
Mechanical alloying and subsequent annealing effects on evolution of microstructure and morphology of TiH2-Ni powders
ZHAO Xiang-yu1, MA Li-qun1, DING Yi1, SHEN Xiao-dong1, 2
1. College of Materials Science and Engineering, Nanjing University of Technology, Nanjing 210009, China;
2. State Key Laboratory of Materials-Oriented Chemical Engineering,
Nanjing University of Technology, Nanjing 210009, China
Received 18 March 2010; accepted 1 July 2010
Abstract: Mechanical alloying and subsequent annealing treatment were carried out to investigate the evolution of the microstructure and morphology of the TiH2-Ni system. The Ni(Ti) solid solution was formed in the initial milling process. When the milling time was 60 h, the alloy with uniform elemental distribution showed an amorphous structure containing a small amount of TiH2 nanocrystalline phase. The annealing treatment at 693 K contributed to a completion of amorphization for the alloy milled for 60 h. For the treatment at 1 073 K, a crystallization reaction for the amorphous phase occurred, leading to the formation of Ti2Ni, TiNi, and TiNi3 compounds by a short time treatment. Moreover, a separation of Ni-rich phases from the matrix and a phase transition among these three compounds occur by a long time treatment.
Key words: Ti-Ni alloy; amorphous alloy; annealing; microstructure
1 Introduction
TiNi alloy is a promising material in the areas of shape memory, corrosion resistance, damping and hydrogen storage[1-4]. Several approaches, such as casting[5], powder metallurgy[6], self-propagating high-temperature synthesis[7] and ion beam deposition[8], have been operated to produce TiNi alloys using elemental powders of Ti and Ni as raw materials. In recent years, a non-equilibrium processing technology, mechanical alloying (MA), has been extensively carried out to synthesize TiNi alloys due to its simplicity, low cost and facility to produce a great amount of materials with metastable phases[9-12]. YE et al[11] reported that amorphous Ti-50%Ni (mole fraction) has was obtained by MA. Similar result has been achieved by GU et al[13]. Ternary Ti-M-Ni disordered alloys (M=Zr, V, Cu, Mn, Fe or B), partially substitution of M for Ti or Ni, could also be prepared by mechanical alloying[4, 14]. MOUSAVI et al[15] found that nanocrystalline TiNi intermetallic could be synthesized by MA without subsequent heat treatment. The studies on crystallization behaviors of TiNi alloys containing metastable phases indicated that three compounds, namely TiNi, Ti2Ni, and TiNi3, could be formed by annealing treatment[11, 13, 15].
In this work, we synthesized a TiH2-Ni alloy by using raw materials of TiH2 and Ni powders. The effects of mechanical alloying (MA) and subsequent annealing treatment on the microstructure and morphology of the TiH2-Ni powders were investigated. The crystallization behavior of the milled powders was also studied.
2 Experimental
2.1 Sample preparation
Powders of TiH2 (50 μm, 99.9%) and Ni (35 μm, 99.9%) were blended together to form a nominal composition of TiH2-50%Ni (mole fraction). They were loaded into a stainless-steel container with stainless-steel balls (10 mm and 20 mm in diameter) under an argon atmosphere. The ball to powder ratio was 20:1. The milling was performed in a planetary mill with a rotation speed of 300 r/min and the milling time was 20, 40 or 60 h. The three milling periods were all carried out continuously without interim sampling. The powders milled for 60 h were subsequently annealed at 693 K for 0.5 h, at 1 073 K for 1 h or at 1 073 K for 8 h under an argon atmosphere.
2.2 Characterizations
X-ray diffraction (XRD) with Cu Kα radiation was carried out in a Thermo ARL X’ TRA diffractometer system to determine the structures of the milled and annealed powders. Transmission electron microscopy (TEM) was also employed for structure observation on the sample after annealing treatment on a JEM 2000 EX unit. Differential thermal analysis (DTA) and thermogravimetry (TG) at a heating rate of 0.33 K/s was performed to present the structural and phase transition during annealing treatment. Scanning electron microscopy (SEM) was used to analyze the morphologies of the samples by a JSM-5900 unit.
3 Results and discussion
3.1 Mechanical alloying
Fig.1 shows the variation of XRD patterns for the TiH2-Ni powders as a function of milling time or heat treatment. It is apparent that the intensities of the diffraction peaks corresponding to the raw materials TiH2 (Cubic, PDF card No. 65—708) and Ni (Cubic, PDF card No. 65—2865) are obviously decreased after 20 h of milling. In addition to decreasing the intensity, continuous ball milling induced considerable broadening of the peaks. This is due to the grain size refinement and the increase in the internal strain induced by MA. The formation of an amorphous phase can be inferred by the peak shape and the background of the pattern for the milled powders at 2θ between 37° and 47°.
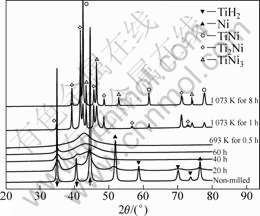
Fig.1 XRD patterns of milled and subsequently annealed TiH2-Ni powders
A gas flowmeter was used to detect the pressure within the milling container at the end of each milling period. It was found that the pressure was almost unchanged after 20 h of milling as compared with the non-milled one, while a drastic increase and a slight increase of the pressure occurred when the milling periods were 40 and 60 h, respectively. This means that hydrogen gas was formed within the container after 40 h of milling. Moreover, the open potentials of the electrodes prepared by the milled powders are -0.989, -0.878 and -0.941 V (vs. Hg/HgO) when the milling periods are 20, 40 and 60 h, respectively. Accordingly, three stages of the milling process can be obtained. The first is the short milling period (in 20 h). In this time range, mechanical milling (MM) leads to a decrease of the stability of titanium hydride and consequently a number of hydrogen atoms adsorb on the powder surface, resulting in the occurrence of hydrogen desorption or hydrogen evolution reaction in the 6 mol/L KOH electrolyte, and thus a large negative open potential of the powders milled for 20 h is presented. In the next time range (from 20 to 40 h), the energy induced by the milling contributes to not only an alloying process (Fig.1), but also the formation of hydrogen gas caused by the decomposition of TiH2 during milling, and thus, the hydrogen concentration on the powder surface is reduced, resulting in the increases of the open potential of the milled powders and the pressure within the container. For further milling (from 40 to 60 h), part of the hydrogen gas is dissociated and subsequently absorbed by the powders, leading to the decreases of the potential of the powders and the pressure. It is interesting that there is no occurrence of hydrogen evolution reaction when the electrode containing the powders milled for 60 h was immersed in the KOH solution, which means that the powders milled for 60 h are more stable. The long time MA induces the alloying between titanium hydride and nickel.
Fig.2 shows that the displacement of Ni peak for the milled powders towards lower angles with increasing the milling time. This indicates that the lattice parameter of
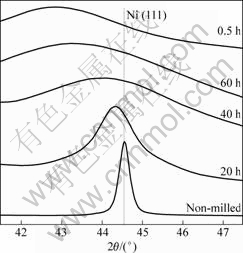
Fig.2 Displacement of Ni peak to lower angles for samples milled at 693 K for different time
Ni increased as the milling time increased due to the diffusion of Ti atoms, formed by the decomposition of TiH2, into the lattice of Ni, resulting in the formation of a Ni(Ti) solid solution. Similar results have been found in the Ti-Ni system of Refs.[13, 15]. No intermetallic phase was formed in TiH2-Ni system during MA. When the milling time was 60 h, the milled product is now predominantly amorphous with a small amount of TiH2 nanocrystalline phase.
3.2 Annealing treatment
Fig.3 shows the DTA and TG curves of the TiH2-Ni powders milled for 60 h. Two exothermic peaks were observed in the applied temperature region. NEVES et al[16] have proposed that in the MA process a great amount of defects were introduced into such as vacancy, dislocation and lattice strain. Therefore, structure relaxation should occur during the DTA test, leading to an exothermic reaction.
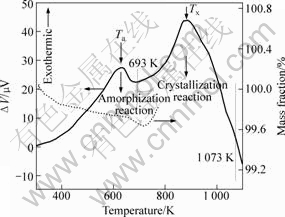
Fig.3 DTA and TG curves of TiH2-Ni powders milled for 60 h
An annealing treatment for the milled powders at 693 K for 0.5 h was carried out. It is interesting that the treated powders showed an amorphous structure characterized by XRD, as shown in Fig.1. This was confirmed by the result of TEM, as shown in Fig.4. The annealing resulted in the disappearance of the diffraction peak of TiH2 of the powders milled for 60 h, as shown in Fig.1. Fig.3 demonstrates that the mass of the powders decreased during the annealing in temperature region below 700 K. A subsequent increase of the mass may be attributed to an oxidation process. The mass loss may be ascribed to two causations, namely the decomposition of TiH2 and hydrogen desorption of the amorphous matrix. SUH et al[17] reported that hydrogen atoms could induce a transformation from nanocrystalline phase to amorphous phase. Similar hydrogen-induced amorphization has been mentioned earlier[18]. It is possibly that the decomposition of TiH2 and hydrogen diffusion in the alloy matrix destroy the long-range order structure during the annealing process, and thus lead to a rearrangement of metallic atoms into short-range order, resulting in the formation of amorphous phase. Moreover, the composition of the amorphous phase of the treated alloy is different from that of the alloy milled for 60 h according to the shift of diffraction peaks to lower angle, as shown in Fig.2. The annealing treatment at a high temperature, based on the second exothermic peak shown in Fig.3, contributes to the formation of Ti-Ni intermetallic compounds: Ti2Ni, TiNi and TiNi3, as shown in Fig.1. The alloy treated for 1 h has a predominant composition of Ti2Ni coexisting with minor phases of TiNi and TiNi3, while the alloy treated for a long time mainly consists of TiNi phase and shows an increase in TiNi3 phase but a drastic decrease in Ti2Ni phase. Perhaps, this is attributed to a higher stability for the short-range order structure corresponding to Ti-50%Ni (mole fraction), which requires a long time for a completion of crystallization.
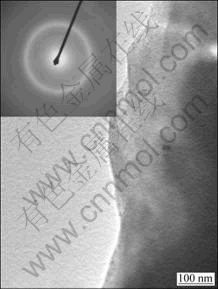
Fig.4 TEM image of TiH2-Ni powder milled for 60 h and subsequently annealed at 693 K for 0.5 h
Fig.5 shows the backscattered electron (BSE) images of the milled and subsequently annealed TiH2-Ni powders. It is well-known that cold-welding and fracture are the two essential processes involved in the milling process[19]. TiH2 and Ni particles were cold welded together by the energy introduced by MA, leading to the formation of a lamellar structure, as shown in Fig.5(a). It can be seen from Fig.5(b) that this lamellar structure disappeared and the elemental distribution in the particle was uniform when the powders were milled for 60 h. The result of XRD demonstrates that the annealing treatment promoted the internal diffusion and a short-range rearrangement of the milled powders at a low temperature, resulting in a completion of amorphization.
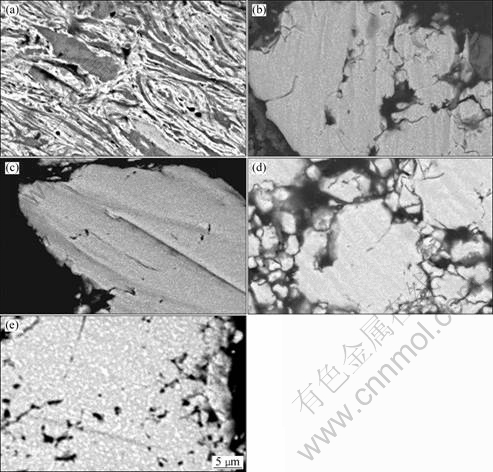
Fig.5 BSE images of milled and subsequently annealed TiH2-Ni powders: (a) Milling for 20 h; (b) Milling for 60 h; (c) Annealing at 693 K for 0.5 h; (d) Annealing at 1 073 K for 1 h; (e) Annealing at 1 073 K for 8 h
It might be that this rearrangement favors the atoms of Ti and Ni to form small clusters; these distributed clusters act as nucleation sites, and the nucleation grows up when a high temperature is applied. Fig.5(c) and Fig.5(d) show that no evident phase separation occurred by the annealing treatment at a low temperature or at a high temperature for a short time. However, the result of XRD shown in Fig.1 demonstrates that the alloy treated at 1 073 K for 1 h contains three phases. Therefore, it is probably that three phases are uniformly distributed and thus the corresponding BSE image presents no evident contrast. When the milled powders were annealed at a high temperature for a long time, Ni-rich phases were evidently separated from the matrix, as shown in Fig.5(e). Apparently, the white region is mainly composed of TiNi3 according to the result of XRD shown in Fig.1. The long time annealing treatment induces a phase transition among the Ti-Ni intermetallic compounds.
4 Conclusions
1) Mechanical milling induced a cold welding between TiH2 and Ni powders, leading to the formation of a lamellar structure in the initial milling process. The Ni(Ti) solid solution was formed during the milling process.
2) The elemental distribution of the milled powders tended to be uniform when a long time milling was applied. The powders milled for 60 h had an amorphous structure containing a few nanocrystalline TiH2 phases.
3) The annealing treatment at 693 K resulted in a completion of amorphization for the powder milled for 60 h. The treatment at a high temperature of 1 073 K contributed to a crystallization of the amorphous phase and, accordingly, the formation of Ti2Ni, TiNi and TiNi3. A long time treatment at 1 073 K induced the occurrence of phase separation and phase transition among these three compounds.
References
[1] OTSUKA K, REN X. Physical metallurgy of Ti-Ni-based shape memory alloys [J]. Prog Mater Sci, 2005, 50(5): 511-678.
[2] FIGUEIRA N, SILVA T M, CARMEZIM M J, FERNANDES J C S. Corrosion behaviour of NiTi alloy [J]. Electrochim Acta, 2009, 54(3): 921-926.
[3] WU S K, LIN H C. Damping characteristics of TiNi binary and ternary shape memory alloys [J]. J Alloys Compd, 2003, 355(1-2): 72-78.
[4] DRENCHEV B, SPASSOV T. Electrochemical hydriding of amorphous and nanocrystalline TiNi-based alloys [J]. J Alloys Compd, 2007, 441(1-2): 197-201.
[5] KITAMURA K, KUCHIDA T, INABA T, TOKUDA M, YOSHIMI Y. Shape memory characteristics of TiNi casting alloys made by using self-propagating high-temperature synthesis [J]. Mater Sci Eng A, 2006, 438-440: 675-678.
[6] HEY J C, JARDINE A P. Shape memory TiNi synthesis from elemental powders [J]. Mater Sci Eng A, 1994, 188(1-2): 291-300.
[7] GOH C W, GU Y W, LIM C S, TAY B Y. Influence of nanocrystalline Ni-Ti reaction agent on self-propagating high-temperature synthesized porous NiTi [J]. Intermetallics, 2007, 15(4): 461-467.
[8] CASTRO A T, CU?LLAR E L, M?NDEZ U O, YACAM?N M J. Crystal structure of TiNi nanoparticles obtained by Ar ion beam deposition [J]. Mater Sci Eng A, 2008, 481-482: 476-478.
[9] SURYANARAYANA C. Mechanical alloying and milling [J]. Prog Mater Sci, 2001, 46(1): 1-184.
[10] SCHWARZA R B, PETRICH R R. Calorimetry study of the synthesis of amorphous Ni-Ti alloys by mechanical alloying [J]. J Less Comm Met, 1988, 140: 171-184.
[11] YE L L, LIU Z G, RAVIPRASAD K, QUAN M X, UMEMOTO M, HU Z Q. Consolidation of MA amorphous NiTi powders by spark plasma sintering [J]. Mater Sci Eng A, 1998, 241(1-2): 290-293.
[12] SCHWARZA R B, PETRICH R R, SAW C K. The synthesis of amorphous Ni-Ti alloy powders by mechanical alloying [J]. J Non-Cryst Solids, 1985, 76(2-3): 281-302.
[13] GU Y W, GOH C W, GOI L S, LIM C S, JARFORS A E W, TAY B Y, YONG M S. Solid state synthesis of nanocrystalline and/or amorphous 50Ni-50Ti alloy [J]. Mater Sci Eng A, 2005, 392(1-2): 222-228.
[14] DRENCHEV B, SPASSOV T. Influence of B substitution for Ti and Ni on the electrochemical hydriding of TiNi [J]. J Alloys Compd, 2008, 474(1-2): 527-530.
[15] MOUSAVI T, KARIMZADEH F, ABBASI M H. Synthesis and characterization of nanocrystalline NiTi intermetallic by mechanical alloying [J]. Mater Sci Eng A, 2008, 487(1-2): 46-51.
[16] NEVES F, MARTINS I, CORREIA J B, OLIVEIRA M, GAFFET E. Reactive extrusion synthesis of mechanically activated Ti-50Ni powders [J]. Intermetallics, 2007, 15(12): 1623-1631.
[17] SUH D, DAUSKARDT R H. Hydrogen effects on the mechanical and fracture behavior of a Zr-Ti-Ni-Cu-Be bulk metallic glass [J]. Scripta Mater, 2000, 42(3): 233-240.
[18] MORI K, AOKI K, MASUMOTO T. Hydrogen-induced amorphization of titanium-based intermetallic compounds with the D019 structure [J]. J Alloys Compd, 1995, 231(1-2): 29-34.
[19] ZHOU J B, RAO K P. Structure and morphology evolution during mechanical alloying of Ti-Al-Si powder systems [J]. J Alloys Compd, 2004, 384(1-2): 125-130.
机械合金化及热处理对TiH2-Ni粉末结构和形貌的影响
赵相玉1,马立群1,丁 毅1,沈晓冬1, 2
1. 南京工业大学 材料科学与工程学院,南京210009;
2. 南京工业大学 材料化学工程国家重点实验室,南京210009
摘 要:研究机械合金化及热处理对TiH2-Ni粉末的结构和形貌的影响。在初始球磨阶段,Ni(Ti)固溶体形成。当球磨至60 h时,非晶结构的TiH2-Ni粉末形成,粉末中还包含少量的纳米晶TiH2相,粉末内元素分布均匀。球磨60 h的粉末经693 K热处理,发生了非晶化反应;经1 073 K短时间热处理后,非晶相结晶,形成Ti2Ni、TiNi和TiNi3相;在相同热处理温度下进一步延长热处理时间可使富镍相析出以及在3种Ti-Ni相之间发生相转变。
关键词:Ti-Ni合金;非晶合金;退火;微结构
(Edited by YANG Hua)
Foundation item: Project (BSCX200901) supported by the Doctorate Fellowship Foundation of Nanjing University of Technology, China
Corresponding author: MA Li-qun; Tel: +86-25-83587243; E-mail: maliqun@njut.edu.cn
DOI: 10.1016/S1003-6326(11)60744-9