
J. Cent. South Univ. (2020) 27: 1424-1431
DOI: https://doi.org/10.1007/s11771-020-4378-y

Modeling heap biooxidation of arsenic-bearing gold ore
LI Jia-feng(李佳峰)1, ZHONG Shui-ping(衷水平)2, TONG Lin-lin(佟琳琳)1,
ZHANG Deng-chao(张登超)3, BAO Dong-bin(鲍东斌)3, YANG Hong-ying(杨洪英)1
1. School of Metallurgy, Northeastern University, Shenyang 110819, China;
2. State Key Laboratory of Gold Extraction, Zijin Mining Group Co., Ltd., Shanghang 364200, China;
3. Xincheng Mining Co., Ltd., Guanhua Rare-precious Metals Group, Chizhou 247100, China
Central South University Press and Springer-Verlag GmbH Germany, part of Springer Nature 2020
Abstract: To design heap biooxidation process, it is necessary to understand its internal rules. The heap biooxidation of gold ore from Anhui province was researched in this study. The results showed that the main microorganisms in the heap were A. ferrooxidans, F. acidiphilum and L. ferrodiazotrophum. Under their combined action, gold leaching extent rose from 35.62% to 78.08% in 80 d. Boltzmann model matches the actual oxidation effect better and the model equations were obtained. The model predicted that the oxidation extents of arsenic and sulfur are 58.577% and 42.122% after one year, and the gold leaching extent was 80.40%. The arsenic and sulfur oxidation extents, and gold leaching extent were all linearly correlated. It is more reliable to predict gold leaching extent by sulfur oxidation extent. These results provided good guidance for practical application in the actual production.
Key words: heap biooxidation; gold ore; microbial community; fitting; correlativity
Cite this article as: LI Jia-feng, ZHONG Shui-ping, TONG Lin-lin, ZHANG Deng-chao, BAO Dong-bin, YANG Hong-ying. Modeling heap biooxidation of arsenic-bearing gold ore [J]. Journal of Central South University, 2020, 27(5): 1424-1431. DOI: https://doi.org/10.1007/s11771-020-4378-y.
1 Introduction
Heap biooxidation has advantages of low cost, pollution free and simple equipment. It has been successfully applied in the recovery of gold, copper, nickel and uranium, in Zijinshan copper ore and Newmont gold ore, etc [1-4]. Under the great pressure of low grade and environmental problems, it is an effective method to recover low grade refractory ore [5-7].
Heap biooxidation technology depends on the oxidation ability of microorganisms to decompose the minerals. The gold particles are exposed and can be dissolved into the solution for recycling [8]. In arsenic-bearing gold ore, pyrite (FeS2) and arsenopyrite (FeAsS) are usually the sulfide minerals in which the gold particles are enclosed [9]. Therefore, the gold leaching extent should be closely related to the oxidation extents of arsenic and sulfur. Heap biooxidation is usually carried out in a large scale in an open air for a long period of time. A deep understanding of the processes occurring in the heap is essential for parametric design [10], which can be achieved quickly and efficiently by model [11-13].
On the other hand, the growth of microorganisms requires an adaptation period after inoculating to the heap [14]. At this stage, microorganisms adapt to the environment and attach to the ore surface. The expected oxidation effect could not be achieved quickly [15, 16]. After the adaptation of the organisms to the environment, the decomposition of the metal minerals commences effectively. Elements such as arsenic and sulfur enter the solution in the form of ions. When oxidation process is carried out to a certain extent, there is a limited growth rate for microorganisms [17]. It is more difficult for bacterial liquid to enter ore particles. Oxidation rates tend to flatten in later stages. Based on the above description, heap biooxidation has the characteristic of “slow-fast-slow”. The oxidation extents of arsenic and sulfur cannot reach 100% in the effective time. The feature plays an important guiding role in the selection of model.
In this study, the model and the correlation in the heap biooxidation process of arsenic-bearing gold ore were studied. The purpose is the quantitative prediction of oxidation effect and the revelation of inner connections between different elements. In this way, short, simple and effective suggestions for production are proposed.
2 Materials and methods
2.1 Heap description
Truck was selected for the construction of the heap with a mass of 15000 t. The heap was 100 m long, 35 m wide and 2-4 m high. There was a slope of 5% along the length direction, and aeration tube was arranged at the bottom. Intermittent spraying method was adopted for irrigation (spraying for 8 h and stopping for 16 h). The experiment lasted for 80 d. Every 10 d, the ore samples in the heap were selected for testing, and the sampling points showed a distribution of 5 m × 7 m.
2.2 Ore properties
The ore sample used in this study was collected from Auhui, China. The gold ore belongs to low grade porphyritic arsenic-bearing gold ore with a gold content of 0.85 g/t, arsenic content of 0.19%, sulfur content of 2.27% and iron content of 3.21%. Particle size distribution of the ore after crushing by a roller was 0-1 mm accounting for 27.76%, 1-2 mm for 19.55%, 2-3.35 mm for 14.36%, 3.35-5 mm for 17.12%, and 5-10 mm for 21.21%. XRD (Figure 1) and microscopic (Figure 2) analyses of the ore showed that the main minerals were pyrite, arsenopyrite, feldspar, quartz and mica. Gold particles were heavily encapsulated and the direct leaching extent was only 36%.
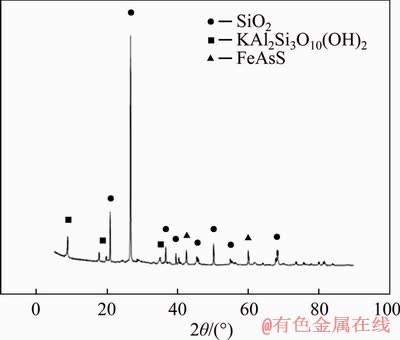
Figure 1 X-ray diffraction pattern of gold ore
2.3 Inoculated strain and medium
Mix microbial culture (designated HQ0211) was provided by Northeastern University Bio-metallurgical Laboratory. The main strains of the inoculated medium were A. ferrooxidans, F. acidiphilum and L. ferriphilum. 9K culture medium was used for the experiment, and the related compositions of the 9K medium were: (NH4)2SO4 3.0 g/L, KCl 0.1 g/L, K2HPO4 0.5 g/L, MgSO4·7H2O 0.5 g/L, Ca(NO3)2 0.01 g/L, FeSO4·7H2O 44.3 g/L. They were dissolved in distilled water and sulfuric acid was used to regulate the pH value. The bacterial liquid was gradually cultured to 1000 m3 with inoculation amount of 10% and initial pH of 1.6.
2.4 Detection methods
The ore samples were leached by using environmental gold extracting agent in a pH range of 11-12. Arsenic content was determined by inductively coupled plasma method and sulfur content by tube furnace. Gold content was determined by foam plastic adsorption-atomic absorption method. Microbial community detection was performed by 16S rRNA high-throughput sequencing method conducted by GENEWIZ (Suzhou) company (NCBI accession number: SRP193408).
2.5 Correlation analysis
Origin (8.0) software was used to analyze the correlation between the oxidation extents of arsenic, sulfur and the leaching extent of gold by linear regression Anova. P value of F-test was obtained to evaluate the correlation. Pearson correlation coefficient, r, was calculated on the basis of P<0.01 to evaluate the correlation degree.
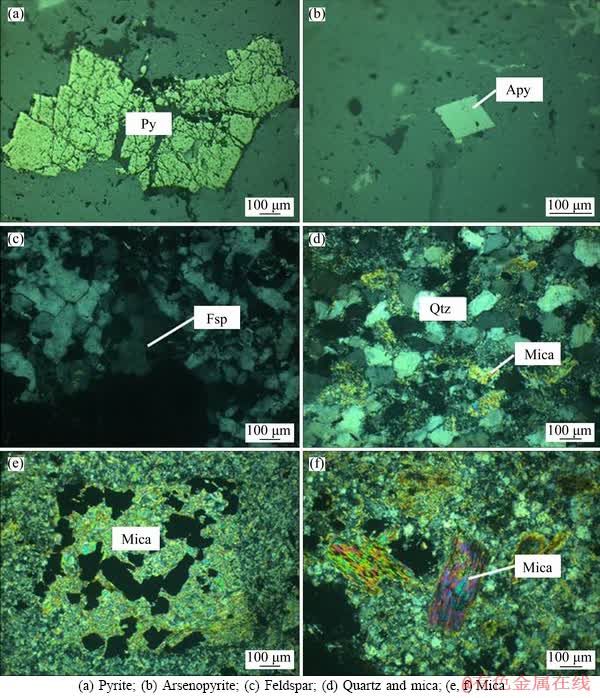
Figure 2 Morphology of major minerals in gold ore:
(1)
where N is sample size, here is 9; x is the independent variable, indicating the oxidation extent of arsenic or sulfur; y is the dependent variable, indicating the gold leaching extent or S oxidation extent.
2.6 Presentation of models
Based on the characteristic of “slow-quick- slow”, it was assumed that the changes of arsenic and sulfur oxidation extents and gold leaching extent were in line with Boltzmann model (Eq. (2)) and Logistic model (Eq. (3)). The data obtained from the ore samples were fitted according to the two models. Therefore, the fitting effects were evaluated and applied.
Boltzmann model:
(2)
Logistic model:
(3)
where x is the independent variable, indicating the oxidation time; y is the dependent variable, indicating the oxidation extent of arsenic, sulfur or gold leaching extent; A1, A2, x0, p and dx are all model parameters.
3 Results and discussion
3.1 Microbial community in heap
Microorganisms are the key to biometallurgy. However, they can be affected by the environment due to their inherent life attributes. Therefore, studying microbial community in a specific environment is a good guidance for improvement of bioleaching [18, 19]. The heap was a medium temperature environment, and the pH of bacterial liquid was 1.5-2.1. The microbial community in the heap had a high alpha diversity: the abundance of Ace was 29, the abundance of chao1 was 28, the abundance of shannon was 2.6, the abundance of simpson was 0.727, and the abundance of goods coverage was 1.
Figure 3 represents the microbial classification result obtained by 16S rRNA high throughput sequencing. With the refinement of classification level, the proportion of major microorganisms can be detected to decrease gradually. It is not a sign of low abundance, but of a concentration of a few microbes.
Due to the harsh environment of the heap, some microorganisms were eliminated due to their inability to adapt to the leaching environment. The maim species were A. ferrooxidans, F. acidiphilum and L. ferrodiazotrophum. They all can oxidize Fe2+, but only the former is the sulfur-oxidizers. Some researchers have demonstrated that a large proportion of sulfur-oxidizers can weaken the formation of passivation layer (sulfur membrane) [20]. A. caldus has a strong adaptability and sulfur oxidation ability, and its inoculation into the heap may speed up the process [21]. L. ferrodiazotrophum didn’t exist in HQ0211 strain but came from the environment. The L. ferrodiazotrophum strains accumulated in the heap and played a significant role in nitrogen- fixation process [22, 23].
3.2 Heap biooxidation results
After eighty days of heap biooxidation, arsenic concentration in the bacterial medium was 2 g/L, sulfate radical concentration increased from 19.6 to 37.6 g/L, and iron concentration increased from 6.4 to 15.6 g/L. Due to the process proceeded outdoors, the bacterial medium was diluted with rain water. On one hand, the index of bacterial liquid would be affected, leading to reduction of the microbial activity. Therefore, pH was adjusted and iron-free 9K medium was added into the 500 m3 reservoir duly. On the other hand, it is impossible to measure the volume of rain accurately, and then the oxidation effect was evaluated by the ore sample. Table 1 lists the arsenic, sulfur oxidation extents and gold leaching extent during the process.
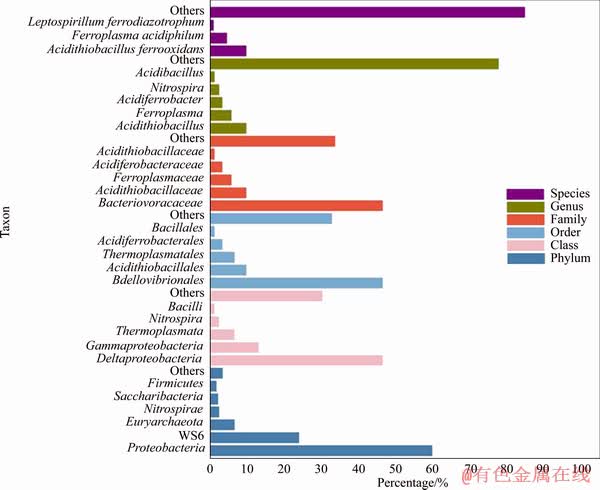
Figure 3 Microbial taxon at different levels
Table 1 Arsenic, sulfur oxidation extents and gold leaching extent during heap biooxidation process
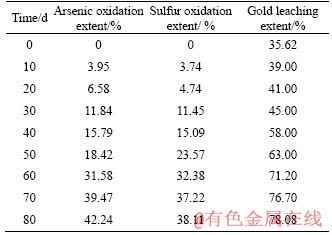
3.3 Correlation analysis
According to biooxidation mechanism of pyritic and arsenopyritic-containing gold ore, pyrite and arsenopyrite are decomposed during the process. Arsenic and sulfur are released into liquid phase and gold particles are exposed to leaching agent [24]. They should be related to each other to some extent by fitting them in pairs based on Table 1, and the fitted curve can be seen in Figures 4-6. Then, P value of F-test and Pearson correlation coefficient r (Table 2) were obtained for significance and correlation analysis.
Because the P values are all less than 0.01, the r can be used to analyze the significance of the relationship, that is, the oxidation extents of arsenic, sulfur and the leaching extent of gold are all linearly correlated, which conforms to the biooxidation mechanism. Besides, because r>r0.01=0.798, they are highly correlated. Therefore, gold leaching extent can be predicted by arsenic and sulfur oxidation extents. It is worth noting that the r value of gold-arsenic is less than that of gold-sulfur, indicating that the prediction of gold leaching extent by sulfur oxidation extent is more reliable.
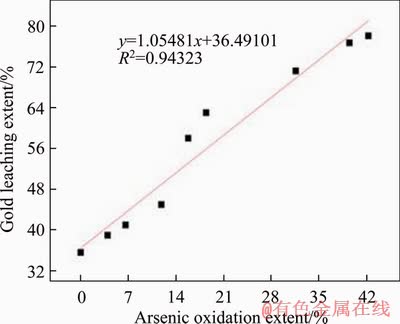
Figure 4 Gold-arsenic linear fitting curve
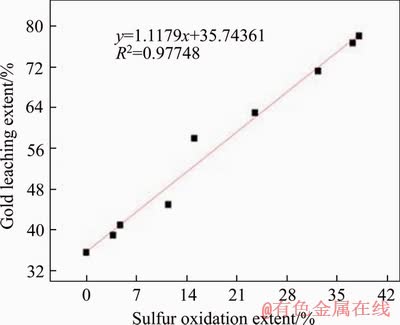
Figure 5 Gold-sulfur linear fitting curve
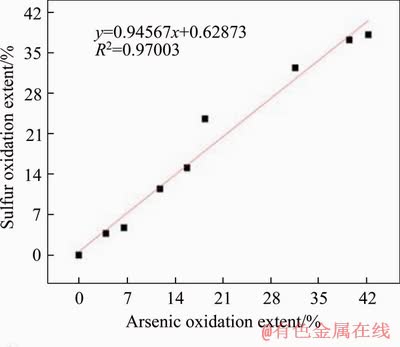
Figure 6 Sulfur-arsenic linear fitting curve
Table 2 P value and r value of linear fitting
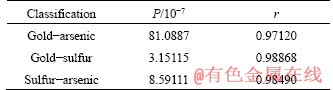
3.4 Predictive model analysis
Although the inoculated HQ0211 microbial strain has been domesticated for a long time, it still needs to adapt to the new environment of the heap [25]. According to Table 1, arsenic, sulfur oxidation extents and gold leaching extent are in line with the characteristic of “slow-fast-slow”. Boltzmann and logistic model fittings were applied (Figures 7-9, Table 3), and the predicted results were compared and analyzed.
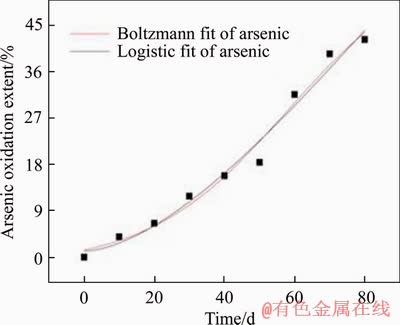
Figure 7 Fitted curve of arsenic oxidation extent
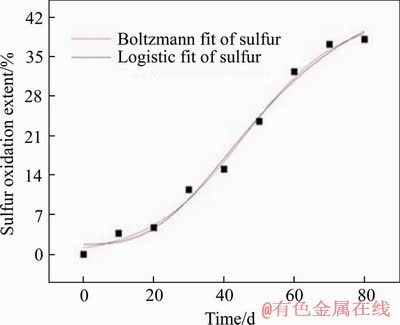
Figure 8 Fitted curve of sulfur oxidation extent
R2 values of both models are greater than 0.96 during the heap biooxidation process of eighty days. That means that both models can be used to predict the oxidation extents of arsenic and sulfur, and gold leaching extent well. However, Boltzmann model presents better result due to its higher R2. In addition, the two models were also used to predict the oxidation effect after one year (365 d), and there was inconsistence in the result with the facts for logistic model. The arsenic oxidation extent was 192.77%. Therefore, Boltzmann model (Eq. (4)-Eq. (6)) is more suitable for predicting heap biooxidation of the gold ore. It was calculated that the oxidation extents of arsenic and sulfur, and gold leaching extent are 58.577% and 42.122%, and 80.40%, respectively, after one year. There was no significant increase. Therefore, it is right to set the oxidation period as eighty days. In this case, the recovery of gold and minimizing production costs are guaranteed. The remaining 20% of the gold that still couldn’t be leached might be wrapped in the insoluble gangue minerals, making it impervious to the leaching agent.
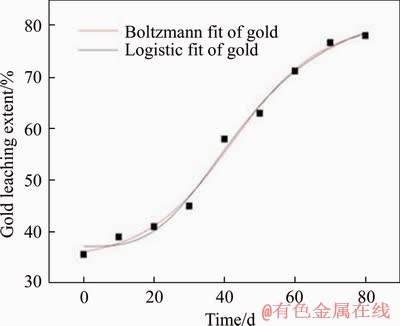
Figure 9 Fitted curve of gold leaching extent
Table 3 Fitting parameters of two models
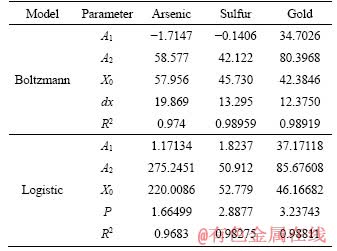
Boltzmann model of arsenic oxidation extent:
(4)
Boltzmann model of sulfur oxidation extent:
(5)
Boltzmann model of gold leaching extent:
(6)
The arsenic and sulfur oxidation extents (58.577% and 42.122%) obtained by this model after one year of oxidation process were substituted into the correlation linear equation in Section 3.3. It was found that the gold leaching extents were 98.28% and 82.83%, respectively. This confirmed that sulfur oxidation extent can predict gold leaching extent more accurately. Therefore, it can be concluded that gold leaching extent can be predicted by sulfur oxidation extent through Boltzmann model and regression equation.
4 Conclusions
1) According to 16S rRNA analysis, the microorganisms in the heap were iron-oxidizers mostly, including A. ferrooxidans, F. acidiphilum and L. ferrodiazotrophum.
2) Under the synergic action of microorganisms, the gold leaching extent of the gold ore rose from 36% to 78% in 80 d.
3) The P values between arsenic and sulfur oxidation extents, and gold leaching extent are all very small, and the r values are all close to 1, which indicates that they are linearly correlated with each other. Based on the r values, it is more reliable to predict gold leaching extent by sulfur oxidation extent.
4) The model analysis results indicated that heap biooxidation process conforms to Boltzmann model, which can make a good assessment of oxidation effect in the future. Arsenic and sulfur oxidation extents, and gold leaching extent should be 58.577%, 42.122% and 80.40%, respectively, after one year later.
5) Through the trend of gold leaching extent obtained from Boltzmann model, the appropriate heap biooxidation period should be 80 d.
References
[1] CERDA J, GONZALEZ S, RIOS J M, QUINTANA T. Uranium concentrates bioproduction in Spain: A case study [J]. FEMS Microbiology Reviews, 1993, 11(1-3): 253-259. DOI: https://doi.org/10.1111/j.1574-6976.1993.tb00292.x.
[2] RIEKKOLA-VANHANEN M. Talvivaara mining company—From a project to a mine [J]. Minerals Engineering, 2013, 48: 2-9. DOI: https://doi.org/10.1016/ j.mineng.2013.04.018.
[3] ROBERTO F F. Commercial heap biooxidation of refractory gold ores—Revisiting Newmont’s successful deployment at Carlin [J]. Minerals Engineering, 2017, 106: 2-6. DOI: https://doi.org/10.1016/j.mineng.2016.09.017.
[4] LIU Xing-yu, CHEN Bo-wei, CHEN Jing-he, ZHANG Ming-jiang, WEN Jian-kang, WANG Dian-zuo, RUAN Ren-man. Spatial variation of microbial community structure in the Zijinshan commercial copper heap bioleaching plant [J]. Minerals Engineering, 2016, 94: 76-82. DOI: https://doi.org/10.1016/j.mineng.2016.05.008.
[5] BRIERLEY C L. How will biomining be applied in future? [J]. Transactions of Nonferrous Metals Society of China, 2008, 18(6): 1302-1310. DOI: https://doi.org/10.1016/ s1003-6326(09)60002-9.
[6] JALALI F, FAKHARI J, ZOLFAGHARI A. Response surface modeling for lab-scale column bioleaching of low-grade uranium ore using a new isolated strain of Acidithiobacillus Ferridurans [J]. Hydrometallurgy, 2019, 185: 194-203. DOI: https://doi.org/10.1016/j.hydromet. 2019.02.014.
[7] TAVAKOLI H Z, ABDOLLAHY M, AHMADI S J, DARBAN A K. Enhancing recovery of uranium column bioleaching by process optimization and kinetic modeling [J]. Transactions of Nonferrous Metals Society of China, 2017, 27(12): 2691-2703. DOI: https://doi.org/10.1016/S1003- 6326(17)60298-X.
[8] ASPIAZU C L, AGUIRRE P, HEDRICH S, SCHIPPERS A. Microbial community analysis inside a biooxidation heap for gold recovery in equador [J]. Solid State Phenomena, 2017, 262: 135-138. DOI: https://doi.org/10.4028/www.scientific. net/SSP.262.135.
[9] HU Jie-hua, HUANG Huai-guo, XIE Hong-zhen, GAN Li-hui, LIU Jian, LONG Min-nan. A scaled-up continuous process for biooxidation as pre-treatment of refractory pyrite-arsenopyrite gold-bearing concentrates [J]. Biochemical Engineering Journal, 2017, 128: 228-234. DOI: https://doi.org/10.1016/j.bej.2017.10.001.
[10] BOUFFARD S C, DIXON D G. Modeling the performance of pyritic biooxidation heaps under various design and operating conditions [J]. Hydrometallurgy, 2009, 95(3, 4): 227-238. DOI: https://doi.org/10.1016/j.hydromet.2008. 06.002.
[11] MCBRIDE D, GEBHARDT J E, CROSS M. A comprehensive gold oxide heap leach model: Development and validation [J]. Hydrometallurgy, 2012, 113-114: 98-108. DOI: https://doi.org/10.1016/j.hydromet.2011.12.003.
[12] ROBERTSON S. Development of an integrated heap leach solution flow and mineral leaching model [J]. Hydrometallurgy, 2017, 169: 79-88. DOI: https://doi.org/ 10.1016/j.hydromet.2016.12.010.
[13] MCBRIDE D, CROFT T N, CROSS M, BENNETT C, GEBHARDT J E. Optimization of a CFD—Heap leach model and sensitivity analysis of process operation [J]. Minerals Engineering, 2014, 63: 57-64. DOI: https://doi.org/10.1016/j.mineng.2013.11.010.
[14] ROLFE M D, RICE C J, LUCCHINI S, PIN C, THOMPSON A, CAMERON A D S, ALSTON M, STRINGER M F, BETTS R P, BARANYI J, PECKM W, HINTONA J C D. Lag phase is a distinct growth phase that prepares bacteria for exponential growth and involves transient metal accumulation [J]. Journal of Bacteriology, 2012, 194(3): 686-701. DOI: https://doi.org/10.1128/ jb.06112-11.
[15] SOTO P, MENESES C, COMTADOR Y, GALLEGUILLOS P, DEMERGASSO C, SERON M. Characterization of oxidizing activity of a microbial community in an industrial bioleaching heap [J]. Advanced Materials Research, 2009, 71-73: 59-62. DOI: https://doi.org/10.4028/www.scientific. net/AMR.71-73.59.
[16] GAO Qi-yu, TANG De-ping, SONG Peng, ZHOU Jian-ping, LI Hong-yu. Characterization of acylated homoserine lactone derivatives and their influence on biofilms of Acidithiobacillus ferrooxidans BY-3 under arsenic stress [J]. Journal of Central South University, 2020, 27(1): 52-63. DOI: https://doi.org/10.1007/s11771-020-4277-2.
[17] MA Li-yuan, WANG Hong-mei, WU Jiang-jun, WANG Yu-guang, ZHANG Du, LIU Xuan-duan. Metatranscriptomics reveals microbial adaptation and resistance to extreme environment coupling with bioleaching performance [J]. Bioresource Technology, 2019, 280: 9-17. DOI: https://doi.org/10.1016/j.biortech.2019.01.117.
[18] HE Zhi-guo, GAO Feng-ling, ZHAO Jian-cun, HU Yue-hua, QIU Guan-zhou. Insights into the dynamics of bacterial communities during chalcopyrite bioleaching [J]. FEMS Microbiology Ecology, 2010, 74(1): 155-164. DOI: https://doi.org/10.1111/j.1574-6941.2010.00943.x.
[19] WANG Jun, ZHU Shan, ZHANG Yan-sheng, ZHAO Hong-bo, HU Ming-hao, YANG Cong-ren, QIN Wen-qing, QIU Guan-zhou. Bioleaching of low-grade copper sulfide ores by Acidithiobacillus ferrooxidans and Acidithiobacillus thiooxidans [J]. Journal of Central South University, 2014, 21: 728-734. DOI: https://doi.org/10.1007/s11771-014- 1995-3.
[20] HEDRICH S, JOULIAN C, GRAUPNER T, SCHIPPERS A, GUEZENNEC A G. Enhanced chalcopyrite dissolution in stirred tank reactors by temperature increase during bioleaching [J]. Hydrometallurgy, 2018, 179: 125-131. DOI: https://doi.org/ 10.1016/j.hydromet.2018.05.018.
[21] WANG Zhao-bao, LI Ya-qing, LIN Jian-qun, PANG Xin, LIU Xiang-mei, LIU Bing-qiang, WANG Rui, ZHANG Cheng-jia, WU Yan, LIN Jian-qiang, CHEN Lin-xu. The two-component system RsrS-RsrR regulates the tetrathionate intermediate pathway for thiosulfate oxidation in Acidithiobacillus caldus [J]. Frontiers in Microbiology, 2016, 7: 1-15. DOI: https://doi.org/10.3389/ fmicb.2016.01755.
[22] GARCIA-MOYANO A, GONZALEZ-TORIL E, MORENO-PAZ M, PARRO V, AMILS R. Evaluation of Leptospirillum spp. in the Río Tinto, a model of interest to biohydrometallurgy [J]. Hydrometallurgy, 2008, 94(1-4): 155-161. DOI: https://doi.org/10.1016/j.hydromet.2008. 05.046.
[23] LI Jia-feng, TONG Lin-lin, XIA Yu, YANG Hong-ying, SAND Wolfgang, XIE Hong-zhen, LAN Bi-bo, ZHONG Shui-ping, AUWALU Ali. Microbial synergy and stoichiometry in heap biooxidation of low-grade porphyry arsenic-bearing gold ore [J]. Extremophiles, 2020, 24(3): 355-364. DOI: https://doi.org/10.1007/s00792-020-01160-6.
[24] MARCHEVSKY N, URBIETA M S, BERNARDELLI C, MAS M, DONATI E R. Zinc recovery during refractory ore biooxidation by an indigenous consortium [J]. International Journal of Mineral Processing, 2015, 138: 30-37. DOI: https://doi.org/10.1016/j.minpro.2015.03.009.
[25] RUAN Ren-man, ZOU Gang, ZHONG Shui-ping, WU Zeng-ling, CHAN B K C, WANG D Z. Why Zijinshan copper bioheapleaching plant works efficiently at low microbial activity—Study on leaching kinetics of copper sulfides and its implications [J]. Minerals Engineering, 2013, 48: 36-43. DOI: https://doi.org/10.1016/j.mineng.2013. 01.002.
(Edited by YANG Hua)
中文导读
含砷金矿生物堆浸氧化的模拟研究
摘要:为了优化生物堆浸氧化设计,必须深入了解内在规律。本研究对安徽省某含砷金矿生物堆浸氧化过程进行分析讨论,结果表明:堆中主要微生物为A. ferrooxidans, F. acidiphilum和L. ferrodiazotrophum等。在它们的协同作用下,80天后堆中矿石金浸出率由35.62%升高至78.08%。Boltzmann模型对实际氧化结果的拟合更佳,并得出了相应的模型方程。用模型预测1年后砷、硫氧化率分别为58.577%和42.122%,金浸出率为80.40%。分析结果表明砷氧化率、硫氧化率和金浸出率均为线性相关关系,用硫氧化率预测金浸出率更精确。这些结果为生物堆浸氧化的实际应用提供了很好的指导。
关键词:生物堆浸氧化;金矿;微生物群落;拟合;相关关系
Foundation item: Project(U1608254) supported by the Special Fund for the National Natural Science Foundation of China; Projects(ZJKY2017(B)KFJJ01, ZJKY2017(B)KFJJ02) supported by the Zijin Mining Group Co., Ltd, China
Received date: 2019-06-30; Accepted date: 2020-04-12
Corresponding author: YANG Hong-ying, PhD, Professor; Tel: +86-24-83673932; E-mail: yanghy@smm.neu.edu.cn