
Microstructure and mechanical properties of TiN/TiAlN multilayer coatings deposited by arc ion plating with separate targets
WEI Yong-qiang, LI Chun-wei, GONG Chun-zhi, TIAN Xiu-bo, YANG Shi-qin
State Key Laboratory of Advanced Welding and Joining,
Harbin Institute of Technology, Harbin 150001, China
Received 9 August 2010; accepted 14 January 2011
Abstract: TiN/TiAlN multilayer coatings were prepared by arc ion plating with separate targets. In order to decrease the unfavorable macroparticles, a straight magnetized filter was used for the low melting aluminium target. The results show that the output plasmas of titanium target without filter and aluminium target with filter reach the substrate with the same order of magnitude. Meanwhile, the number of macroparticles in TiN/TiAlN multilayer coatings deposited with separate targets is only 1/10-1/3 of that deposited with alloy target reported in literature. Al atom addition may lead to the decrease of peak at (200) lattice plane and strengthening of peak at (111) and (220) lattice planes. The measured hardness of TiN/TiAlN multilayer coatings accords with the mixture principle and the maximum hardness is HV2495. The adhesion strength reaches 75 N.
Key words: arc ion plating; TiN/TiAlN coatings; M2 high speed steel; macroparticles; hardness; bilayer modulation
1 Introduction
TiAlN coatings are used in dry cutting operations because of their high oxidation temperature (800- 900 °C)[1-2] and good wear resistance[3]. To further improve the hardness and the oxidation resistance, multilayer coatings with layer dimensions on the nano-scale have been utilized. Multilayer coatings have new structural features and improved mechanical properties responding to specific service requirements. PALDEY and DEEVI[4-5] and CHEN et al[6] have investigated the surface properties of single layer, multilayer and gradient (Ti, Al)N coatings. BRAIC et al[7] proposed TiN/TiAlN hard coatings as possible candidate to be applied as protective layer on medical implants or prosthesis. BARSHILIA and RAJAM[8] employed Raman-scattering to study the interface structure of nanolayered TiAlN/TiN grown by reactive DC magnetron sputtering. They found that the interfacial diffusion led to the low hardness of TiAlN/TiN multilayer coatings. WU et al[9] found that the TiN/TiAlN multilayer coating have better properties than TiAlN monolayer coating. Most of these researches employed TiAl alloy targets to deposit TiAlN coating. This may increase the cost of tests and cause the difficulties to adjust the composition of coatings[10]. Therefore, it is interesting to fabricate TiAlN by separate pure metal targets.
KNOTEK et al[11] demonstrated that aluminum target was nitrided by an increasing nitrogen process pressure, resulting in a higher melting point and thus reducing macroparticles emission. WUHRER et al[12-13] employed magnetron sputtering with individual pure titanium and aluminium targets. They reported the effects of nitrogen partial pressure and aluminium content on coating, and target-substrate distance on microstructure, surface morphology and properties of TiAlN coatings. The low melting point targets inclined to the emission of more macroparticles. In order to reduce the volume fraction of macroparticles within the coatings, many filter approaches[14-15] were invented to separate and remove macroparticles from the cathodic arc plasma.
In this work, the TiN/TiAlN multilayer coatings were deposited on M2 high speed steel(HSS) by arc ion plating. In order to substitute separate pure metal targets for TiAl alloy target and reduce the macroparticles from Al target of low melting point, the straight magnetized filter was used. The effects of bilayer modulation on characteristics of TiN/TiAlN multilayer coatings were studied, including phase composition, preferred orientation, surface morphology and mechanical properties.
2 Experimental
TiN/TiAlN multilayer coatings were deposited on M2 HSS (0.93% C, 6.38% W, 5.25% Mo, 4.22% Cr, 1.92% V, Fe balance) substrates with 30 mm in diameter and 4 mm in thickness. The samples were grounded with a series of emery papers of 600 grit, 800 grit and finally polished to mirror-like with 1 μm-diamond powder. Then the samples were ultrasonically cleaned with acetone and ethanol in an ultrasonic bath for 10 min, respectively, blow dried and stored in a desiccator prior to the coating operation. Distances between the substrate and the Ti and Al targets were 200 mm and 420 mm, respectively.
The schematic diagram of the arc ion plating apparatus used to deposit TiN/TiAlN multilayer coatings is shown in Fig.1. Those substrates were placed on a rotary sample holder. Targets were pure Ti (99.9% in molar fraction) and pure Al (99.9% in molar fraction), from which titanium and aluminum plasmas were generated respectively. For the Al target, the unwanted negative macroparticles were decreased by a rectilinear magnetic filter duct. After the chamber was evacuated to below 5×10-2 Pa and then high pure Ar gas (99.999% in volume fraction) was introduced into the chamber to a pressure of 1.0 Pa with flow speed of 5 mL/min. Those substrates were pre-treated by Ar glow discharge with bias of -750 V. After those samples were cleaned by Ar ion sputtering, high pure N2 gas (99.999% in volume fraction) was added as a reactive gas to a pressure of 1.2 Pa. TiN monolayer was deposited as interlayer. Then TiN and TiAlN were deposited alternately on substrates by rotating the holder with the rotation speed of 30 r/min. Ti and Al targets were evaporated by arc-discharge with an arc current of 80 A. Deposition rates were approximately 2 nm/s and 3 nm/s for TiN and TiAlN, respectively. TiN/TiAlN multilayer coatings with different bilayer modulations were deposited simply by varying the arcing time for Al cathode. The bilayer modulation periods were 60, 90, 120 and 150 s. Details about the deposition parameters of multilayer coatings are listed in Table 1.
The microstructure of the multilayer coatings was studied by X-ray diffractometry (XRD) using a Phillips diffractometer operated in Bragg-Brentano configuration with Cu (Kα) radiation. Scanning electron microscopy (SEM) was used to analyze the coatings morphology and thickness. Micro-hardness tests were also carried out in Shimadzu Vickers micro-hardness DUH-W201S-E tester with a load of 800 mN. The adhesion of the TiN/TiAlN multilayer coatings was investigated with an automatic scratch-tester at MFT-4000 equipped with the diamond indenter with a tip of 120° in cone angle and 0.2 mm in radius. The normal force of the indenter onto the sample surface was increased continuously from 0 to 80 N with the loading speed of 50 N/min and the track length was 4 mm.
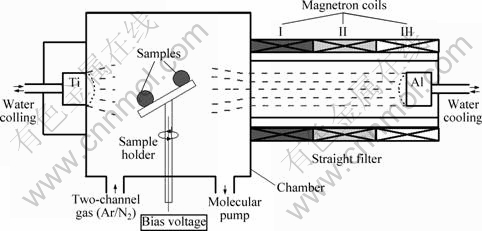
Fig.1 Schematic diagram of arc ion plating apparatus
Table 1 Parameters for deposition of TiN/TiAlN multilayer coatings
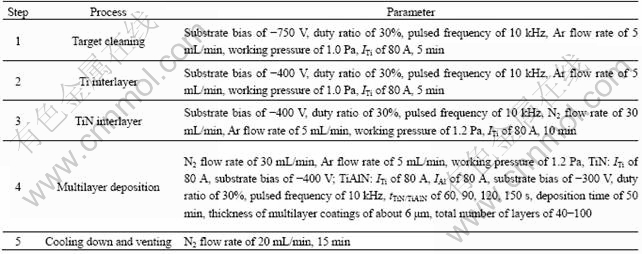
3 Results and discussion
3.1 Substrate average current
As shown in Fig.2, the sample holder kept stationary. Those substrates (1#, 2#, 3#, 4#) were insulated each other and -50 V DC bias was applied to them, which were 201 stainless steel with dimensions of 50 mm×50 mm×0.7 mm. Ar gas was introduced into the chamber to a pressure of 1.0 Pa with flow speed of 10 mL/min. An arc current of 70-90 A was used. As shown in Fig.3, at 1# position the maximum average current can reach 8 mA for Al target, but about 4 mA for Ti target. It may be caused by the focus of magnetic field, leading to that the Al plasma came out from the straight pipe and was more concentrated. Another reason may be that at the same target current, Al target can produce more plasma than Ti target. Although the distance between Al target and substrates was further than that between Ti target and substrates, the Al plasma remained to be more than the Ti plasma. These results show the feasibility of arc ion plating with separate targets and straight magnetized filter. In practical applications, the several sources could be utilized to improve efficiency and optimize the mole ratio of Al to Ti in multilayer coatings.
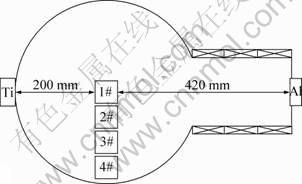
Fig.2 Schematic diagram for substrate current measurement
3.2 Phase composition
Figure 4 presents the XRD patterns of TiN/TiAlN multilayer coatings. The structure of multilayer coatings strongly changed with the increase of bilayer modulation from 60 s to 150 s. All investigated coatings were highly textured with a preferential orientation in the (111) and (220) direction with the corresponding 2θ of about 36.5° and 61.4°, respectively. The intensities of other diffraction peaks such as (200), (311) and (222) were very low, which were often observed in the PVD processes[16-17]. The lattice parameter of AlN is 0.412 nm and the lattice parameter of TiN is 0.424 nm. So the titanium atoms of TiN lattice were replaced by aluminum atoms to cause the lattice distortion and the internal stress change in TiAlN, which leads to the XRD peaks of TiN/TiAlN multilayer coatings overall shift to the right[18]. The peak width of textured grains in the growth direction (111) was very narrow, indicating a high degree of crystallinity[19]. XRD peaks of (111) and (220) have different relative intensities for the multilayer coatings with different bilayer modulation. The difference in the relative intensity was indicative of a variation of the oriented grains in number. Modulation structure can affect not only the growth rate but also the preferred orientation of epilayers[20]. The XRD peak for (220) broadened and shifted to higher 2θ values with the increase of bilayer modulation. By further comparison of diffraction patterns, it is recognized that the intensity ratios of the reflections of cubic phase were influenced by bilayer modulation.
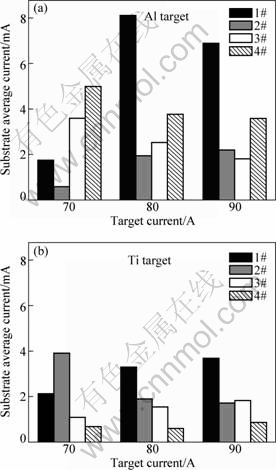
Fig.3 Substrate average current at different positions: (a) Al target; (b) Ti target
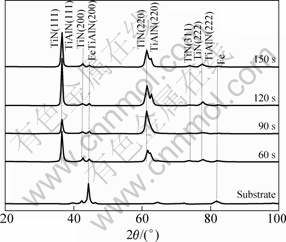
Fig.4 XRD patterns for TiN/TiAlN coatings with different bilayer periods
3.3 Surface morphology and number of macroparticles
Figure 5 shows SEM images of TiN/TiAlN coatings with different bilayer modulations. The surface of coatings was dense even with some macroparticles, which was a unique feature of arc ion plating. The number distribution of macroparticles with different sizes was counted and analyzed. As shown in Fig.6, the number of different size macroparticles increased with bilayer modulation. It is attributed to deposited probability of the macroparticles enlarged by using the Al target. With the increase of Al target arcing time, more Al macroparticles reached the substrate. Although the straight magnetized filter could reduce the arrival probability of macroparticles, the Al target with low melting point still increased greatly this probability. While compared with the results from LIU et al[21], the number of macroparticles in coating deposited by arc ion plating with separate targets and straight magnetized filter decreased to 1/10-1/3.
3.4 Cross-section morphology and thickness
Figure 7 presents cross-sectional images of the TiN/TiAlN multilayer coatings with different bilayer modulation periods. The TiN/TiAlN coatings have the thickness of 5.6-6.7 μm, as shown in Fig.8. With the increase of Al target arcing time, more Al plasma reached the surface of substrate. Although the total time was equal, the Al target stable arcing time was lengthened with the increase of bilayer modulation periods. The Al plasma from the straight pipe was more concentrated by magnetic mirror effect with the same target current, and Al target produced more plasma than Ti target. Although the distance between Al target and substrate was further than that between Ti target and substrate, the Al plasma remained to be more than the Ti plasma. Therefore, the thickness of multilayer coatings becomes larger with the increase of bilayer modulation periods.
3.5 Mechanical characteristics
Figure 9 reveals that the adhesion strength increased with the increase of bilayer modulation periods and reached 75 N at 150 s. Figure 10 shows the hardness of TiN/TiAlN multilayer films with different bilayer modulation periods. With the increase of bilayer modulation periods, the hardness reached HV2495, which was 2.7 times that of M2 HSS substrate. In the literature, the hardness value measured for monolayer (Ti, Al)N was 28-32.4 GPa[5] and monolayer TiN was
16-20 GPa[5, 22]. To provide an estimation of the hardness enhancement associated with multilayer effects, the rule of mixture was applied to TiN/TiAlN[23].

where H is the hardness and t is the thickness of the layers. The calculated hardness value was 24-26 GPa, which was 1-3 GPa larger than the measured value.
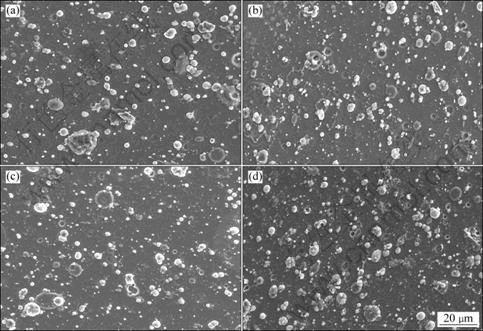
Fig.5 Surface SEM micrographs of TiN/TiAlN coatings with different bilayer modulation periods: (a) 60 s; (b) 90 s; (c) 120 s; (d) 150 s
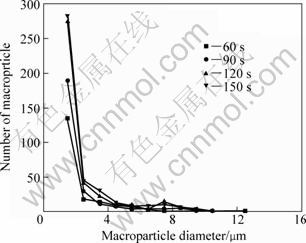
Fig.6 Number of macroparticles in TiN/TiAlN coatings with different bilayer modulation periods
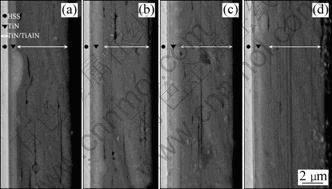
Fig.7 Cross-section SEM micrographs of TiN/TiAlN coatings with different bilayer modulation periods: (a) 60 s; (b) 90 s; (c) 120 s; (d) 150 s
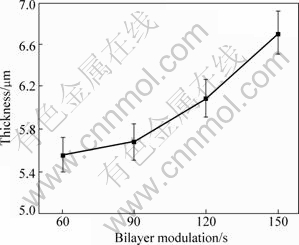
Fig.8 Thickness of TiN/TiAlN coatings with different bilayer modulation periods
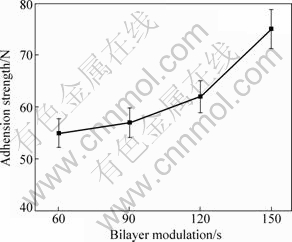
Fig.9 Adhesion strength of TiN/TiAlN coatings with different bilayer modulation periods
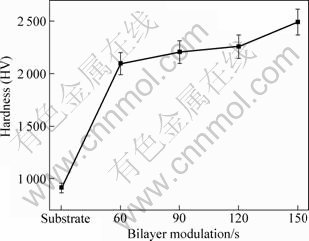
Fig.10 Hardness of TiN/TiAlN coatings with different bilayer modulation periods
4 Conclusions
1) The separate target arc ion plating with a straight magnetized filter may be proper substituent for alloy target to deposit TiAlN coatings.
2) With the increase of bilayer modulation period, the thickness of TiN/TiAlN multilayer coatings increases and the number of macroparticles increases with the increase of Al target arcing time.
3) The value for the critical loads of the TiN/TiAlN multilayer coatings depends on the bilayer modulation period and the adhesion strength reaches 75 N at bilayer modulation period of 150 s.
4) The hardness of TiN/TiAlN multilayer coatings increases with the increase of the coating thickness. The maximum hardness reaches HV2495, which was 2.7 times that of M2 HSS substrate.
References
[1] RU Q, HU S. Effects of Ti0.5Al0.5N coatings on the protecting against oxidation for titanium alloys [J]. Rare Metals, 2010, 29(2): 154-161.
[2] LIU Ai-dong, YANG Shi-wei. High temperature oxidation behavior of (Ti, Al)N coating deposited by arc ion plating [J]. Transactions of Nonferrous Metals Society of China, 2005, 15(S3): 398-401.
[3] ZHENG L Y, ZHAO L X, XIONG W H. Tribological properties of TiAlN-coated cermets [J]. Rare Metals, 2009(1): 57-62.
[4] PALDEY S, DEEVI S C. Properties of single layer and gradient (Ti,Al)N coatings [J]. Materials Science and Engineering A, 2003, 361(1-2): 1-8.
[5] PALDEY S, DEEVI S C. Single layer and multilayer wear resistant coatings of (Ti,Al)N: A review [J]. Materials Science and Engineering A, 2003, 342(1-2): 58-79.
[6] CHEN L, DU Y, YIN F, LI J. Mechanical properties of (Ti, Al)N monolayer and TiN/(Ti, Al)N multilayer coatings [J]. International Journal of Refractory Metals and Hard Materials, 2007, 25(1): 72-76.
[7] BRAIC M, BALACEANU M, BRAIC V, VLADESCU A, PAVELESCU G, ALBULESCU M. Synthesis and characterization of TiN, TiAIN and TiN/TiAIN biocompatible coatings [J]. Surface and Coatings Technology, 2005, 200(1-4): 1014-1017.
[8] BARSHILIA H C, RAJAM K S. A Raman-scattering study on the interface structure of nanolayered TiAIN/TiN and TiN/NbN multilayer thin films grown by reactive dc magnetron sputtering [J]. Journal of Applied Physics, 2005, 98(1): 014311-014319.
[9] WU En-xi, CHEN Li, YIN Fei, WANG Xiu-quan. Microstructure and physical properties of PVD TiN/(Ti, Al)N multilayer coatings [J]. Transactions of Nonferrous Metals Society of China, 2005, 15(5): 1072-1076.
[10] ZHANG J. Composition control of alloy coatings and composition design of cathode targets in multi-arc ion plating [J]. Journal of Materials Science and Technology, 2000, 16(3): 348-350.
[11] KNOTEK O, L FFLER F, SCHOLL H J, BARIMANI C. The multisource arc process for depositing ternary Cr- and Ti-based coatings [J]. Surface and Coatings Technology, 1994, 68-69: 309-313.
[12] WUHRER R, KIM S, YEUNG W Y. Effect of nitrogen partial pressure on the surface morphology and properties of reactive dc magnetron sputtered (Ti, Al)N coatings [J]. Scripta Materialia, 1997, 37(8): 1163-1169.
[13] WUHRER R, YEUNG W Y. Effect of target-substrate working distance on magnetron sputter deposition of nanostructured titanium aluminium nitride coatings [J]. Scripta Materialia, 2003, 49(3): 199-205.
[14] ANDERS A. Approaches to rid cathodic arc plasmas of macro- and nanoparticles: A review [J]. Surface and Coatings Technology, 1999, 120-121: 319-330.
[15] LI Cheng-ming, ZHANG Yong, CAO Er-yan, XUE Ming-lun. Deposition and properties of high quality (TiAl)N hard coatings [J]. Transactions of Nonferrous Metals Society of China, 2001, 11(S1): 179-182. (in Chinese)
[16] GREDIC T, ZLATANOVIC M. Plasma deposition of (Ti,Al)N coatings at various magnetron discharge power levels [J]. Surface and Coatings Technology, 1991, 48(1): 25-30.
[17] KNOTEK O, LEYENDECKER T. On the structure of (Ti, Al)N-PVD coatings [J]. Journal of Solid State Chemistry, 1987, 70(2): 318-322.
[18] HORLING A, HULTMAN L, ODEN M, SJOLEN J, KARLSSON L. Thermal stability of arc evaporated high aluminum-content Ti1-xAlxN thin films [J]. Journal of Vacuum Science and Technology A, 2002, 20(5): 1815-1823.
[19] MENDIBIDE C, STEYER P, FONTAINE J, GOUDEAU P. Improvement of the tribological behaviour of PVD nanostratified TiN/CrN coatings—An explanation [J]. Surface and Coatings Technology, 2006, 201(7): 4119-4124.
[20] XU X M, WANG J, AN J, ZHAO Y, ZHANG Q Y. Effect of modulation structure on the growth behavior and mechanical properties of TiN/ZrN multilayers [J]. Surface and Coatings Technology, 2007, 201(9-11): 5582-5586.
[21] LIU X B, JOHNSON C, LI C M, XU J, CROSS C. Developing TiAlN coatings for intermediate temperature solid oxide fuel cell interconnect applications [J]. International Journal of Hydrogen Energy, 2008, 33(1): 189-196.
[22] RZEPIEJEWSKA-MALYSKA K, PARLINSKA-WOJTAN M, WASMER K, HEJDUK K, MICHLER J. In-situ SEM indentation studies of the deformation mechanisms in TiN, CrN and TiN/CrN [J]. Micron, 2009, 40(1): 22-27.
[23] SANTANA A E, KARIMI A, DERFLINGER V H, SCHUTZE A. Microstructure and mechanical behavior of TiAlCrN multilayer thin films [J]. Surface and Coatings Technology, 2004, 177-178: 334-340.
分离靶电弧离子镀制备TiN/TiAlN多层薄膜的
微观结构和力学性能
魏永强,李春伟,巩春志,田修波,杨士勤
哈尔滨工业大学 先进焊接与连接国家重点实验室,哈尔滨 150001
摘 要:采用分离靶电弧离子镀制备TiN/TiAlN多层薄膜。为了减少大颗粒的不利影响,利用直线型磁过滤方法来减少低熔点铝靶产生的大颗粒。结果表明,没有过滤的钛靶和磁过滤的铝靶等离子体到达基体的输出量在相同的数量级,同时,采用该方法制备的薄膜中的大颗粒数目是文献中报道的合金靶制备的薄膜大颗粒数目的1/10~1/3。Al元素的添加引起薄膜在(200)晶面的峰值降低,而在(111)和(220)晶面的峰值增强。TiN/TiAlN多层薄膜的最大硬度为HV2495,薄膜的硬度增强符合混合法则,结合力达75 N。
关键词:电弧离子镀;TiN/TiAlN薄膜;M2高速钢;大颗粒;硬度;双层调制
(Edited by YANG Hua)
Foundation item: Projects (50773015, 10775036) supported by the National Natural Science Foundation of China
Corresponding author: TIAN Xiu-bo; Tel: +86-451-86418784; E-mail: xiubotian@163.com
DOI: 10.1016/S1003-6326(11)60823-6