
CFD simulation on performance of new type umbrella plate scrubber
LI Shan-hong(李珊红), LI Cai-ting(李彩亭), ZENG Guang-ming(曾光明),
LI Si-min(李思民), WANG Fei(王 飞), WANG Da-yong(王大勇)
College of Environmental Science and Engineering, Hunan University, Changsha 410082, China
Received 25 April 2007; accepted 26 June 2007
Abstract: A new type of umbrella plate scrubber was developed to address the pollution due to the dust, dioxide sulfur and other harmful gases, which were emitted from coal-burning boilers. The performance of the new device was studied through computational fluid dynamics(CFD) simulation and experiment methods. Initial work included experimental measurement of inlet-velocity, and gas phase simulation using Reynolds stress model(RSM). After gas phase was converged, particles were injected from the inlet of the new device. Discrete phase model(DPM) was used for particle trajectories determination. The pressure drop and the collection efficiency of the new device were predicted through simulation. The simulation results show that the pressure drop of the new devices is 230-250 Pa and the efficiency is 84%-86%, with the inlet velocity equal to 10.6 m/s and the dust concentration ranging from 2 to 22 g/m3. The CFD simulation results of the new device show good agreement with experimental data. The relative error of the pressure drop and the efficiency is approximately 4% and 10% respectively. The results obtained both from the numerical simulation and from the experiment demonstrate that CFD simulation is an effective method for this type of study.
Key words: umbrella plates; scrubber; CFD; discrete phase model(DPM); performance
1 Introduction
Over the recent years, as computing costs have decreased, computational fluid dynamics(CFD) has found widespread applications, particularly in the simulation of complicated flows[1]. These include applications in the aerospace, automobile, and combustion[2-4]. In addition, CFD has led to shift of scientific study and engineering design through experiments to numerical simulation[5]. For instance, in the environmental engineering, CFD was applied to evaluate the indoor air quality and to simulate the pollution dispersion, and the diffusion of automobile exhaust into canyons or tunnels[6-8]. Furthermore, abundant literature reported the simulation of performance evaluation and optimal design of secondary precipitator, cyclone, hydro-cyclone, and fluidization bed[9-12]. Recently, a few researchers reported the CFD simulation on venturi and sieve plates scrubbers[13-14]. However, as far as the study on the new type umbrella plate scrubber is concerned, few researchers reported its performance with complex structure through CFD simulation. Therefore, the objective of this work is to perform a two phase flow CFD simulation and experimental study on the scrubber with new type umbrella plates. The study of the liquid drop scrubbing inside the scrubber will be reported in the future.
2 Experimental
2.1 Apparatus
Experimental apparatus: micro-computer digital manometer (Model SYT2000, Shanghai Guigu Instruments Co., Ltd); Pitot tube (Model Y25-150); thermometer; aneroid barometer (Changchun Metorological Aptitude Apparatus Factory); rubber hoses; mettler analytical balance (Model AE200, Mettler Toledo Shanghai Co., Ltd); laser particle analyzer (Model JL-1155, Chengdou Jinxing Powder Analyse Equipment Co., Ltd); micro-computer smoke dust parallel sample instrument (Model TH-880IV, Wuhan Tianhong Aptitude Apparatus Factory).
2.2 Experimental set-up
The schematic diagram of experimental set-up is shown in Fig.1. Dust gas enters the scrubber from the inlet tube, passes through the new type umbrella plates (reverse lay out), then is dewatered through demister, and the purified gas is released to the atmosphere through the exhaust fan. Absorbed liquid is extracted from the supernatant fluid in the circulating water tank and sprays through the nozzle. The scrubbed liquid returns to the circulating water tanker. The waste is cleared up periodically.
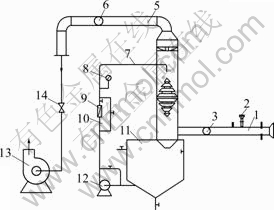
Fig.1 Schematic diagram of experimental set-up: 1 Inlet (gas); 2 Feeder; 3 Inlet test cross section; 4 Scrubber; 5 Outlet(gas); 6 Outlet test cross section; 7 Inlet (water); 8 Manometer; 9 Flow meter; 10 Valve; 11 Circulating water tank; 12 Pump; 13 Exhaust fan; 14 Volume damper
Therein, the upper and lower umbrella plates, both are placed reversely. Dust gas up-flows along the lower umbrella plates and front impacts the absorbing liquid which down-flows from the upper umbrella plates. The absorbing liquid forms liquid membrane at the umbrella plates, and down-flows through layer upon layer umbrella plates. The surfaces increase gradually and obtain the maximum at the lowest umbrella plate. And then the liquid down-flows along the opposite place umbrella plates. Dust gas is scrubbed through further contact with the liquid membrane. The absorbing liquid sheds from the orifice at the bottom layer plate. Dust gas is purified through inertial collision, intercepts of the umbrella plates and wetting, absorption of the absorbing liquid, and the adsorption among the particles.
The pressure drop and efficiency of the new device were measured at the inlet-velocity from 10.6 to 15.5 m/s and the dust concentration from 2 to 22 g/m3. The experimental data would provide parameters for the simulation and validate the simulated results.
3 Simulation
3.1 Governing equations
For diluted dust gas, the incompressible N-S equations supplemented by a suitable turbulence model, such as k-ε turbulence model and Reynolds stress model (RSM), are appropriate for modeling the flow in the new type umbrella plate scrubber. The following equations describe the steady-state conservations of mass and momentum.
Continuity equation:
(1)
Momentum equation:

(2)
where ui is the velocity; xi is the position; i, j=1, 2, 3; t is the time; μ is the viscosity; ρ is the density; p is the pressure; τij is Reynolds stress tensor.
3.2 Reynolds stress model(RSM)
As well known to all, the k-ε model and RSM belong to a group of turbulence models known as Reynolds Average Navier-Stokes(RANS) that provides time-averaged flow variables. These variables can be time independent for cases of unsteady state flows. And the k-ε model is widely applied in practical engineering area. Nevertheless, JONES and LAUNDER pointed out that k-ε model failed to provide reliable results of swirling flows and highly strained angular velocities of rotating flows[15]. RSM abandoned the isotropic eddy-viscosity hypothesis, considered the anisotropic of the flow, and could describe the swirling flow[16]. Since the authors are dealing here with a complex flow, RSM are studied. The transport equations for the Reynolds stresses can be expressed as
(3)
where Dij is the diffusion term; Pij is the production term; Φij is the pressure-strain term; εij is the dissipation rate;
is the mean velocity; xk is the position; i, j, k=1, 2, 3; ρ is the constant mass density. Here,
is the ith fluctuation velocity component, ui is the instantaneous velocity.
3.3 Modeling of particle-phase flow by discrete phase modeling(DPM)
The particles are injected as a discrete phase that can be simulated in a Lagrangian frame of reference by defining the initial position, velocity and size of individual particles. The spherical particles disperse in the gas flow. The initial conditions, along with the inputs defining the physical properties of the discrete phase, are used to initiate trajectory calculations. The trajectory calculations are based on the force balance on the particle, using the local continuous phase conditions as the particle moves along with the gas flow. The formulation assumes that the particle phase is sufficiently dilute, the particle-particle interactions and the effects of the particles on the gas flow are negligible. It can be described as the following equation:
(4)
Other contributions to the force on the particle (buoyancy, virtual mass and Basset term) are negligible because of the assumption of the small fluid-to-particle density ratio. The drag factor f is defined as
(5)
where ug is the gas phase velocity, up is the particle velocity, dp is the particle diameter, μg is the air viscosity, ρp is the particle density, g is the gravity acceleration, CD is the drag coefficient.
3.4 Computation
3.4.1 Methods and zone of calculation
Firstly, the flow field of the continuous gas phase is solved through RSM. After the gas flow converges, DPM is selected to describe the movement of the particles. The calculation zone of the new type umbrella plate scrubber is from the inlet test cross section to outlet test cross section, and the liquid interface. The diameter of the scrubber is 200 mm. The umbrella plates are made up of plates with tilted angle of 45?.
3.4.2 Boundary conditions
An inlet velocity boundary condition and an outlet pressure boundary condition (outlet gauge pressure equals zero) are applied. The fly ash is set as solid phase. The particles distribution is shown in Fig.2. Column walls are considered as no-slid. The closed wall surfaces are treated as standard wall function. The hypothesis assumes that the particles are collected when they collide with the wall of the new type umbrella plate scrubber. The particles leaving the outlet tube are regarded as escape. Particles collision with the umbrella plates and the demister, are considered as inelastic collision.
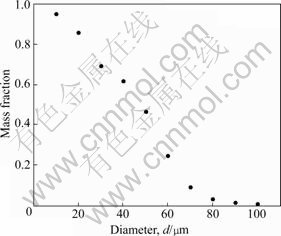
Fig.2 Particle diameter distribution of fly ash
3.4.3 Solution of equations
The flow of dust gas through the new type umbrella plate scrubber is simulated using a CFD software package (Fluent). The domain is discretized into arbitrary unstructured polyhedron. The discrete form of the governing equations for each cell is obtained such that the conservation principles are obeyed on each polyhedron. In order to solve the above Eqns.(1)-(5), segregated iterative procedure is adopted for the current application. The first order upwind is used for the momentum. The SIMPLE algorithm is used to solve the coupled continuity and momentum equations[16].
3.5 Simulation results and analysis
1) With uin=12 m/s, Fig.3 shows the velocity magnitude distribution along the Z axial. The velocity magnitude fluctuated range is from 1 to 10 m/s near the umbrella plates. At the entrance to the scrubber, there is a big velocity climax. So is it at the outlet tube. As the liquid phase is considered, the empty tower velocity is larger. It is more benefit for the dust particles purification because the soakage increases.
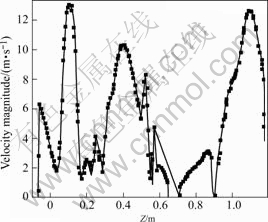
Fig.3 Velocity magnitude (uin=12 m/s)
2) With uin=12 m/s, Cin=5.822 3 g/s and Y=0 section, the distribution of the Z-velocity, pressure, and DPM concentration in the new type umbrella plates scrubber are shown in Figs.4(a), (b) and (c).
As shown in Fig.4, with uin=12 m/s, Cin=5.822 3 g/s and Y=0 section, the simulation of the Z-velocity, pressure, and DPM concentration distribution in the new type umbrella plates scrubber reflects the motion state of gas flow and particles. The dust gas enters the scrubber and continuously moves forward because of inertia. After collision with the wall, the gas changes moving direction and moves up. The larger particles deposit because of the gravity force while most of the finer particles follow the gas flow because of their lack of inertia. Some finer particles bypass the new scrubber, and agglomerate into larger particles, which then deposit because of the interception and collision with the umbrella plates. In addition, there exist a lot of vortexes near the umbrella plates, which would potentially help the gas-solid blending. In the scrubber, the number of the particles drops significantly, which indicates high collection efficiency of the designed equipment. Meanwhile, the pressure drop is about 200 Pa across the umbrella plates. The authors believe that the gas cross-flow around the umbrella plates causes the increase of the friction.
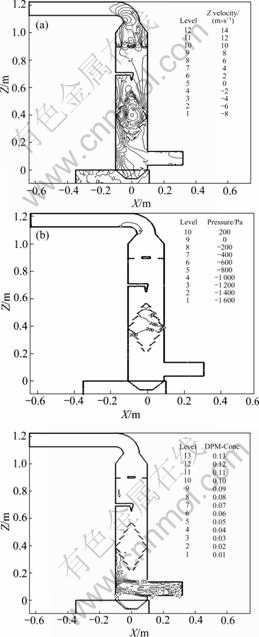
Fig.4 Z-velocity(a), pressure(b), and DPM concentration (c) distribution of new type umbrella plate scrubber (uin=12 m/s, Cin=5.822 3 g/s and Y=0 section)
3) With Cin=5.822 3 g/s, the relationship between the pressure drop(Δp), efficiency(η) and inlet-velocity is shown in Fig.5.
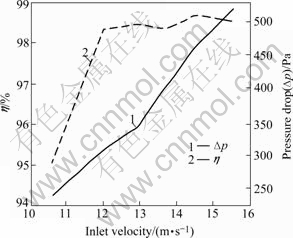
Fig.5 Relationship between pressure drop, efficiency and inlet-velocity (Cin=5.822 3 g/s)
From Fig.5, it can be seen that Δp and η increase with the inlet-velocity increasing. When uin≥12 m/s, Δp increases quickly, while η keeps almost constant. It can be explained as follows. The friction between the phases increases with the gas flow velocity, which, in turn, causes the resistance to increase significantly. On the other side, the increase of the gas flow velocity leads to more collision of particles to the scrubber plates. This contributes to the fact that more particles deposit and get separated. Therefore, the η increases. Hence, the best operating parameter, gas inlet velocity, is about 12 m/s (Note, the velocity of the empty scrubber equals 3 m/s).
4 Comparison
The RSM and DPM are adopted to simulate and study the new type umbrella plate scrubber. Fig.6 shows the relationship of Δp and η to the dust concentration with the dust concentration Cin in the range 2-22 g/m3 and inlet velocity uin equal to 10.6 m/s. The simulated Δp and η of the new type umbrella plate scrubber is 230-250 Pa and 84%-86%, respectively. In comparison with the experiment results, the relative error is below 4% and 10%, respectively. However, when Cin≤6.532 0 g/m3, there exists obvious difference between the simulated η and the experimental observation. This is because the dilute particles are used in the experiment, and hence the collision opportunity of the particles reduces. In general, the simulation results agree well with the experimental data.
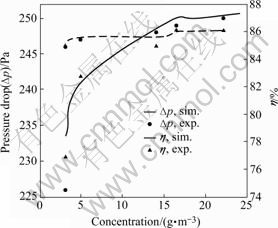
Fig.6 Comparison of efficiency and pressure drop between experiment and simulation (gas-solid two phase, uin=10.6 m/s)
5 Conclusions
1) The umbrella plates in the new type umbrella plate scrubber increase the contact interface, the turbulent intensity, and the retention time, which, in turn, contribute to the particles separation.
2) The CFD simulation results demonstrate the distribution of the velocity, pressure, and concentration of the new type umbrella plate scrubber. Based on the results, optimal design of the equipment set-up can be realized.
3) With uin=10.6 m/s and Cin=2-22 g/m, the CFD simulation results agree very well with experimental data. The relative difference of Δp and η between simulation results and experimental data are below 4% and 10% respectively. This demonstrates again that CFD simulation is an effective tool to evaluate and predict the performance of the new device.
In general, the CFD simulation can improve efficiency, reduce cost, and shorten experimental periods. In addition, there is no disturbance of the experiment apparatus. Furthermore, it can deliver detailed data in visualizable format which are not obtainable via experiments. It can also provide insights for optimal equipment design.
References
[1] BERNARDO S, MORI M, PERES A P, DION?SIO R P. 3-D computational fluid dynamics for gas and gas-particle flows in a cyclone with different inlet section angles [J]. Powder Technology, 2006,162(3): 190-200.
[2] LEE C W, PALMA, P C SIMMONS K, PICKERING S J. Comparison of computational fluid dynamics and particle image velocimetry data for the airflow in an aeroengine bearing chamber [J]. Journal of Engineering for Gas Turbines and Powder, 2005, 127(4): 697-703.
[3] LYSENKO D A, SOLOMATNIKOV A A. Numerical modeling of turbulent heat exchange in the combustion chambers of gas-turbine plants with the use of the FLUENT package [J]. Journal of Engineering Physics and Thermophysics, 2003, 76(4): 888-891.
[4] H?SEYIN Y, NESRIN K, BILGE A, GAMZE B. Numerical study on local entropy generation in a burner fueled with various fuels [J]. Heat and Mass Transfer, 2005, 41(6): 519-534.
[5] PANT K, CROW C T, IRVING P. On the design of miniature cyclones for the collection of bioaerosols [J]. Powder Technology, 2002,125(2/3): 260-265.
[6] POSNER J D, BUCHANAN C R, DUNN-RANKIN D. Measure- ment and prediction of indoor air flow in a model room [J]. Energy and Buildings, 2003, 35(5): 515-526.
[7] WANG X, MCNAMARA K F. Evaluation of CFD simulation using RANS turbulence models for building effects on pollutant dispersion [J]. Environmental Fluid Mechanics, 2006, 6(2): 181-202.
[8] ISAO K, KIYOSHI U, YUKIO Y, YOSHIKAWA Y, MORIKAWA T. A wind-tunnel study on exhaust gas dispersion from road vehicles (I): Velocity and concentration fields behind single vehicles [J]. Journal of Wind Engineering and Industrial Aerodynamics, 2006, 94(9): 639-658.
[9] KARAMA A B, ONYEJEKWE O O, BROUCKAERT C J, BUCKLEY C A. The use of computational fluid dynamics(CFD) technique for evaluating the efficiency of an activated sludge reactor [J]. Water Science and Technology, 1999, 39(10/11): 329-332.
[10] JIAO J Y, ZHENG Y, SUN G G, WANG J. Study of the separation efficiency and the flow field of dynamic cyclone [J]. Separation and Purification Technology, 2006, 49(2): 157-166.
[11] NARASIMHA M, SRIPRIYA R, BANERJEE P K. CFD modelling of hydrocyclone-prediction of cut size [J]. International Journal of Mineral Processing, 2005, 75(1/2): 53-68.
[12] LU H L, ZHAO Y H, DING J M, DIMITRI G, LI W. Investigation of mixing/segregation of mixture particles in gas-solid fluidized beds [J]. Chemical Engineering Science, 2007, 62(1/2): 301-317.
[13] XAVIER G, SARR? M, JAVIER LAFUENTE F. Fluid flow and pumping efficiency in an ejector-venturi scrubber [J]. Chemical and Engineering Processing, 2004, 43(2): 127-136.
[14] MOHEBBI A, TAHERI M, FATHIKALJAHI J, TALAIE M R. Simulation of an orifice scrubber performance based on eulerian/lagrangian method [J]. Journal of Hazardous Materials, 2003, 100(1/3): 13-25.
[15] JONES W P, LAUNDER B E. The prediction of laminarization with a two-equation model of turbulence [J]. Internation of Journal Heat and Mass Transfer, 1972, 15(2): 301-314.
[16] VERSTEEG H K, MALALASEKERA W. An introduction to computational fluid dynamics: The finite volume method [M]. London: British Library Cataloguing in Publication Data, 1995.
Foundation item: Project(03JJY2002) supported by the Key Project of Natural Science Foundation of Hunan Province, China; Project(NCET-04-0769) supported by the Program for New Century Excellent Talents in University, China; Project(105126) supported by the Key Project of Ministry of Education of China
Corresponding author: LI Cai-ting; Tel: +86-731-8649216; E-mail: ctli@hnu.cn
(Edited by YUAN Sai-qian)