Trans. Nonferrous Met. Soc. China 24(2014) 954-960
Preparation of semi-solid 7075 aluminum alloy slurry by serpentine pouring channel
Wen-zhi ZHU, Wei-min MAO, Qin TU
School of Materials Science and Engineering, University of Science and Technology Beijing, Beijing 100083, China
Received 8 June 2013; accepted 9 August 2013
Abstract: The semi-solid slurry of 7075 aluminum alloy was prepared by a serpentine pouring channel (SCP). Influences of pouring temperature and the number of turns on the microstructure of semi-solid 7075 alloy slurry were investigated. The results demonstrated that the semi-solid 7075 aluminum alloy slurry with satisfied quality could be generated by a serpentine pouring channel when the pouring temperature was in the range of 680-700 °C. At a given pouring temperature, the equivalent size of the primary α(Al) grains decreased and the shape factor increased with the increase of the number of turns. During the slurry preparation of semi-solid 7075 aluminum alloy, the flow direction of alloy melt changed many times when it flowed in a curved and closed serpentine channel. With the effect of “stirring” in it , the primary nuclei gradually evolved into spherical and near-spherical grains.
Key words: 7075 aluminum alloy; semi-solid slurry; serpentine channel; primary α(Al)
1 Introduction
Semi-solid metal (SSM) processing was developed by FLEMINGS et al [1] in the 1970s and has gained increasing interest among researchers during the past two decades. The most important characteristic of semi-solid forming technology is a kind of semi-solid structure with the solid phase presenting in a near spherical form. In order to meet this demand, a wide spectrum of routes and processes have been developed to prepare semisolid slurry. The conventional processes are mechanical stirring and electromagnetic stirring [2,3], however, the conventional processes require specialized equipments so the preparation processes are complex and the electrical energy is required more. Recently, a controlled nucleation method has been reported, which is simple, practical and less expensive because of no application of stirring. According to this idea, several techniques are developed, such as the new rheocasting (NRC) [4-7], the vertical pipe [8,9], rotating duct [10], damper cooling tube method [11,12], wavelike sloping plate [13]. These techniques have similarities, that is, pouring the alloy melt through a plate or tube without stirring. Therefore, they greatly reduce energy consumption and save the cost of production.
Based on the controlled nucleation method, the serpentine channel process was invented. The serpentine channel was made of graphite and it consisted of two symmetrical blocks locked together by screw bolts and nuts. During the period of preparing semisolid slurry, the flow direction of alloy melt changed many times when it flowed in a curved and closed serpentine channel. With the effect of “stirring” in the serpentine curve, the primary nuclei gradually evolved into spherical and near-spherical grains. In this work, the integral microstructure of semi-solid 7075 aluminum alloy slurry by serpentine channel process was studied, and the influences of pouring temperature and the curve numbers on the microstructure of semi-solid 7075 alloy slurry were investigated.
2 Experimental
2.1 Materials and apparatus
The experimental material is 7075 aluminum alloy. The chemical compositions of the alloy are listed in Table 1. The liquidus and solidus temperatures of this alloy are 640 and 477 °C, respectively, tested by differential scanning calorimetric (DSC) method. The temperature range of semi-solid is 163 °C.
The schematic of SCP apparatus and the slurry preparation process is shown in Fig. 1 [14]. It consists of melting crucible, serpentine channel, collective crucible and K-type thermocouple. The melting apparatus is a crucible resistance furnace. The serpentine channel is made of graphite and it consists of two symmetrical blocks locked together by screw bolts and nuts. The collective crucible, with an average diameter of 127 mm, is made of stainless steel. A Ni-Cr/Ni-Si thermocouple is used to measure the temperatures of the melt, the inner wall of serpentine channel and the slurry. The temperature displayed was accurate to ±1 °C.
Table 1 Chemical compositions of 7075 aluminum alloy (mass fraction, %)

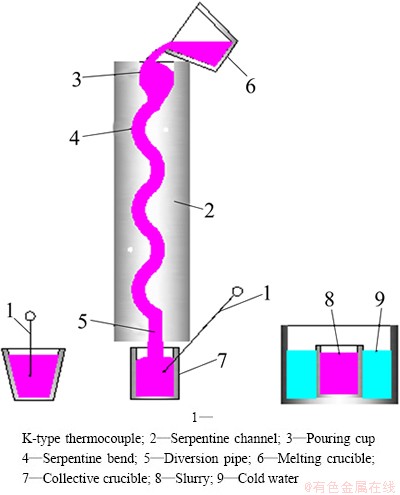
Fig. 1 Schematic diagram of preparing semi-solid 7075 aluminum alloy slurry by serpentine channel pouring
2.2 Methods
The alloy was melted in a crucible resistance furnace at 760-770 °C, and then incubated for 10 min. The melt was cooled to the chosen pouring temperature and cast into a stainless steel crucible via serpentine channel, at the same time, the outlet temperatures of the slurry were measured. Then the slurry was quenched in cold water rapidly.
The microstructures of samples polished and etched in Keller’s reagent (the solution of 1% HF, 1.5% HCL, 2.5% HNO3 and 95% distilled water) for 25 s, were analyzed by optical microscopy. The diameter of primary α(Al) grains was calculated as: D=2(A/π)1/2 (where A is the area of a grain), and the shape factor of primary α(Al) grains was calculated as: F=4πA/P2 (where A and P are the area and perimeter of a grain, respectively).
3 Results
3.1 Microstructure of semi-solid slurry of 7075 aluminum alloy by SCP
Figure 2 shows the microstructure of 7075 aluminum alloy billet prepared by conventional casting with the collective crucible at 700 °C and quenched at 630 °C. The white phase is primary α(Al) and the black matrix is the eutectic phase. Typical dendrites of conventional solidification with length of more than 200 μm are observed.
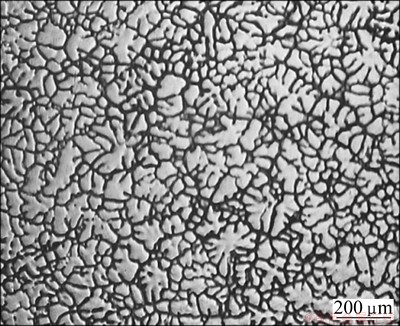
Fig. 2 Typical dendrites microstructure of 7075 alloy prepared by conventional solidification
Figure 3 shows the microstructures of the semisolid slurry prepared by SCP with different processing parameters. It is clear that the solidification microstructures consist of non-dendrites primary α(Al) grains which disperse uniformly in the matrix and eutectics at intergranular boundaries. The grain diameter and the shape factor of primary α(Al) grains are listed in Table 2. Figures 3(a)-(c) show the microstructures of 7075 aluminum alloy semi-solid slurry poured at 720, 700, 680 °C by 4 turns serpentine channel, respectively. The grain diameters and the shape factors of primary α(Al) grains are 73, 71, 70 μm and 0.58, 0.60, 0.76, respectively. Figure 3(d)-(f) show the microstructures of 7075 aluminum alloy semi-solid slurry poured at 720, 700, 680 °C by 5 turns serpentine channel, respectively. The grain diameters and the shape factors of primary α(Al) grains are 72, 70, 68 μm and 0.61, 0.66, 0.77, respectively. Figures 3(9)-3(i) show the microstructures of 7075 aluminum alloy semi-solid slurry poured at 720, 700, 680 °C by 6 turns serpentine channel, respectively. The grain diameters and the shape factors of primary α(Al) grains are 70, 68, 64 μm and 0.72, 0.74, 0.78, respectively. It is concluded that the size and morphology of primary α(Al) grains are influenced significantly by pouring temperature and the number of turns.
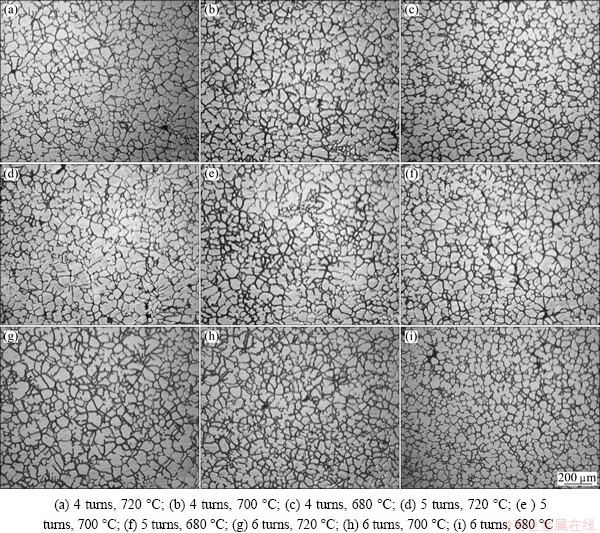
Fig. 3 Microstructures of semi-solid 7075 aluminum alloy slurries prepared by serpentine channel pouring process at different number of turns and pouring temperatures
Table 2 Processing parameters and characteristic size of semi-solid slurries
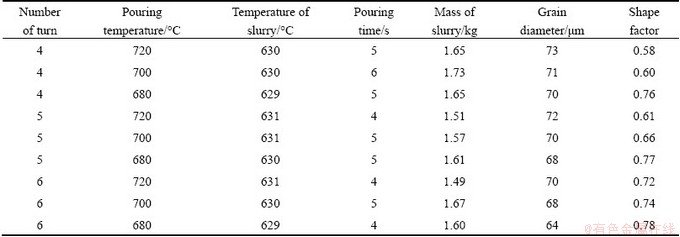
3.2 Influence of pouring temperature on slurry microstructure
As shown in Figs. 3(a)-(c), the primary α(Al) grains being more spherical and with non-dendrites emerged under the condition of decreasing pouring temperature using the same serpentine channel. In Fig. 3(a), as the slurry was prepared at 720 °C, the morphology of primary α(Al) grains is irregular and some ones are still stuck together. A similar microstructure can be seen and there are still some contacted grains, as shown in Fig. 3(b). When a lower pouring temperature is adopted, such as 680 °C, most contacted grains separate compared with the as-cast structure shown in Figs. 3(a) and (b) and the primary α(Al) grains become more spherical. The schematic diagram of hanging slurry in serpentine channel is shown in Fig. 4. It is clear that the hanging slurry increases with decreasing pouring temperature. The variation of grain diameters and the shape factors with different pouring temperatures are shown in Fig. 5. The results indicate that the grain diameter increases with increasing pouring temperature and the shape factor decreases with increasing pouring temperature. It can be concluded from this group of experiments that the better spheroidization of dendrites α(Al) can be obtained with a relatively lower pouring temperature by SCP, however, the pouring temperature should not be too low because the hanging slurry in serpentine channel may plug up the channel. So the semi-solid slurry is prepared with satisfied quality when the pouring temperature range of this group of experiments is between 680 and 700 °C.
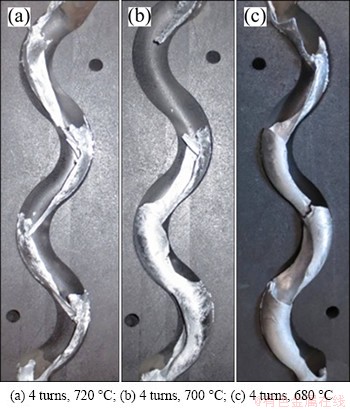
Fig. 4 Schematic diagram of hanging slurry in serpentine channel at different number of turns and pourning temperatures
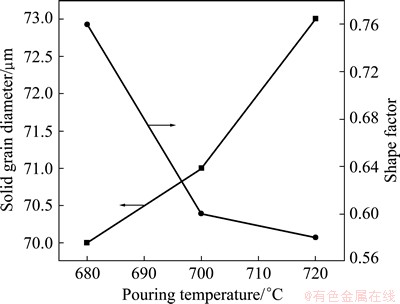
Fig. 5 Pouring temperature versus grain diameter and shape factor
3.3 Influence of number of turns on slurry microstructure
As shown in Figs. 3(c), (f) and (i), the primary α(Al) grains are smaller and more spherical by 6 turns serpentine channel compared with the other primary α(Al) grains at the same pouring temperature. In Fig. 3(c), as the slurry is prepared by 4 turns serpentine channel, the shape of primary α(Al) grains are polygon. The similar microstructure can be seen but the grain is is smaller, as shown in Fig. 3(b). Compared with the as-cast structure shown in Figs. 3(c) and (f), the primary α(Al) grains in Fig. 3(i) become more spherical and the grain size is much smaller. The schematic diagrams of hanging slurry in serpentine channel are shown in Fig. 6. It is clear that the hanging slurry increases with the number of turns increasing. The variation of grain diameters and the shape factors with different number of turns is shown in Fig. 7. The results indicate that the grain diameter decreases with the increasing number of turns and the shape factor increases with the increasing turn numbers. It can be seen from this group of experiments that the best spheroidization of dendritic α(Al) can be obtained with 6 turns serpentine channel, but the hanging slurry is the most. Considering continuous casting, the best serpentine channel of this group of experiments is 5 turns.
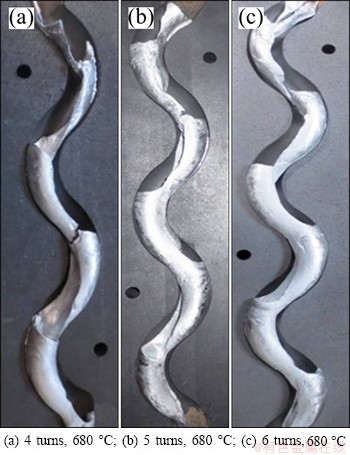
Fig. 6 Schematic diagram of hanging slurry in serpentine channel
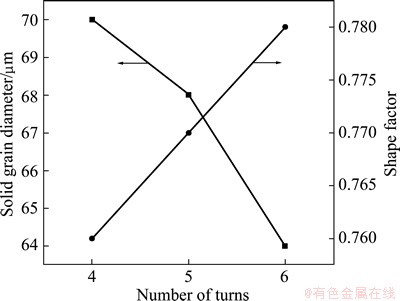
Fig. 7 Number of turns versus grain diameter and shape factor
4 Discussion
Two mechanisms proposed to explain the grain structure formation are dendrite fragmentation [15-18] and spherical microstructures formation directly from the alloy melt [19,20]. According to the experimental analysis, it can be concluded that the reliable mechanism of serpentine channel process is the latter.
The main mechanism of crystal nucleation of the alloy melt on the inner concave surfaces of serpentine channel is heterogeneous nucleation, whose nucleation ratio depends on the critical energy of nucleation and the activation energy of diffusion of the atom in the liquid phase. During the pouring process, the alloy melt flows down along the inner concave surfaces by the action of gravity, whose velocity varied with time and displacements are different in different points, both of which must lead to the occurring of shearing forces. What’s more, the magnitudes and directions of shearing forces vary with the positions of the alloy melt. In a word, all of which can be seen as the action of self-stirring of serpentine channel. And, turbulence caused by the action of self-stirring of the alloy melt prompting more shaping of micro heat fluctuation and phase fluctuation is beneficial for crystal nucleation and indirectly increases the number of the core interface of heterogeneous nucleation. At the same time, energy fluctuation decreases the critical energy of nucleation and therefore increases the nucleation ratio.
For one thing, the alloy melt on the surfaces of serpentine channel has super-cooling temperature, which largely prompts the crystal nucleation of the alloy melt that is tightly stuck to the inner surfaces of the serpentine channel. For another, the nucleation substrate is concave surface that is better for crystal nucleation than other surfaces such as horizontal surfaces or convex surfaces. Besides, the density of alloy melt near the inner concave surfaces and that near the centre zone in the serpentine channel are different, which causes the fast convection amid the alloy melt. And also, the fast convection makes the temperature of the whole melt relatively even and super-cooling, which also can make a large number of crystal nuclei emerge.
What’s more, these crystal nuclei can float down or move into the alloy melt and grow up when they flow down along with the alloy melt, that is to say, not all these crystal nuclei grow up locally, but also in the places where they have been brought, which again prompts the crystal nucleation in extremely large number. Therefore, under the function of alloy melt’s washing down and the self-stirring of serpentine channel, one part of crystal nuclei near the inner surfaces that moves or floats into the rest alloy melt or flows down becomes the free crystal nuclei, and the other part that will stay on the inner surfaces and then grow up becomes the solid shell sticking to the inner surfaces.
The temperature gradient of solid-liquid frontier, the concentration gradient of solutes and the intensity and direction of heat diffusion of the alloy melt in the serpentine channel decide the future microstructures of primary crystal nuclei. During the SCP process, the fast convection amid the alloy melt causes the instaneous mixing of inner heat and different parts of the alloy melt, which makes the component and the temperature field of the melt on the whole relatively even and the temperature and concentration gradient rather decrease. Along with the motion in the convection of melt, the fast self-rotating of these crystal nuclei puts themselves in a relatively even growing circumstance and makes them continuously change their positions when they move down, which weakens the requirements of dendrites emerging by a large scale. Under this condition, the crystal nuclei will grow up evenly in all directions and finally turn into spherical grains or near spherical grains. Figure 8 shows the microstructures of solidified shell.
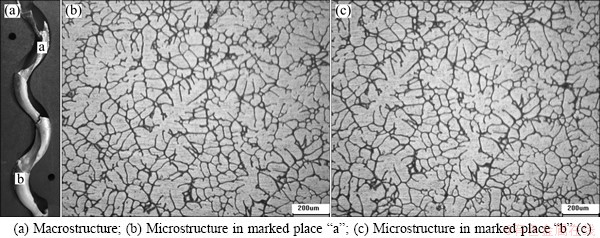
Fig. 8 Microstructures of solidified shell
The rosette grains in Fig. 8(c) are less than those in Fig. 8(b), but the near spherical grains are relatively more, which is a piece of further evidence that the alloy melt self-stirring action in the channel can prevent the primary chilling crystal nuclei from growing towards into dendrites. On the contrary, the crystal nuclei will be inclined to turn into spherical or near spherical grains by the comprehensive function of being washing down and convection.
5 Conclusions
1) The semi-solid 7075 aluminum alloy slurry with satisfied quality can be generated by a serpentine pouring channel and the solid shell inside the channel can be avoided when the pouring temperature is in the range of 680 and 700 °C.
2) With a given pouring temperature, the equivalent size of the primary α(Al) grains decreases and the shape factor increases with the increasing of the number of turns, at the same time, as the solid shell inside the channel increases, the chance to obtain a great deal of semi-solid slurry decreases.
3) During the preparation for the slurry of semi-solid 7075 aluminum alloy, the flow direction of alloy melt changes many times when it flows in a curved and closed serpentine channel. With the effect of “stirring” in the serpentine curve, the primary nuclei gradually evolve into spherical and near-spherical grains.
References
[1] FLEMINGS M C. Behavior of metal alloys in the semi-solid state [J]. Metallurgical and Materials Transaction A, 1991, 22(5): 957-981.
[2] MAO Wei-min. Semisolid metal forming technology [M]. Beijing: China Machine Press, 2004: 6. (in Chinese)
[3] LUO Shou-jing, TIAN Wen-tong, XIE Shui-sheng, MAO Wei-min. Semi-solid processing technology and application [J]. The Chinese Journal of Nonferrous Metals, 2000, 10(6): 765-769. (in Chinese)
[4] LUKASSON M, APELIAN D, DASGUPTA R. Alloy characterization for the new UBE rheocasting process [J]. AFS Transactions, 2002, 110: 271-284.
[5] EASTON M A, KAUFMANN H, FRAGNER W. The effect of chemical grain refinement and low superheat pouring on the structure of NRC castings of aluminum alloy Al-7Si-0.4Mg [J]. Materials Science and Engineering A, 2006, 420(1-2): 135-143.
[6] CARDOSO L E, ATKINSON H V, JONES H. Cooling slopecasting to obtain thixotropic feedstock. I: Observations with a transparent analogue [J]. Journal of Materials Science, 2008, 43(16): 5448-5455.
[7] LEGORETTA E C, ATKINSON H V, JONES H. Cooling slope casting to obtain thixotropic feedstock. II: Observations with A356 alloy [J]. Journal of Materials Science, 2008, 43(16): 5456-5469.
[8] YANG Xiao-rong, MAO Wei-min, PEI Sheng. Preparation of semi-solid A356 alloy feedstock cast through vertical pipe [J]. Materials Science and Technology, 2007, 23(9): 1049-1053.
[9] YANG Xiao-rong, MAO Wei-min, PEI Sheng. Influence of process parameters on microstructure of semisolid A356 alloy slug cast through vertical pipe [J]. Transactions of Nonferrous Metals Society of China, 2008, 18(1): 99-103.
[10] GUO H M, YANG X J, HU B. Rheocasting of aluminum alloy A356 by low superheat pouring with a shear field [J]. Acta Metallurgica Sinica, 2006, 19(5): 328-334.
[11] XIE Shui-sheng, ZHANG Xiao-li, YANG Hao-qiang. Study on numerical simulation and experiment of fabrication magnesium semisolid slurry by damper cooling tube method [J]. AIP Conference Proceedings, 2007, 908(1): 557-562.
[12] ZHANG Xiao-li, XIE Shui-sheng, LI Yan-ju, YANG Hao-qiang, JIN Jun-ze. A356 aluminum alloy semisolid slurry prepared by damper cooling tube process [J]. Rare Metal Material and Engineering, 2007, 36(5): 915-919.
[13] GUAN Ren-guo, LI Jian-ping, CHEN Li-qing, WANG Chao. Mechanism alloy microstructure formation during vibrating wavelike sloping plate process [J]. Transactions of Material Research, 2008, 22(4): 363-368.
[14] CHEN Zheng-zhou, MAO Wei-min, WU Zong-chuang. Semi-solid A356 Al-alloy slurry prepared by serpentine pipe with multiple curves casting [J]. The Chinese Journal of Nonferrous Metals, 2011, 21(1): 95-101. (in Chinese)
[15] CHUTT J E, STJOHN D H, DAHLE A K. Equiaxed solidification of Al-Si alloys [J]. Materials Science and Technology, 1999, 15: 495-500.
[16] DOHERTY R D, LEE H I, FEEST E A. Microstructure of stir-cast metals [J]. Materials Science and Engineering, 1984, 65(1): 181-189.
[17] HELAWELL A. Grain evolution in conventional and rheocasting [C]//Proceedings of the 4th International Conference on Semi-Solid Processing of Alloys and Composites. Sheffield: University of Sheffield Press, 1996: 60-65.
[18] MAO Wei-min, ZHAO Ai-min, CUI Cheng-lin, ZHONG Xue-you. The formation mechanism of non-dendritic primary α(Al) phases in semi-solid AiSi7Mg alloy [J]. Acta Metallugica Sinica, 1999, 35(9): 971-974. (in Chinese)
[19] JI S, FAN Z. Solidification behavior of Sn-15% Pb alloy under a high shear rate and high intensity of turbulence during semisolid processing [J]. Metallurgical and Materials Transactions A, 2002, 33(11): 3511-3520.
[20] LI Tao, HUANG Wei-dong, LIN Xin. Formation of globular structure during semi-solid material processing [J]. The Chinese Journal of Nonferrous Metals, 2000, 10(5): 635-639. (in Chinese).
蛇形通道浇注制备半固态7075铝合金浆料
朱文志,毛卫民,涂 琴
北京科技大学 材料科学与工程学院,北京 100083
摘 要:采用蛇形通道浇注技术制备半固态7075 铝合金浆料,研究浇注温度和弯道数量对半固态7075铝合金浆料微观组织的影响。结果表明:当浇注温度为680~700 °C时,可以制备出质量较好的半固态7075铝合金浆料;在相同浇注温度条件下,随着弯道数量的增加,初生α(Al)的平均晶粒尺寸减小,形状因子提高。在浇注制备半固态7075铝合金浆料过程中,合金熔体在具有一定弧度且封闭的蛇形弯道内流动并多次改变流动方向,具有类似“搅拌”的功能,使得初生晶核逐渐演变为球形或近球形晶粒。
关键词:铝合金7075;半固态浆料;蛇形通道;初生α(Al)
(Edited by Chao WANG)
Foundation item: Project (2011CB606302-1) supported by the National Basic Research Program of China
Corresponding author: Wei-min MAO; Tel: +86-13911069562; E-mail: weiminmao@263.net
DOI: 10.1016/S1003-6326(14)63148-4