J. Cent. South Univ. Technol. (2011) 18: 1359-1364
DOI: 10.1007/s11771-011-0846-8
Microstructure and microwave dielectric properties of CaO-B2O3-SiO2 glass ceramics with various B2O3 contents
WEI Peng-fei(韦鹏飞)1, 2, ZHOU Hong-qing(周洪庆)1, ZHU Hai-kui(朱海奎)1,
DAI Bin(戴斌)1, WANG Jie(王杰)1
1. College of Materials Science and Engineering, Nanjing University of Technology, Nanjing 210009, China;
2. College of Materials Engineering, Jinling Institute of Technology, Nanjing 211169, China
? Central South University Press and Springer-Verlag Berlin Heidelberg 2011
Abstract: The effects of B2O3 addition on both the sintering behavior and microwave dielectric properties of CaO-B2O3-SiO2 (CBS) glass ceramics were investigated by Fourier transform infrared spectroscopy (FTIR), X-ray diffractometry (XRD) and scanning electron microscopy (SEM). The results show that the increasing amount of B2O3 causes the increase of the contents of [BO3], [BO4] and [SiO4], which deduces the increase of CaB2O4 and α-SiO2 and the decrease of CaSiO3 correspondingly. No new phase is observed throughout the entire experiments. A bulk density of 2.54 g/cm3, a thermal expansion coefficient value of 11.95×10-6 °C -1 (20-500°C), a dielectric constant εr value of 6.42 and a dielectric loss tan δ value of 0.000 9 (measured at 9.7 GHz) are obtained for CBS glass ceramics containing 35% B2O3 (mass fraction) sintered at 850 °C for 15 min.
Key words: CaO-B2O3-SiO2; B2O3 content; Fourier transform infrared spectroscopy; microstructure; dielectric properties
1 Introduction
In the development of co-fired multilayer circuits (CMC), low temperature co-fired ceramic (LTCC) played a decisive role as a base material. The LTCC is crucial in the development of various modules and substrates. This technology combines many thin layers of ceramics and conductors resulting in multilayer LTCC modules and is generally used in the form of a three-dimensional (3D) wiring circuit board today, using low permittivity (usually εr<4-9) dielectric components. Additionally, it enables a versatile mixture of passive microwave components such as micro-strips, strip lines and dividers. Furthermore, these integrated components are interconnected with 3D strip line circuitry [1]. Calcium borosilicate glass (CaO-B2O3-SiO2; CBS), as one of the most important LTCC substrate materials, was reported in many literatures [2-4]. It was sintered at temperatures below 900 °C, and offered low dielectric loss characters and low thermal expansion coefficient (close to Si and Ga/As). Additionally, it is very robust against environmental stress. Several studies had shown that the addition of B2O3 in microwave materials can perform the advantages of lowering sintering temperatures and improving the dielectric properties [5-8]. However, as one of the most common glass- formers [9], vitreous B2O3 has a structure consisting of a random network of boroxol rings and [BO3] triangles connected by B-O-B linkages [10]. It was reported that the addition of a network modifier in borate glasses could produce the conversion of the triangular [BO3] structural units to [BO4] tetrahedral units with a coordination number of four [11]. Researchers concluded that B existed in glass with the coordination polyhedron of [BO3] and [BO4], and the “boron abnormality” phenomenon might arise [12-13]. The main objective of this work is to investigate the microstructure of CBS glass ceramics with B2O3 addition. The variations of the microwave dielectric properties were also investigated in terms of the microstructural changes.
2 Experimental
The composition of the glass was determined according to the phase diagram of the CBS systems. The glass contained 25%-40% CaO, 25%-40% SiO2, 15%- 40% B2O3, P2O5 and 2% ZnO (all in mass fraction) in total. CaCO3, SiO2 and H3BO3 of reagent grade were chosen as the raw materials. So, the composition chosen for the experimental samples was listed in Table 1. They were dry-mixed in polyethylene jars with zirconia balls for 8 h. After being mixed uniformly, the powders were put into Pt crucible and molten in the temperature range of 1 400-1 700 °C in air. It was held at melting temperature for 30-120 min. Then, the molten glass was quenched into de-ion water. The cullets were dried and milled with d 5 mm zirconia balls to obtain an average particle size less than 5 μm. Furthermore, the powders prepared above were mixed with an amount of polyvinyl alcohol (12% PVA, mass fraction), pressed under a pressure of 100 MPa to obtain green compacts with diameter of 13 mm and thickness of 4-10 mm, and then followed by sintering at the temperatures between 800 °C and 925 °C for 15 min with a heating rate of 5 °C/min. In order to understand the effects of glass composition on the sintering characteristic, the powders were pressed under a pressure of 100 MPa to obtain green compacts with length of 40 mm, width of 4 mm and thickness of 5 mm. Dilatometric analysis was performed to characterize the shrinkage of the glass with respect to temperature. Experiments were performed with a DIL 402C dilatometer in air and at a heating rate of 5 °C/min.
Table 1 Composition of different CBS glasses
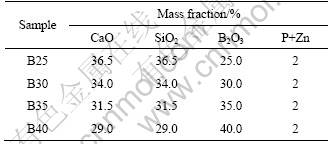
The IR spectra of the glass ceramic samples were recorded at room temperature using the KBr disc technique. The samples were ground into fine powders. Then, 2 mg glass powders and 200 mg KBr powders were accurately weighed for semiquantitative analysis of the glass structure. A Fourier transform infrared spectroscope (FTIR, Nicolet Nexus, Thermo Nicolet) was used and the wave number was ranged from 400- 1 500 cm-1. The phase composition of the samples was determined by X-ray diffraction with Cu Kα radiation (XRD ARL X/TRA). The crystal structures were examined by scanning electron microscopy (SEM JSM-5900). Bulk density of the fired samples on the CBS glass ceramics were measured by Archimedean immersion method using water as media with the accuracy of ±0.001 g/cm3. The dielectric properties such as dielectric constant (εr) and dielectric loss (tan δ) were measured at 10 GHz by network analyzer (8722ET, Agilent). Cylindrical-shaped samples with an aspect ratio (diameter/height) ≥1 were used to chock off other interfering modes. The samples were positioned inside the circular cavity with good contact to the top and bottom plates. Dielectric constant and tan δ were calculated from the frequency of the TM010 resonant mode.
3 Results and discussion
3.1 Effect of B2O3 on FTIR absorption spectra of CBS glass ceramics
FTIR spectroscopy was used to obtain the essential information concerning the arrangement of the structural units of the studied glass ceramics. The experimental FTIR spectra of the CBS glass ceramics with various contents of B2O3 are presented in Fig.1.
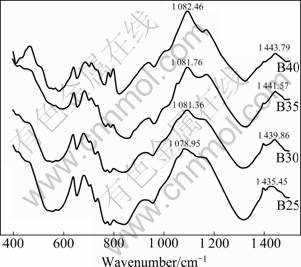
Fig.1 FTIR spectra of CaO-xB2O3-SiO2 glass ceramics with 25%≤x≤40% (mass fraction)
It is known that in the vitreous B2O3 about 80% of the boron atoms are presented in the B3O6 boroxol rings, which are interconnected by independent [BO3] groups [14]. Thus, the vibrational modes of the vitreous borate network are mainly active in three infrared spectral regions. The IR features located in the first region that ranges between 1 200 and 1 500 cm-1 are due to the asymmetric stretching relaxation of B—O bonds from the [BO3] trigonal units. The second region ranging between 800 cm-1 and 1 200 cm-1 and its spectral features are due to the B—O bond stretching of [BO4] tetrahedral units. In the third region ranging from 400 cm-1 to 800 cm-1, there is an important band located around 700 cm-1 assigned to the bending vibrations of various borate segments [14-15]. The bands observed in these FTIR spectra and their assignments are summarized in Table 2 [16-17]. The most obvious change at about 700, 1 080 and 1 440 cm-1 in Fig.1 is that the vibrating bands gradually become stronger. This indicates that the amount of [BO3] and [BO4] increases with the increasing of B2O3 content. At the same time, the vibrating bands of the B40 sample at about 460 cm-1 gradually become stronger. The addition of CaO as a network modifier, can offer free oxygen. So, when the free oxygen is full enough, [BO3] has priority to associate itself with free oxygen to form [BO4]. The rest of the free oxygen can associate with SiO2, and the free oxygen mainly serves to destroy Si—O—Si and to form non-bridging oxygen. The amount of [BO3] increases with the content of boron, and then, the amount of [BO4] increases. The free oxygen in the systems is overused by [BO3] trigonal units to form [BO4] tetrahedral units, the amount of free oxygen decreases, and then the amount of [SiO4] increases. This can explain the fact that the position of these bands are shifted to the higher frequencies.
Table 2 Assignment of IR absorbance spectra of samples
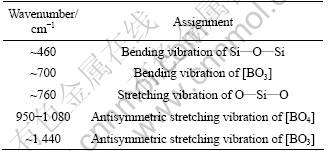
3.2 Effect of B2O3 on XRD results of CBS glass ceramics
The X-ray diffraction patterns of the sintered samples with different amounts of B2O3 are illustrated in Fig.2. The main crystalline phases are composed of CaB2O4, CaSiO3 and α-SiO2. The major crystalline phases of the four samples do not change, irrespective of the amount of B2O3 additions. But the amount of each crystalline phase varies greatly. It is noticed that the intensity of the main crystal diffraction peaks at 2θ=26.6° and 29.3° increase with increasing the B2O3 content. The position and intensity of the diffraction peaks are analyzed using powder diffraction files (PDF) and are found to be characteristic of CaB2O4 and α-SiO2 crystalline phases. However, the intensity of diffraction peaks of the CaSiO3 crystalline phases at 2θ=28.4° decreases with increasing the B2O3 content. The facts can be explained by FTIR spectra of the CBS glass ceramics systems with various contents of B2O3 in Fig.1. From the previous FTIR spectra analysis, [BO3], [BO4] and [SiO4] increase with the increase of B2O3 content. Ca ions have priority to associate with [BO3] and [BO4] to form CaB2O4 crystalline phases due to its larger ionic strength. So, the rest of Ca ions combines with [SiO4] to form CaSiO3 crystalline phases. Without enough Ca ions, [SiO4] associates with itself to form α-SiO2 crystalline phases.
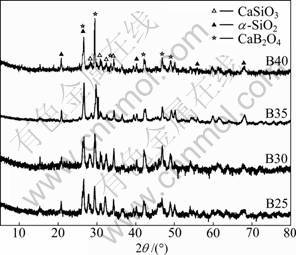
Fig.2 XRD results of CaO-xB2O3-SiO2 glass ceramics with 25%≤x≤40% (mass fraction)
3.3 Effect of B2O3 on sintered and dielectric properties of CBS glass ceramics
Figure 3 shows the SEM micrographs of the fractured surface of CaO-xB2O3-SiO2 glass ceramics with 25%≤x≤40% (mass fraction) sintered at 900, 870, 850 and 830 °C for 15 min, respectively. It is indicated that B2O3 is not only a glass networker, but also a good sintering aid, which is able to reduce the sintering temperature of the glass ceramics [18-19]. The B25 sample sintered at 900 °C is more porous in Fig.3(a). With the increasing addition of B2O3, the pore size and porosity decrease obviously (Figs.3(b)-(d)). At the same time, the grain size decreases with the increase of B2O3 amount. This is due to the structural changes from [BO3] to [BO4] as the content of the glass networker B2O3 increases. The [BO3] groups in the borosilicate glasses prefer a coordination change to [BO4] rather than producing non-bridging oxygen. This structural change in [BO4] will increase the stability of the glasses. It can prevent the glass phases from converting to the crystalline phases. So, the grain size of the B40 sample in Fig.3(d) is smaller than others. The more the B2O3 is added, the more the glass phases form. The B40 sample has the most glass phases in all samples. This can be accompanied by the bulk density variation of the samples with different B2O3 contents.
Figure 4 shows the variation in the bulk density of the CBS glass ceramics as a function of the B2O3 content. When the content of B2O3 increases from 25% to 35%, the bulk density considerably increases up to 2.54 g/cm3 from 2.40 g/cm3. But the bulk density decreases slightly when the B2O3 content is 40%. The SEM images reveal that the microstructure of the samples becomes dense with the increasing addition of B2O3. However, when the amount of B2O3 content is more than 35%, the bulk density of the sintered samples declines to 2.47 g/cm3. This is attributed to the formation of too much glass phases.
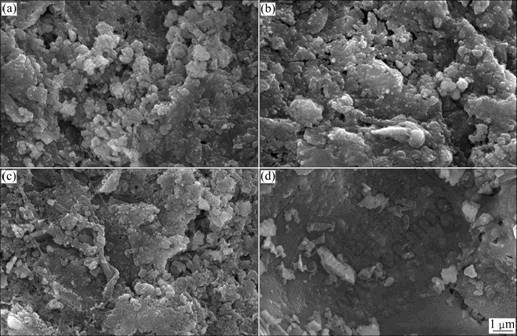
Fig.3 SEM micrographs of CaO-xB2O3-SiO2 glass ceramics with 25%≤x≤40% (mass fraction): (a) B25, 900 °C; (b) B30, 870 °C; (c) B35, 850 °C; (d) B40, 830 °C
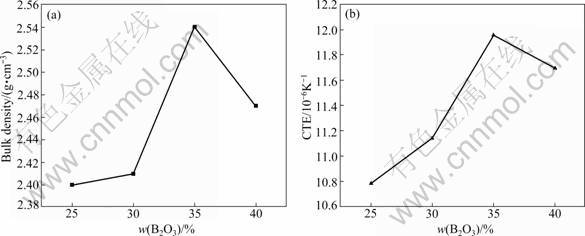
Fig.4 Sintering and thermal properties of CaO-xB2O3-SiO2 glass ceramics with 25%≤x≤40% (mass fraction): (a) Bulk density; (b) Coefficient of thermal expansion
The coefficient of thermal expansion (CTE) of CBS glass ceramics is also shown in Fig.4. The behavior of CTE is similar to that of the bulk density, indicating that microstructure is an important factor influencing the CTE of the samples. The maximum thermal expansion coefficient is observed on the B35 sample, and the value is 11.95×10-6 °C-1. A further addition of B2O3 over 35% leads to a decrease in the CTE of the B40 sample. However, it is still higher than that of 30% B2O3. In the previous discussion, [BO4] can prevent the glass phases from converting to the crystalline phases. It can be observed in Fig.3 that the crystalline phases of the B25 and B30 samples are more than those of the B35 and B40 samples. A large amount of glass phases in the B40 sample can be found. The increasing amplitude of vibration of atoms with increasing temperature causes thermal expansion. This increased amplitude generally causes an increase in the average interatomic distance, and then it increases the length or volume of a sample. The coefficient of linear thermal expansion is defined as the ratio of the change in length per unit length and per unit temperature [20]. In Fig.3, it can be realized that the structure of the B35 sample is more compact than other samples. So, the B35 sample has the highest thermal expansion coefficient. In the same system, the imporosity crystalline phases have higher thermal expansion coefficients than the porosity glass phases. The excessive [BO4] causes more glass phases. When the content of B2O3 is more than 35%, a large number of glass phases lead to a less thermal expansion coefficient.
Figure 5 summarizes the εr and tan δ of the sintered CBS samples with different B2O3 contents. The values of εr and tan δ vary greatly as the amount of B2O3 content increases from 25% to 40%. Among these glass ceramics, the B25 sample has the lowest dielectric constant (εr= 6.18), and its dielectric loss is the highest (tan δ=0.034). The B35 sample has the highest dielectric constant (εr= 6.42), and its dielectric loss is the lowest (tan δ=0.000 9). The εr value of the well-sintered CBS samples are mainly influences by the major crystalline phases (CaSiO3, CaB2O4 and SiO2) and glass phases, whose εr values are about 5.4, 7, 3.8 and 7, respectively [4]. In the previous discussion, it could be known that CaB2O4 and α-SiO2 crystalline phases increase with the increase of B2O3 content, while CaSiO3 changes in a contrary tendency. The εr value of CBS samples rises firstly and reaches its maximum on 6.42 (the B35 sample), then drops to 6.28. The increasing of α-SiO2 phases hampers the electronic transfer rate, and it gives rise to the decrease of dielectric loss. At the same time, the bulk density is closely related to the dielectric loss. Therefore, the samples with the highest bulk density have the lowest dielectric loss. For example, the B35 sample with 2.54 g/cm3 has dielectric loss of 0.000 9. Furthermore, in glass ceramics systems, glass phases as the main reason result in the increase of dielectric loss [4]. So, the tan δ value of CBS samples presents to drop first and reaches the minimum of 0.000 9 (the B35 sample), then rises to 0.014.
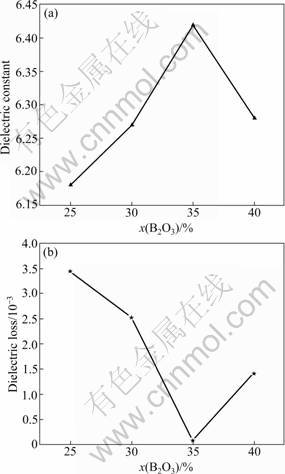
Fig.5 Dielectric properties of CaO-xB2O3-SiO2 glass ceramics with 25≤x≤40 (mass fraction, %): (a) Dielectric constant; (b) ielectric loss
4 Conclusions
1) The most obvious change at about 700, 1 080 and 1 440 cm-1 on Fourier transform infrared spectra of CaO- Ba2O3-SiO2 is that the vibrating bands gradually become stronger. The amount of [BO3] and [BO4] increases with the increase of B2O3 content. At the same time, the [SiO4] vibrating bands of the B40 sample at about 460 cm-1 gradually become stronger.
2) CaB2O4 crystalline phases increase with increasing the B2O3 content of the samples. Without enough Ca ions, CaSiO3 crystalline phases decrease and α-SiO2 crystalline phases increase for the CaO-xB2O3- SiO2 glass ceramics.
3) As the amount of B2O3 increases, the bulk density, CTE and dielectric constant of the samples increase to a maximum and then decrease thereafter. A bulk density of 2.54 g/cm3, a CTE value of 11.95×10-6 °C-1 (20-500 °C), a εr value of 6.42 and a tan δ value of 0.000 9 (measured at 9.7 GHz) are obtained for 35% B2O3 (mass fraction) of CBS glass ceramics sintered at 850 °C for 15 min.
References
[1] SEBASTIAN M T, JANTUNEN H. Low loss dielectric materials for LTCC applications: A review [J]. International Materials Reviews, 2008, 53(2): 57-90.
[2] ZHU Hai-kui, LIU Min, ZHOU Hong-qing, LI Li-quan, L? An-guo. Study on properties of CaO-B2O3-SiO2 system glass-ceramic [J]. Materials Research Bulletin, 2007, 42(6): 1137-1144.
[3] ZHU Hai-kui, ZHOU Hong-qing, LIU Min, WEI Peng-fei, NING Ge. Low temperature sintering and properties of CaO-B2O3-SiO2 system glass ceramics for LTCC applications [J]. Journal of Alloys and Compounds, 2009, 482(1/2): 272-275.
[4] ZHU Hai-kui, ZHOU Hong-qing, LIU Min, WEI Peng-fei, XU Gui-jun, NING Ge. Microstructure and microwave dielectric characteristics of CaO-B2O3-SiO2 glass ceramics [J]. Journal of Material Science, 2009, 20(11): 1135-1139.
[5] ZENG Qun, LI Wei, SHI Jian-lin, GUO Jing-kun, CHEN Heng, LIU Ming-lin. Effect of B2O3 on the sintering and microwave dielectric properties of M-phase LiNb0.6Ti0.5O3 ceramics [J]. Journal of the European Ceramic Society, 2007, 27(1): 261-265.
[6] LIM E S, KIM B S, LEE J H, KIM J J. Characterization of the low temperature firing BaO-B2O3-SiO2 glass: The effect of BaO content [J]. Journal of the European Ceramic Society, 2007, 27(2/3): 825- 829.
[7] HUANG Cheng-liang, WANG Jun-jie, LI Bing-jing, LEE Wen-chang. Effect of B2O3 additives on sintering and microwave dielectric behaviors of 0.66Ca(Mg1/3Nb2/3)O3-0.34CaTiO3 ceramics [J]. Journal of Alloys and Compound, 2008, 461(1/2): 440-446.
[8] YUKSEL B, KIRTAY S, OZKAN O, ACIKALIN E, ERKALFA H. The effect of B2O3 addition to microstructure and magnetic properties of Ni0.4Zn0.6Fe2O4 ferrite [J]. Journal of Magnetism and Magnetic Materials, 2008, 320(5): 714-718.
[9] LOROSCH J, COUZI M, PELOVZ J. Brillouin and raman scattering study of borate glasses [J]. Journal of Non-crystalline Solids, 1984, 69(1): 1-25.
[10] WANG H P, XU S Q, LU S Q, ZHAO S L, WANG B L. Dielectric properties and microstructure of CaSiO3, ceramics with B2O3 addition [J]. Ceram Int, 2009, 35(7): 2715-2718.
[11] RADA S, CULEA M, NEUMANN M. Structural role of europium ions in lead–borate glasses inferred from spectroscopic and DFT studies [J]. Chemical Physics Letters, 2008, 460(1/2/3): 196-199.
[12] KLOSS T. Advances in the process of floating borosilicate glasses and some recent applications for specialty borosilicate float glasses [J]. Glass Technology, 2000, 41(6): 177-181.
[13] BOCCACCINI A R, ACEVEDO D, DERICIOGLU A F. Processing and characterization of model optimum mechanical composites in the system sapphire fibre/borosilicate glass matrix [J]. Journal of Materials Processing Technology, 2005, 169(2): 270-280.
[14] KASHIF I, SOLIMAN A A, FAROUK H. Effect of copper addition on density and magnetic susceptibility of lithium borate glasses [J]. Physical B, 2008, 403(21/22): 3903-3906.
[15] RADA S, CULEA M, CULEA E. Structure of TeO2·B2O3 glasses inferred from infrared spectroscopy and DFT calculations [J]. Journal of Non-crystalline Solids, 2008, 354(52/53/54): 5491-5495.
[16] EGILI K E. Infrared studies of Na2O-B2O3-SiO2 and Al2O3-Na2O- B2O3-SiO2 glasses [J]. Physical B, 2003, 325: 340-348.
[17] LIU Shu-jiang, LU An-xian, TANG Xiao-dong, HE Shao-bo. Influence of addition of B2O3 on properties of Yb3+-doped phosphate laser glass [J]. Journal of Central South University of Technology, 2006, 13(5): 468-472.
[18] KIM M H, NAHM S, LEE W S. Effect of B2O3 and CuO on the sintering temperature and microwave dielectric properties of Ba(Zn1/3Ta2/3)O3 ceramics [J]. Japanese Journal of Applied Physics, 2005, 44(5A): 3091-3094.
[19] KIM M H, JEONG Y H, NAHM, S. Effect of B2O3 and CuO additives on the sintering temperature and microwave dielectric properties of Ba(Zn1/3Nb2/3)O3 ceramics [J]. Journal of the European Ceramic Society, 2006, 26(10/11): 2139-2142.
[20] GUAN Zeng-duo, ZHANG Zhong-tai, JIAO Jin-sheng. Physical properties of inorganic materials [M]. Beijing: Tsinghua University Press, 2000: 119-346. (in Chinese)
(Edited by HE Yun-bin)
Foundation item: Project(2007AA03Z0455) supported by the National High-Technology Research and Development Program of China; Project(BE2009168) supported by the Natural Science Foundation of Jiangsu Province in China; Project supported by the Priority Academic Program Development of Jiangsu Higher Education Institution, China
Received date: 2010-07-15; Accepted date: 2010-10-08
Corresponding author: ZHOU Hong-qing, Professor; Tel: +86-25-86639976; E-mail: hqzhou323@yahoo.cn