Article ID: 1003-6326(2005)03-0594-06
Reduction of TiO2 with hydrogen cold plasma in
DC pulsed glow discharge
ZHANG Yu-wen(张玉文), DING Wei-zhong(丁伟中), LU Xiong-gang(鲁雄刚),
GUO Shu-qiang(郭曙强), XU Kuang-di(徐匡迪)
(Shanghai Key Laboratory of Modern Metallurgy and Material Processing,
Shanghai University, Shanghai 200072, China)
Abstract: The reduction of TiO2 to Ti2O3 with hydrogen cold plasma generated by a DC pulsed glow discharge was realized under 2500Pa at 1233K. Only a little of Ti10O19 and Ti9O17 was detected for using molecular hydrogen. Enhancement effects of hydrogen cold plasma on the reduction were discussed in terms of thermodynamic coupling, kinetics and plasma sheath. The exited hydrogen species are considered more effective reducing agents. It is instructive to reduce refractory oxides with plasma hydrogen at the reduced temperature.
Key words: hydrogen; cold plasma; reduction; TiO2; pulsed glow discharge CLC
number: TF11; O646.9 Document code: A
1 INTRODUCTION
In extractive metallurgy many metal oxides are reduced with carbon. The carbothermic reduction process produces a great lot of CO2 which is responsible for greenhouse effect. Hydrogen in place of carbon for the reduction of oxides should be a clean reductant[1, 2]. However, if the substitution of hydrogen for carbon is made, the hydrogen reduction should first be enhanced[3]. It is generally accepted that the hydrogen reduction of refractory oxides will not produce their metals unless there is significant atomic hydrogen species in the system[4]. Some researches have been carried out on the reduction of metal oxides in thermal plasma hydrogen[5-13]. In fact, the thermal plasmas are mainly used in those researches as a high-enthalpy source. The effect of active plasma hydrogen is significantly influenced by the plasma type(thermal or cold plasma).
In this study, hydrogen cold plasma was used to reduce metal oxide. Cold plasma is one of the plasma in which the temperature of gas molecular and ions is significantly lower than that of free electrons. The temperature of heavy particles is less than 1300K,whereas the electron temperature reaches around 10000K[14]. This is instructive to reduce refractory oxides with plasma hydrogen at the reduced temperature.
2 FEASIBILITY OF REDUCTION OF METAL OXIDES WITH HYDROGEN COLD PLASMA
The Gibbs free energies for reduction of TiO2 and Cr2O3 with molecular and monatomic hydrogen are plotted in Fig.1. Monatomic hydrogen is one of the active species in the cold plasma. It can be seen from the graph that there is a contrary trend of the free energy variation for different hydrogen species reduction. With the increase of reaction temperature, the Gibbs free energy for molecular hydrogen reduction decreases and its reducibility goes up, whereas that for plasma hydrogen is in an opposite way. As the reaction temperature is higher than several thousands K, the Gibbs free energy for plasma hydrogen reduction becomes positive and the reaction of TiO2 and Cr2O3 can not occur spontaneously. Despite using 100% atomic hydrogen reactant, the calculation result is indicative of trouble for reaction. The background temperature must be well below 4400K the temperature at which water produced by the reduction will be dissociated into hydrogen and oxygen, leading to a back-reaction, as shown in Fig.2. The lifetime of the active hydrogen species should be long enough to react with metal oxides, especially with refractory oxides. The work by Belmonte et al[16] has discussed that the high density of atomic hydrogen can be obtained in Ar-H2 glow discharge, if a surface process(i.e. hydrogen recombination on the reaction walls) is limited by making the wall temperature as low as possible. Indeed, the diffusion of atomic hydrogen to the wall is extremely fast. While the gas temperature is high, the heterogeneous recombination of atomic species occurs rapidly on the wall.
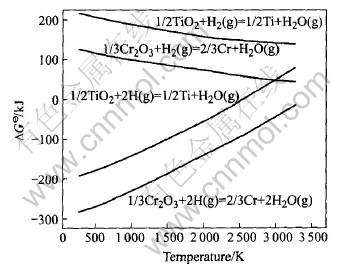
Fig.1
—T curves for reduction of TiO2 and Cr2O3 with atomic hydrogen and molecular hydrogen[15]
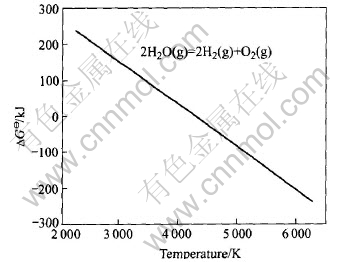
Fig.2 Gibbs free energy variation for dissociation of H2O with temperature[15]
All analyses as above indicate that compared with thermal plasma in traditional extractive metallurgy, a cold plasma with properly low temperature is a more feasible system for oxide reduction. In this paper, the reduction of TiO2 in hydrogen cold plasma produced by DC pulsed glow discharge was studied.
3 EXPERIMENTAL
The experimental apparatus is schematically shown in Fig.3. A pulsed power electrical source was applied to generate and maintain hydrogen cold plasma between the cathode and anode consisting of stainless steel plates. The vertical reaction chamber was made of a fused silica tube. During the experiments, an oxide sample was placed on the nether plate. The hydrogen gas was introduced through a hollow pipe connected to the upper electrode plate. Oxide tablets with a diameter of 11mm and a thickness of 2mm were prepared from pure TiO2 powder after pressing and sintering. At the beginning, the reaction chamber was first evacuated by a vacuum pump and then flushed with high-purity-grade hydrogen. A power supply to the heater and a gas flow were adjusted to the desired temperature and pressure. DC power was turned on and cold plasma was ignited. The temperature was checked by a thermocouple underneath the sample.
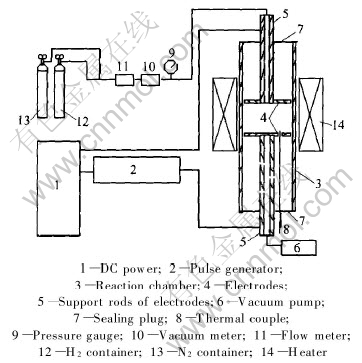
Fig.3 Schematic diagram of experimental apparatus
Upon completion of an experiment, the plasma was extinguished and the specimen was allowed to cool in the chamber under a continuous flow of nitrogen. The specimen was analyzed by a X-ray diffractometer(Rigaku D/max-rC, Cu-K) to determine what crystalline products were formed during an experiment. A scanning electron microscope and an optical microscope were used to detect the configuration of specimen surface and its cross section.
In these experiments, the space between the two plates was 10mm and a pulse on/off time ratio was 300μs/500μs. To compare the reactivity of plasma hydrogen with molecular hydrogen, TiO2 tablets were reduced by molecular hydrogen with a heater placed around the same reaction chamber.
4 RESULTS AND DISCUSSION
4.1 X-ray diffraction analysis
Fig.4 shows X-ray diffraction patterns for the raw and reduced samples. The patterns illustrate that under the condition of a pressure of 2500Pa and a temperature of 1233K, after 60min, TiO2 is reduced with plasma hydrogen to Ti2O3, Ti3O5 and a small quantity of Ti9O17. A few of Ti10O19 and Ti9O17 are detected and the main phase is TiO2 after molecular hydrogen reduction. The input power for plasma hydrogen is 300W.This indicates that TiO2 reacts rapidly and intensely with plasma hydrogen compared with molecular hydrogen. The plasma hydrogen is obviously more reactive than molecular hydrogen.
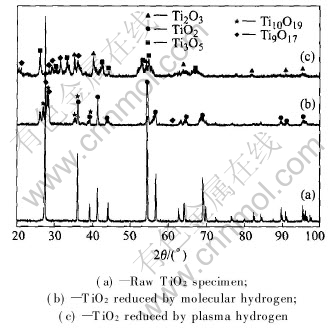
Fig.4 X-ray diffraction patterns for different TiO2 specimens
Visual examination of different TiO2 specimens is shown in Fig.5. The raw TiO2 specimen is white. With molecular hydrogen reduction, the specimen becomes light gray, and X-ray diffraction analysis of the gray material reveals the presence of TiO2 and a slight amount of Ti10O19 and Ti9O17. The intensity of the diffraction peaks for TiO2 decreases with the increase of reaction time, indicating that the molecular hydrogen reduction takes place continuously throughout the experiment. A white layer of TiO2 is observed below the gray layer. The white TiO2 underneath the gray layer is considered an unreduced core.
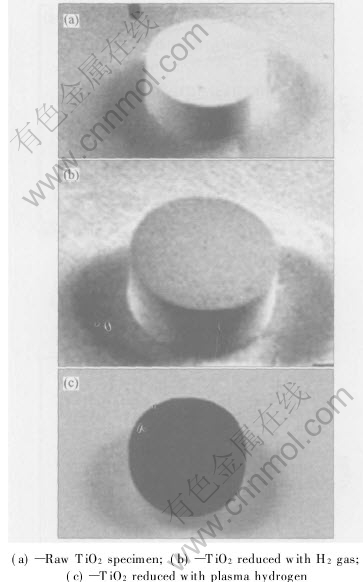
Fig.5 Photos of different TiO2 specimens
When reducing with plasma hydrogen, the specimen looks black, as shown in Fig.5(c). Based on the observation of its cross section by an optical microscopy, it is found that a gray layer and an unreacted layer exist under the black layer, as shown in Fig.6. A black thickness of 1mm is measured for a specimen exposed to hydrogen plasma with 10min and 2mm with 60min. The product layer thickness varies with applied voltage, gas pressure and reaction time. As the reaction interface gradually moves forward from the surface to the inner region, the reaction should obey the shrinking core model. According to a prediction from the related stability diagram[17], the products reduced from TiO2 at 1233K are Ti9O17,Ti3O5 and Ti2O3, respectively.
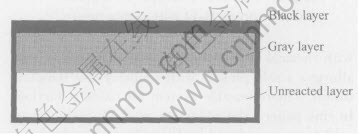
Fig.6 Scheme of reacted specimen
4.2 SEM images
The raw TiO2 particles are non-spherical, as shown in Fig.7(a). With molecular hydrogen reduction, the size of the particles has no change(see Fig.7(b)). As previously mentioned, the surface color changes from white to light gray. The specimen reduced with hydrogen cold plasma takes on black and its main phase is Ti2O3 for 60min, as shown in Fig.7(c). Fig.7(d) gives an evidence of sputtering when the reaction lasts 200min.
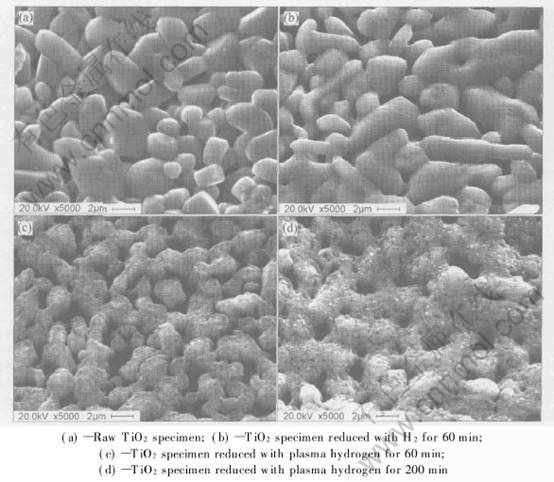
Fig.7 SEM images of different TiO2 specimens
Form the SEM images, the etching effect of hydrogen cold plasma on oxide particles appears distinctively. The oxide smooth surface becomes much rough. The amount of etching spot on the particles depends on the reduction time. After experiments, black deposits are found on the electrodes and the reaction chamber wall. The deposits are considered being Ti2O3 and Ti3O5 by sputtering during the reduction.
5 CONSIDERATIONS OF PLASMA ENHANCEMENT EFFECT
5.1 Thermodynamic coupling
Thermodynamic coupling for the reduction of metal oxides with hydrogen cold plasma can be described as follows:
Reduction with molecular hydrogen:

Activation of molecular hydrogen:

Thermodynamic coupling(1)-(2):

For reaction (2), high-energy electrons(e*) produced in the plasma push the reaction, H2(g)+e*=2H(g)+e, to the right, forming active atomic hydrogen and an electron with reduced energy(e).This kind of dissociation for molecular hydrogen by high-energy electrons is much more effective and advantageous than thermal dissociation[18]. Through thermodynamic coupling, the Gibbs free energy for the reduction of metal oxides with hydrogen becomes negative from positive or further negative even at low temperature. Since the system temperature is determined by molecular and ions temperature, the reduction of oxide will take place at low temperature.
5.2 Importance of plasma sheath
In principle of plasma chemistry, the plasma sheath plays an important role for reaction. The plasma sheath, as shown in Fig.8, forms around a specimen in contact with plasma. In this figure, H+ and e denote an ionic species and an electron, respectively. This sheath develops as a result of the difference in mobility of the free electrons and ions species in the plasma[19].
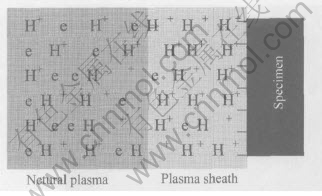
Fig.8 Schematic diagram of plasma sheath in plasma hydrogen
With different charge-to-mass ratio, mobility is various. As a result, free electrons impact the specimen surface more frequently than ions and neutrals. Eventually, the specimen takes on negative charge. By coulombic force, positive ions are attracted and accelerated, while the velocity of followed free electrons are decreased. The accelerated ions impact the specimen surface, breaking the bonds of Me—O to allow the reduction proceeding.
For a discharge under the condition of high gas pressure and voltage, the phenomenon of heavy etching and sputtering(see section 4.2 and Fig.7) also indicates that active hydrogen species with high energy and specimen interact strongly on each other. The high-energy hydrogen species impact the specimen surface and transfer their energy to the surface. As a result, there are more active species arriving at the surface. This is very important for the generation of new phase nucleus. The particles with over activation energy increase. All these effects offer improved thermodynamics and kinetics over the conventional hydrogen reduction.
A schematic diagram of activation energy for reduction of oxides with different hydrogen species is plotted in Fig.9. The reaction,H2+MeO=Me+H2O, may proceed at high temperature. But the high activation energy actually presents the reduction occurring. Hydrogen should be excited to active states(atoms or ions) with high energy. The energy of excited species is high. The activation energy(E2 and E3) of reactions with the active hydrogen species are lower than the one(E1) with hot molecular hydrogen. When the active species are of much higher energy, the activation energy(EA) may be zero or negative. A* is present of the hydrogen species with higher energy. So, the excitement of hydrogen is necessary for oxide reduction. This means that the activation energy of hydrogen reduction for active species is much smaller than that for molecular hydrogen.
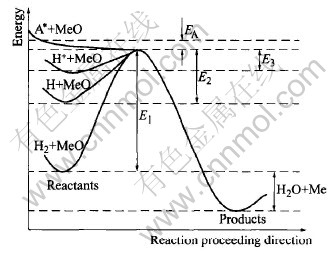
Fig.9 Schematic diagram of activation energy for reduction of oxide with different hydrogen species
6 REDUCIBILITY OF DIFFERENT HYDROGEN SPECIES
The reported TiO2 reductions with different hydrogen species under condition of normal atmosphere and hot plasma are illustrated in Fig.10. The ratio of O to Ti of the final reduction products is plotted as a function of temperature. The solid marks represent the reduction with molecular hydrogen, and the hollow ones are with plasma hydrogen. The molar ratio of O to Ti decreases with the increase of temperature if hydrogen gas is used as a reducing agent. Though the same product, Ti2O3, has been detected after reduction with either hot or cold plasma, the reaction temperature with hot plasma is much higher. So, the oxide reduction with cold plasma hydrogen is very effective.
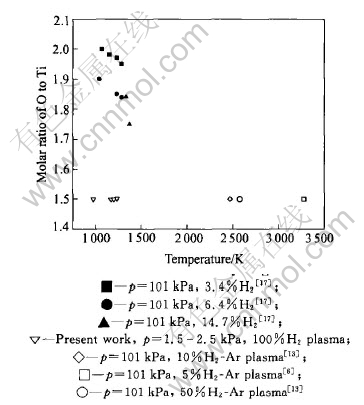
Fig.10 Comparison of molar ratio of O to Ti obtained products reduced with plasma hydrogen and hot molecular hydrogen
7 CONCLUSIONS
Hydrogen gas can be chemically activated by DC glow discharge to reduce refractory oxide TiO2. The experiment results show that the reduction products are Ti2O3, Ti3O5 and a small quantity of Ti9O17 under the condition of hydrogen cold plasma with 2500Pa and 1233K. However, only a little of Ti10O19 and Ti9O17 is detected and the dominant oxide is unreduced TiO2 with molecular hydrogen. The enhancement mechanism is thought to be an effect of active hydrogen species. Oxide reduction with active hydrogen species needs smaller activation energy. The acceleration of hydrogen ions across the plasma sheath around a specimen makes the reduction effective.
REFERENCES
[1]Birat J P, Vizioz J P. CO2 emissions and the steel industrys available responses to the greenhouse effect [J]. La Revue de Metallurgie, 1999(10): 1203-1215.
[2]XU Kuang-di,JIANG Guo-chang, XU Jian-lun, et al. Theoretic analysis on iron and steel processing in 21st century [A]. Xiangshan Science Meeting Center 125th Science Proceeding in Beijing [C]. Beijing: Xiangshan Science Meeting Center, 1999. 31-34.(in Chinese)
[3]ZHANG Yu-wen, DING Wei-zhong, GUO Shu-qiang, et al. Reduction of metal oxide in nonequilibrium hydrogen plasma [J]. The Chinese Journal of Nonferrous Metals, 2004, 14(2): 315-321.(in chinese)
[4]Robine C V. Representation of mixed reactive gases on free energy(ellingham-richardson) diagrams [J]. Metall Mater Trans B, 1996, 27B(2): 65-69.
[5]Dembovsky V. Steel refining by chemically active plasma [J].Journal of Materials Processing Technology, 1998, 78(1): 34-42.
[6]Kitamura T, Shibata K, Takeda K. In-flight reduction of Fe2O3, Cr2O3, TiO2 and Al2O3 by Ar-H2 and Ar-CH2 plasma [J]. ISIJ International, 1993, 33(11): 1150-1158.
[7]Alemany C, Trassy C, Pateyron B, et al. Refining of metallurgical-grade silicon by inductive plasma [J]. Solar Energy Materials and Solar Cells, 2002, 72(1): 41-48.
[8]Watanabe T, Soyama M, Kanzawa A, et al. Reduction and separation of silica-alumina mixture with argon-hydrogen thermal plasmas [J]. Thin Solid Film, 1999, 345(1): 161-166.
[9]Mohai I,Szépv lgyi J, Károly Z, et al. Reduction of metallurgical wastes in an RF thermal plasma reactor [J]. Plasma Chemistry and Plasma Processing, 2001, 21(4): 547-563.
[10]Dietmar V, Eberhard S, Herbert W. Experiments on the smelting reduction of oxides of iron,chromium and vanadium and their mixtures with argon/methane-plasma [J]. Steel Research, 1989, 60(3): 177-181.
[11]Huczko A, Meubus P. Vapor phase reduction of chromic oxide in an Ar-H2 Rf plasma [J]. Metallurgical Transactions B,1988,19(12): 927-933.
[12]Koji K, Nobuyasu K, Iso M, et al. Reduction of molten iron oxide and FeO bearing slags by H2-Ar plasma [J]. Transactions ISIJ, 1984, 24: 7-16.
[13]Palmer R A, Doan T M, Lloyd P G, et al. Reduction of TiO2 with hydrogen plasma [J]. Plasma Chemistry and Plasma Processing, 2002, 22(3): 335-350.
[14]Flamm D L, Manos D M, Flamm D L, et al. Introduction to plasma chemistry [A]. Plasma Etching an Introduction [C].San Diego, CA: Academic Press,1989. 115.
[15]Roine A. Outokumpu HSC Chemistry for Windows: Chemical Reaction and Equilibrium Software with Extensive Thermochemical Database, Pori, Finland: Outokumpu,1999.
[16]Belmonte T, Thiebaut J M, Michel H. Reduction of metallic oxides by late Ar-H2-N2 postdischarge(Ⅰ)—application to copper oxides [J]. J Vac Sci Technol A, 2002, 20(4): 1347-1352.
[17]Bullard D E, Lynch D C. Reduction of titanium dioxide in a nonequilibrium hydrogen plasma [J]. Metall Mater Trans B,1997, 28B(6): 1069-1080.
[18]Hideo S, ZHANG Hai-bo, ZHANG Dan, et al. Electronic Engineering for Plasma [M]. Beijing: Science Pressing(OHM), 2002. 34-36.
[19]Polak L S, Lebedev Y A. Plasma Chemistry [M]. Cambridge: Cambridge International Science Press,1998.
(Edited by LI Xiang-qun)
Foundation item: Project(00JC14013) supported by the Natural Science Foundation of Shanghai, China
Received date: 2004-03-02; Accepted date: 2004-12-20
Correspondence: DING Wei-zhong, Professor, PhD; Tel: +86-21-56331618; E-mail: wzhding@public7.sta.net.cn