
Elimination of phosphorus vaporizing from molten silicon at finite reduced pressure
ZHENG Song-sheng1, 2, Jafar SAFARIAN 2, Seongho SEOK3, Sungwook KIM3,
Tangstad MERETE2, LUO Xue-tao1
1. Department of Materials Science and Engineering, Xiamen University, Xiamen 361005, China;
2. Department of Material Science, Norwegian University of Science and Technology, Trondheim 7034, Norway;
3. Silicon Refining Research Team, Research Institute of Science and Technology, Pohang 790-600, Korea
Received 21 September 2010; accepted 7 November 2010
Abstract: Elimination of phosphorus vaporizing from silicon was investigated. Si-P alloy made from electronic grade silicon was used. All the samples were analyzed by GD-MS. Theory calculation determines that phosphorus evaporates from molten silicon as gas species P and P2 at a finite reduced pressure. The experimental results show that phosphorus mass fraction can be decreased from 0.046% (460ppmw) to around 0.001% (10ppmw) under the condition of temperature 1 873 K, chamber pressure 0.6-0.8 Pa, holding time 1 h. Both experimental data and calculation results agree that at high phosphorus concentration, phosphorus removal is quite dependent on high chamber pressure while it becomes independent on low chamber pressure. The reason is that phosphorus evaporates from molten silicon as gas species P2 at a relatively high phosphorus concentration, while gas species P will be dominated in its vapour at low phosphorus content.
Key words: phosphorus concentration; molten silicon; equilibrium partial pressure; temperature; chamber pressure
1 Introduction
Phosphorus is an impurity in silicon, which is difficult to be removed. For solar cell applications, there are limitations on the level of impurities in semiconductor-grade silicon, but the acceptable levels are higher. The maximum permissible concentrations of individual impurities in solar-grade silicon are defined by studying the conversion efficiency of solar cells as a function of impurity concentration. As shown in Fig.1, the limits on impurity concentrations in p-type silicon for impurities determining the degradation threshold of solar cells were reported by BATHEY and CRETELLA[1], GRIBOV and ZINOV’EV[2] and DIETL[3].
As listed in Table 1, comparison of typical impurity concentrations in metallurgical grade silicon (MG-Si) and solar grade silicon (SoG-Si) has been reviewed by GRIBOV and ZINOV’EV[2], and the corresponding segregation coefficients have been measured by DERA[4], DAVIS et al[5], BATHEY and CRETELLA[1] and SIGMUND[6].
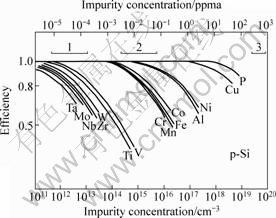
Fig.1 Limits on impurity concentrations in p-type silicon for impurities determining degradation threshold of solar cells: 1—Semiconductor-grade; 2—Solar-grade; 3—Metallurgical- grade[1-2]
Table 1 Impurity content of metallurgical-grade silicon and solar-grade silicon
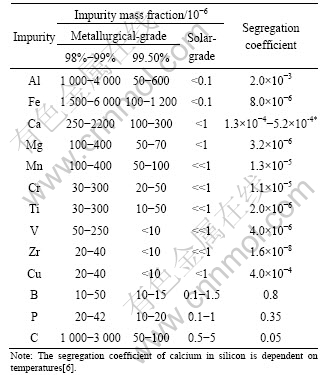
Phosphorus is unavoidably present in the silica and carbon raw materials used for the arc-furnace reduction process, with segregation coefficients equal to 0.35, and very limited phosphorus can be removed by solidification.
Vacuum refining is a comparatively effective process for the elimination of phosphorus from silicon melts. Experiments to remove phosphorus under vacuum conditions have been reported by SUZUKI et al[7], IKEDA and MAEDA[8], YUGE et al[9], PIRES et al[10], MIYAKE et al[11], ZHENG et al[12-13]. But vacuum refining from Si-P binary system has never been investigated. The present work will execute vacuum refining process based on the Si-P binary system.
2 Theory calculations
2.1 Thermodynamics
According to the investigation of MIKI et al[14], the Gibbs energy change of phosphorus dissolution into molten silicon is determined in the temperature range from 1 723 to 1 848 K by equilibrating a molten silicon-phosphorus alloy in a controlled phosphorus partial pressure and is expressed as follows:
(1)
(2)
The Gibbs energy change has a relationship with reaction equilibrium constant of Eq.(1) as
(3)
So that, the equilibrium partial pressure of species P2 can be derived from Eq.(3):
(4)
The Gibbs energy change of the reaction from gas species P2 to species P is [14]
(5)
(6)
Similarly, the equilibrium partial pressure of species P can also be derived as follows:
(7)
(8)
(9)
(10)
The Gibbs energy change of the reaction from gas species P2 to species P4 is[15]
(11)
(12)
Therefore, the equilibrium partial pressure of species P4 can be analogized as
(13)
(14)
(15)
(16)
where K is the equilibrium constant; patm is atmospheric pressure (101 325Pa); and fP is the activity coefficient of phosphorus relative to 1% (mass fraction) in liquid silicon. According to the Wagner Model[16]:
(17)
where εij is the activity interaction coefficient. When taking no account of the effects of other impurities, we have
(18)
Where[17]
(19)
The equilibrium partial pressures of species P, P2 and P4 can be calculated and plotted as a function of phosphorus concentration in Fig.2.
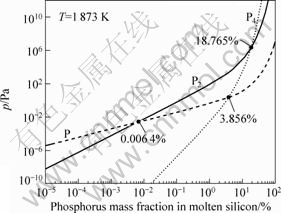
Fig.2 Equilibrium partial pressure of species P, P2 and P4 as function of phosphorus concentration in molten silicon at 1 873 K
As shown in Fig.2, at 1 873 K, monatomic gas species P will be dominant in the gas phase when the phosphorus mass fraction in silicon is less than 0.006 4% (64ppmw), which agrees with the research of MIKI et al[14] that at low phosphorus concentrations below 0.005% (50ppmw), monatomic phosphorus vapor is dominant in the gas phase at 1 823 K.
Species P2 will become the main gas at phosphorus mass fraction range of 0.006 4%-18.765%. The partial pressure of species P4 will greater than that of species P when phosphorus concentration is greater than 3.865%. Species P4 dominates in the gas when the phosphorus concentration is over 18.765%. We believed phosphorus evaporating from molten silicon mainly as gas species P and P2 due to the fact that phosphorus concentration in metallurgical grade silicon (MG-Si) is normally around 0.01% (100ppmw).
2.2 Kinetics
Evaporation at a vacuum follows the Hertz-Langmuir-Knudsen equation reviewed by HICKMAN in 1966[18]
(20)
where α is the evaporation coefficient (0≤α≤1). According to LANGMUIR[19], α is close to unity for a vapor with high molecular mass under vacuum condition. When the vapor evaporates into a finite pressure gas rather than into a vacuum, the evaporation coefficient α will no longer be unity. CHATTERJEE et al[20] reported a vaporization coefficient of butylated hydroxytoluene (BHT) as α≈5.8×10-5.
A simulation model of phosphorus removal from silicon at finite pressures has been investigated by ZHENG et al[21]. The gas species of phosphorus evaporating from molten silicon is believed to be gas species P and P2, and they follow first and second order kinetics, respectively:
(21)
(22)
A mass balance of phosphorus from the above two equations gives
(23)
Integrating Eq.(23) from initial state (t0, w(P)0) to final state (t, w(P)), we obtain
(24)
The original phosphorus content (w(P)0), the crucible size (A), the silicon liquid volume (V), the chamber pressure (p) and the temperature (T) can be measured during the experiments, after calculating out the mass transfer coefficient of species P and P2, a simulation of phosphorus removal by vacuum refining is easy to be carried out.
Based on this model, phosphorus content as a function of chamber pressure is plotted in Fig.3 by setting
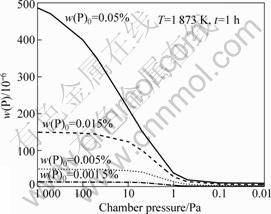
Fig.3 P mass fraction in Si-P alloy as function of chamber pressure calculated from Ref.[21]
original phosphorus concentration as a variation. The other parameters are taken from the experimental condition.
As shown in Fig.3, when phosphorus content in silicon is as high as couple hundreds, phosphorus removal highly depends on high chamber pressure. When phosphorus content in silicon is less than 0.005% (50ppmw), no obvious phosphorus can be evaporated from silicon at high chamber pressure of 6-7 Pa. The curves in Fig.3 also show that phosphorus removal is mostly independent of low chamber pressure. The reason is that phosphorus evaporates from molten silicon as gas species P2 at relatively high phosphorus concentration, while gas species P will be dominated in its vapour at low phosphorus content.
3 Experimental
A vacuum induction furnace from NTNU Norway called ‘B?lzer’ was used for vacuum refining. The copper coil was positioned approximately at the centre of a water-cooled vacuum chamber with 0.6 m in inner diameter and 0.6 m in depth. The carbon crucible with high purity (Carbone Lorraine Grade 2020) and 0.04 m in inner diameter, 0.05 m in outer diameter and 0.09 m in depth was located in the medium of copper coil and surrounded by insulation graphite felt.
Si-P master alloy was made from electronic grade silicon and red phosphorus. At each experiment, 80 kg Si-P alloy was used. In order to avoid the effects of phosphorus evaporation during the melting stage, melting process was carried out under argon pressure of above 60 kPa. During the refining process, the temperature was measured continuously with B-type thermocouple (platinum-30% rhodium vs. platinum-6% rhodium). With continuous temperature measurement and manual control of the power input to the furnace, the temperature change of the melt could be maintained at a constant within ±10 K. The silicon was cooled down in the crucible without casting and the ingot was sawed, polished and analyzed by GD-MS (in Fig.4). The experimental parameters and their ranges of variation are listed in Table 2.
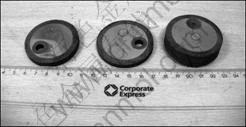
Fig.4 Samples for GD-MS analyses
Table 2 Experimental parameters and their ranges of variation
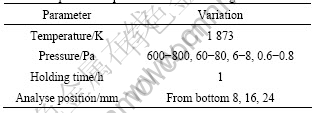
4 Results and discussion
The experimental data of phosphorus removal from Si-P binary system by induction vacuum refining at different vacuum pressures are plotted in Fig.5, where the conditions are 1 873 K and 1 h.
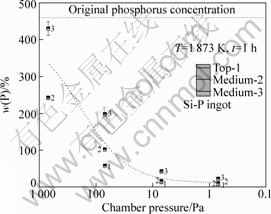
Fig.5 Plots of phosphorus concentration to chamber pressure
As shown in Fig.5, phosphorus can be decreased from 0.046% (460ppmw) to 0.001% (10ppmw) at the condition of temperature 1 873 K, chamber pressure 0.6-0.8 Pa, holding time 1 h. Phosphorus content is inhomogeneous and increases from the top to bottom of the Si-P ingot, since the cooling starts from the top and the segregation coefficient of phosphorus in silicon is 0.35[19]. Phosphorus also can be removed by 30%-50% at high chamber pressure of 600-800 Pa by induction refining starting with original P mass fraction of 0.046% (460ppmw). Phosphorus removal is quite dependent on high chamber pressure while it becomes independent on low chamber pressure, which agrees with our simulation model[21].
Comparison of theory calculation and experimental data from the work of present authors[21] and the data from YUGE et al[9] is shown in Fig.6.
As shown in Fig.6, experiments in pilot scale executed under high vacuum agree very well with the numerical results, while the deviation does exist in small scale experiments which have been carried out under high reduced pressure.
The deviation between the model and the experimental data indicates that the value of evaporation
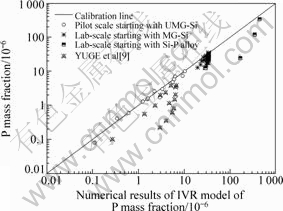
Fig.6 Comparison of experimental data and numerical results of IVR Model
coefficient (α) should not be taken as unity when a perfect vacuum is not attained. On the other hand, the increase of the surface area of silicon liquid should not be ignored, so the surface area will increase by induction stirring, especially significant in lab scale experiments. The effects of other impurities on phosphorus evaporation should be taken into consideration as well. More investigation will be carried out.
5 Conclusions
1) Phosphorus evaporates from molten silicon as gas species P and P2. Phosphorus evaporates from molten silicon as gas species P2 at high phosphorus concentration, while gas species P is dominated in its vapour at low phosphorus content.
2) Elimination of phosphorus from Si-P binary system by induction refining at a finite reduced pressure is carried out. All the samples are analyzed by GD-MS. Phosphorus can be decreased from 0.046% (460ppmw) to around 0.005% (10ppmw) under the condition of temperature 1 873 K, chamber pressure 0.6-0.8 Pa, holding time 1 h.
3) Phosphorus removal is quite dependent on high chamber pressure while it becomes independent on low chamber pressure, which agrees with our simulation model.
4) The deviation between the experimental data and the numerical results is due to the decrease of evaporation coefficient under reduced pressure without perfect vacuum, the increase of surface area by induction stirring and the effects of other impurities in silicon.
Nomenclature
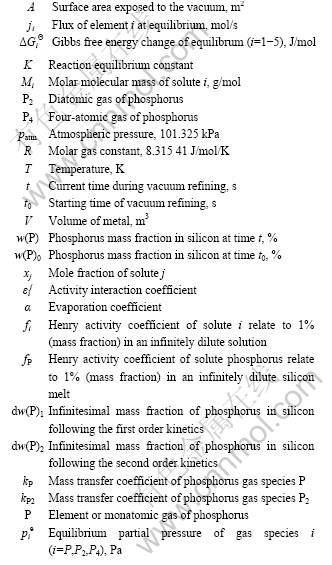
References
[1] BATHEY B R, CRETELLA M C. Review solar-grade silicon [J]. Journal of Materials Science, 1982, 17(2): 3077-3096.
[2] GRIBOV B G, ZINOV'EV K V. Preparation of high-purity silicon for solar cells [J]. Inorganic Materials, 2003, 39(7): 653-662.
[3] DIETL J. Hydrometallurgical purification of metallurgical-grade silicon [J]. Solar Cells, 1983, 10(4): 145-154
[4] KODERA H. Diffusion coefficients of impurities in silicon melt [J]. Japanese Journal of Applied Physics, 1963, 2(4): 212-219.
[5] DAVIS J R, ROHATGI A, H HOPKINS R, BLAIS P D, RAI-CHOUDHURY P, MCCORMICK J R, MOLLENKOPF H C. Impurities in silicon solar cells [J]. IEEE Transactions on Electron Devices, 1980, 27(4): 677-687.
[6] SIGMUND H. Solubilities of magnesium and calcium in silicon [J]. Journal of the Electrochemical Society, 1982, 129(12): 2809-2812.
[7] SUZUKI K, SAKAGUCHI K, NAKAGIRI T, AND SANO N. Gaseous removal of phosphorus and boron from molten silicon [J]. J Japan Inst Metals, 1990, 54(2): 161-167. (in Japanese)
[8] IKEDA T, MAEDA M. Purification of metallurgical silicon for solar-grade silicon by electron beam button melting [J]. ISIJ International, 1992, 32(5): 635-642.
[9] YUGE N, HANAZAWA K, NISHIKAWA K, TERASHIMA H. Removal of phosphorus, aluminum and calcium by evaporation in molten silicon [J]. J Japan Inst Metals, 1997, 61(10): 1086-1093. (in Japanese)
[10] PIRES J C S, OTUBO J, BRAGA A F B, MEI P R. The purification of metallurgical grade silicon by electron beam melting [J]. Journal of Materials Processing Technology, 2005, 169(1): 16-20.
[11] MIYAKE M, HIRAMATSU T, MAEDA M. Removal of phosphorus and antimony in silicon by electron beam melting at low vacuum [J]. J Japan Inst Metals, 2006, 70(1): 43-46. (in Japanese)
[12] ZHENG Song-sheng, CAI Jing, CHEN Chao, LUO Xue-tao. Thermodynamics of phosphorus removal from silicon melts by vacuum induction refining [C]//The 3rd International Workshop on Science and Technology of Crystalline Si Solar Cells (CSSC-3). Trondheim, Norway: Norwegian University of Science and Technology, 2009.
[13] ZHENG Song-sheng, CHEN Chao, LUO Xue-tao. Research progress in phosphorus removal by metallurgical refining [J]. Materials Review, 2009, 23(19): 11-14, 19. (in Chinese)
[14] MIKI T, MORITA K, SANO N. Thermodynamics of phosphorus in molten silicon [J]. Metallurgical and Materials Transaction B, 1996, 27B(6): 937-941.
[15] SCHLESINGER M E. The thermodynamic properties of phosphorus and solid binary phosphides [J]. Chem Rev, 2002, 102(11): 4267-4301.
[16] WAGNER C. Thermodynamics of alloys [M]. Cambridge: Addision-wesley Press, Inc., 1952: 51-53.
[17] SHIMPO T, YOSHIKAWA T, MORITA K. Thermodynamic study of the effect of calcium on removal of phosphorus from silicon by acid leaching treatment [J]. Metallurgical and Materials Transactions B, 2004, 35(4): 277-284.
[18] HICKMAN K. Reviewing the evaporation coefficient [J]. Desalination, 1966, 1(1): 13-29.
[19] LANGMUIR I. The vapor pressure of metallic tungsten [J]. Physical Review (second series), 1913, 2(5): 329-342.
[20] CHATTERJEE K, DOLLIMORE D, ALEXANDER K. A new application for the Antoine equation in formulation development [J]. International Journal of Pharmaceutics, 2001, 213(10): 31-44.
[21] ZHENG S, ENGH T A, TANGSTAD M, LUO X. A simulation of phosphorus removal by induction vacuum refining [C]//Renewable Energy Research Conference. Trondheim, June 2010.
[22] ZHENG Song-sheng, ENGH T A, TANGSTAD M, LUO Xue-tao. Numerical simulation of phosphorus removal from silicon by induction vacuum refining [J]. Metallurgical and Materials Transactions A, DOI: 10.1007/s11661-011-0621-3.
有限负压下熔体硅中磷的挥发去除
郑淞生1, 2, Jafar SAFARIAN2, Seongho SEOK3, Sungwook KIM3, Merete TANGSTAD2, 罗学涛1
1. 厦门大学 材料科学与工程系,厦门 361005, 中国;
2. Department of Material Science, Norwegian University of Science and Technology, Trondheim 7034, Norway;
3. Silicon Refining Research Team, Research Institute of Science and Technology, Pohang 790-600, Korea
摘 要:对有限负压下熔体硅中磷的挥发去除进行研究。采用电子级硅配制Si-P合金,并采用GD-MS来检测实验前后硅中的磷含量。理论计算结果表明:在有限负压下,硅中的磷以P和P2的气体形式从熔体硅中挥发。实验结果显示:在温度1 873 K、真空度0.6-0.8 Pa、熔炼3 600 s的条件下,熔体硅中的磷从0.046% (460ppmw)下降到0.001% (10ppmw)。实验结果与理论结果一致表明:当熔体硅中磷的含量大且炉腔内气压相对较高时,磷的去除与气压高度相关;而当炉腔气压很低时,磷的去除基本与气压无关。原因是在相对高磷含量的熔体硅中,磷主要以P2气体的形式挥发;在磷含量较低时,磷主要以单原子气体P的形式挥发。
关键词:磷浓度;熔体硅;平衡分压;温度;真空度
(Edited by YANG Hua)
Foundation item: Project (2007J0012) supported by the Natural Science Foundation of Fujian Province, China; Project (2007HZ0005-2) supported by the Key Technological Program of Fujian Province, China; Project (BASIC-10341702) supported by Norwegian Research Council
Corresponding author: LUO Xue-tao; Tel: +86-59-22184881; E-mail: xuetao@xmu.edu.cn; Tangstad MERETE; Tel: + 47-73-594938; E-mail: merete.tangstad@material.ntnu.no
DOI: 10.1016/S1003-6326(11)60768-1