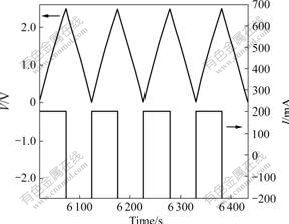
Fig.5 Charge-discharge cycling curves for PC-based supercapacitor at current of 200 mA
To investigate the influence of electrolyte on electrochemical behavior of the carbon based wound supercapacitors, we measured the charge-discharge curves of the two kinds of supercapacitors based on different electrolytes. Fig.6 shows the charge-discharge curves for AN-based and PC-based supercapacitors at the current of 100 mA and 25 ℃. It can be seen that the charge-discharge curves are linear. The charge time and discharge time of AN-based supercapacitor are longer than those of the PC-based supercapacitor.
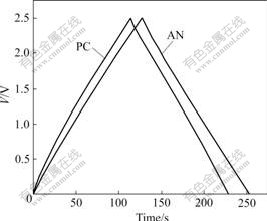
Fig.6 Charge-discharge curves for AN-based and PC-based supercapacitors at current of 100 mA
The capacitance and RES of the AN-based supercapacitor and PC-based supercapacitor at different currents and 25 ℃are shown in Fig.7. When the charge-discharge current is 5 mA, the discharge capacitances of the AN-based supercapacitor and PC-based supercapacitor are 5.2 and 4.9 F, respectively. From Fig.7, it can be seen that the capacitance of the supercapacitor decreases with the increase of charge-discharge current. And, the charge-discharge current is expanded to 1 000 mA, the capacitances of the AN-based supercapacitor and PC-based supercapacitor are 4.84 and 4.08 F, respectively, exhibiting good high- power characteristics.
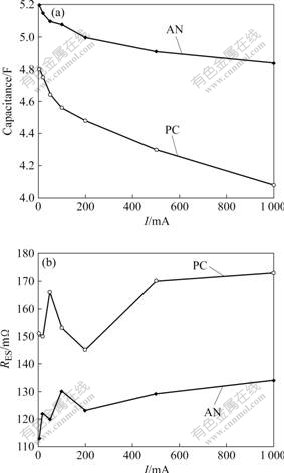
Fig.7 Capacitance (a) and resistance (b) of AN-based supercapacitor and PC-based supercapacitor at different currents
Meanwhile, compared with the AN-based supercapacitor and PC-based supercapacitor, it can be seen that capacitance of the former is always higher than that of the latter, which can be explained by the difference in resistance and dielectric constant existing between two solvents. Moreover, the former shows better rate discharge property because of higher conductivity electrolyte used.
RES of the AN-based supercapacitor and PC-based supercapacitor ranges from 113 to 134 mΩ and from 145 to 175 mΩ, respectively. The resistance of the latter is larger than that of the former. The increase of the RES of the PC-based supercapacitor comes from the higher resistance of PC electrolyte as compared with AN electrolyte.
3.4 Influence of temperature on capacitance behavior of supercapacitor
Temperature aspects of supercapacitor play an important role for optimization of supercapacitor performance with respect to a specific application. As demonstrated above, charge-discharge curve is a well-suited technique to characterize supercapacitor performance. We, therefore, measured the charge- discharge curves of the AN-based supercapacitor and PC-based supercapacitor at various temperatures and the current of 100 mA in order to determine the dependence of characteristics parameters on temperature. The capacitance and RES of the AN-based supercapacitor and PC-based supercapacitor between 60 and -40 ℃ are shown in Fig.8. It can be seen that the activated carbon wound supercapacitors have good capacitive behavior in the temperature range from 60 to -40 ℃. The dependence of the capacitance of the AN-based supercapcitor is rather weak. However, it can be seen that a significant decrease of capacitance is observed in the PC-based supercapacitor, which is almost absent for the AN-based supercapacitor exhibiting minor temperature effect. In addition, a significant increase of the resistance with the decrease of temperature is observed for PC-based supercapacitor, which the RES increases from 135 to 741 mΩ. Such an obvious increased resistance will have significant impact on the power capability of the supercapacitor at low temperature. However, the resistance of AN-based supercapacitor slightly increases in the temperature range from 60 ℃ to -40 ℃.
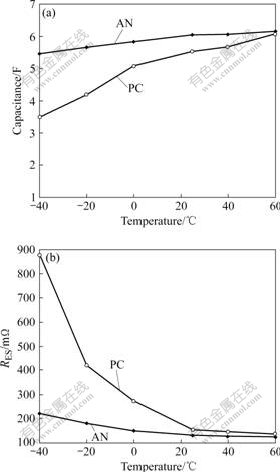
Fig.8 Capacitance (a) and resistance (b) of AN-based supercapacitor and PC-based supercapacitor at different temperatures
4 Conclusions
(1) Activated carbon wound supercapacitors are built based on TEABF4/PC and TEABF4/AN electrolytes.
(2) The supercapacitors of the two electrolytes exhibit good capacitive behavior in the voltage range of 0-2.5 V.
(3) Capacitances of the AN-based supercapacitor and PC-based supercapacitor range from 4.84 to 5.2 F and from 4.08 to 4.9 F, respectively, at the discharge current between 5 and 1 000 mA and 25 ℃. Moreover, RES of the AN-based supercapacitor and PC-based supercapacitor ranges from 113 to 134 mΩ and from 145 to 175 mΩ, respectively.
(4) The supercapacitors can operate in the temperature range of 60 to -40 ℃. Compared with PC-based supercapacitors, the AN-based supercapacitors have higher capacitance and lower RES and minor temperature effect, exhibiting better power characteristics.
References
[1] K?TZ R, CARLEN M. Principles and applications of electrochemical capacitors [J]. Electrochimica Acta, 2000, 45(15/16): 2483-2498.
[2] HUGGINS R A. Supercapacitors and electrochemical pulse sources [J]. Solid State Ionics, 2000, 134(1/2): 179-195.
[3] MARIE-FRANCOISE J N, GUALOUS H, OUTBIB R, BERTHON A. 42V power net with supercapacitor and battery for automotive applications [J]. Journal of Power Sources, 2005, 143(1/2): 275-283.
[4] BURKE A. R&D considerations for the performance and application of electrochemical capacitors [J]. Electrochimica Acta, 2007, 53(3): 1083-1091.
[5] SARANGAPANI S, TILAK B V, CHENC P. Materials for electrochemical capacitors [J]. Journal of the Electrochemical Society, 1996, 143(11): 3791-3799.
[6] MACHEFAUX E, BROUSSE T, B?LANGER D, GUYOMARD D. Supercapacitor behavior of new substituted manganese dioxides [J]. Journal of Power Sources, 2007, 165(2): 651-655.
[7] KIM J H, SHARMA A K, LEE Y S. Synthesis of polypyrrole and carbon nano-fiber composite for the electrode of electrochemical capacitors [J]. Materials Letters, 2006, 60(13/14): 1697-1701.
[8] QU D Y. Studies of the activated carbons used in double-layer supercapacitors [J]. Journal of Power Sources, 2002, 109(2): 403-411.
[9] BARBIERI O, HAHN M, HERZOG A, K?TZ, R. Capacitance limits of high surface area activated carbons for double layer capacitors [J]. Carbon, 2005, 43(6): 1303-1310.
[10] PANDOLFO A G, HOLLENKAMP A F. Carbon properties and their role in supercapacitors [J]. Journal of Power Sources, 2006, 157(1): 11-27.
[11] CENTENO T A, HAHN M, FERNANDEZ, K?TZ R, STOECKLI F. Correlation between capacitances of porous carbons in acidic and aprotic EDLC electrolytes [J]. Electrochemistry Communications, 2007, 9(6): 1242-1246.
[12] LOTA G, CENTENO T A, FRACKOWIAK E, STOECKLI F. Improvement of the structural and chemical properties of a commercial activated carbon for its application in electrochemical capacitors [J]. Electrochimica Acta, 2008, 53(5): 2210-2216.
[13] UE M, TAKEDA M, TAKEHARA M, MORI S. Electrochemical properties of quaternary ammonium salts for electrochemical capacitors [J]. Journal of the Electrochemical Society, 1997, 144(8): 2684-2688.
[14] CONWAY B E. Electrochemical supercapacitors [M]. New York: Kluwer Academic/Plenum Publisher, 1999.
[15] HU C C, WANG C C. Effect of electrolytes and electrochemical pretreatments on the capacitive characteristics of activated carbon fabrics for supercapacitors [J]. Journal of Power Sources, 2004, 125(2): 299-308.
[16] PELL W G, CONWAY B E. Analysis of power limitations at porous supercapacitor electrodes under cyclic voltammetry modulation and DC charge [J]. Journal of Power Sources, 2001, 96(1): 57-67.
Foundation item: Project(20803095) supported by the National Natural Science Foundation of China; Project(2008AA03Z207) supported by the National High Technology Research and Development Program of China
Received date: 2008-08-05; Accepted date: 2008-10-14
Corresponding author: ZHANG Zhi-an, PhD; Tel: +86-731-8876454; E-mail: zhianzhang@sina.com
(Edited by YANG You-ping)