Article ID: 1003-6326(2005)02-0432-05
Laser cladded AlCuFe+Sn quasicrystalline
composite coatings
FENG Li-ping(冯莉萍)1, 2, SHAO Tian-min(邵天敏)1,
JIN Yong-ji(金永吉)1, E.Fleury3, D.H.Kim4,
CHEN Da-rong(陈大融)1, WEI Yue-guang(魏悦广)2
(1. State Key Laboratory of Tribology, Tsinghua University, Beijing 100084, China;
2. State Key Laboratory of Non-Linearity Mechanics, Insititute of Mechanics, Chinese Academy of Sciences, Beijing 100080, China;
3. Korea Institute of Science and Technology, Seoul 130-650, Korea;
4. Center for Noncrystalline Materials, Yonsei University, Seoul 120-749, Korea)
Abstract: Al-Cu-Fe+Sn quasicrystalline(QC) composite coatings with different volume fractions of Sn, i.e.12%, 20% and 30%, were prepared by laser cladding technique. The effects of soft phase Sn and processing parameters on the microstructure, microhardness and frictional behavior of the coatings were investigated. The results show that after laser cladding, i-phase existing in the powder is decomposed and element Sn reacts with Cu, forming β-CuSn. The volume fraction of Sn addition has less obvious effect on the microstructure, microhardness and friction performance than that of plasma sprayed coatings. The best performance in terms of microhardness and friction are obtained for the coating containing 20% Sn additions prepared with the laser power of 950W and scanning velocity of 3mm/s.
Key words: AlCuFe+Sn composite; laser cladding; coating; tribology CLC number: TG113; TG115
Document code: A
1 INTRODUCTION
Research on quasicrystalline(QC) materials has demonstrated many desirable characteristics such as high hardness[1, 2], oxidation and corrosion resistance[3], low thermal, low electrical conductivity and unusual optical properties[4, 5] and plasticity at elevated temperature[6, 7]. The shortcoming of quasicrystals generally manifests itself as extreme brittleness in bulk form. To reduce the impact of their brittle nature, quasicrystals have been grown as thick and thin coatings. A modern tribological coating is an engineered surface system that consists of multiple layers and phases[8, 9]. Incorporation of different functional components allows a coating system to have a combination of properties that were previously considered mutually exclusive, e.g. high hardness and high toughness, or high toughness and low friction. Quasicrystals with low surface energy and high hardness suggest them may have significant potential as tribological materials[10-12]. Just the same as other brittle materials, the addition of a ductile phase enables significant improvement of the fracture toughness[13, 14]. Therefore, production of quasicrystalline composites, which takes full of the advantage of QC and avoids their major drawbacks, becomes a feasible way for their applications. Resent studies have demonstrated that the addition of a ductile phases such as FeAl and Sn in QC coatings give approximately a threefold increase of the wear resistance[15, 16]. Plasma spray is particularly appropriate for preparation of coatings containing a high fraction of i-phase, but the drawback of this technique is the inferior microstructure features such as interlamellar and pores, intra- and inter-splat microcracks and splat boundaries generally observed in the coatings. These features greatly influence the physical and mechanical properties[17, 18]. But the techniques of laser remelted - plasma sprayed or laser cladded can eliminate these defects and become one potential technique to fabricate composite coatings comprised icosahedral phase with high wear resistance[19, 20]. This work was thus undertaken with the aim of identifying the role of a ductile phase Sn and processing parameters on the room temperature tribological behavior of laser cladded quasicrystalline composites coatings. The wear performance of laser-remelted plasma sprayed coatings was significantly improved only for the coating containing a high volume fraction of i-phase.
2 EXPERIMENTAL
Gas atomized Al-Cu-Fe based composite powders with different volume fraction(12%, 20%, 30%, respectively) of Sn were selected for deposition of QC composite coatings[14]. The powder size of 38-60μm was selected and d24mm×10mm medium carbon steel (0.45%C, 0.65%Mn and 0.2%Si) discs were used as substrates. A CW YAG laser was employed for laser cladding experiments. Detailed descriptions of the processing conditions used for the laser cladded coatings are given in Table 1. A parameter Θ, defined as the ratio of laser power and scanning velocity, (Θ=P/v), was used to evaluate influences of the processing parameters on the microstructure and mechanical property. Spot diameter was 5mm. Argon was used as shielding gas. Five tracks were cladded on the substrate side by side with an overlap ratio of approximately 30%.
A DM-400 micro-indenter was used for measuring the microhardness of the coatings. Friction and wear behavior of the laser cladded QC composite coatings were investigated with a ball on disk SRV friction and wear test machine under reciprocating motion. Balls of 12.7mm in diameter, made of GCr15 bearing steel(1%C, 0.3%Mn and 1.5%Cr) with hardness of Hv742 were used. The applied load, frequency and reciprocating stroke were set as 10N, 50Hz and 0.6mm, respectively. All tests were carried out at room temperature and under non-lubrication conditions. X-ray diffraction(XRD) and scanning electron microscope(SEM) with energy dispersive spectrometer(EDX) analysis system were employed for phase identification, microstructural characterization and composition analysis of the coatings.
3 RESULTS AND DISCUSSION
3.1 XRD analysis
Fig.1 shows the XRD patterns of Al-Cu-Fe+20%Sn atomized powders and coatings. In Fig.1(a), the powders composed of the icosahedral(i), Sn(tetragonal) and ε2-Al2Cu3 (hexagonal approximant) phases can be observed. After laser processing(Fig.1(b)), phases in the cladding layers were identified as β-CuSn, α-AlFe(Cu) with elemental phase Sn. The Sn and CuSn content in the coatings varied with the initial Sn content in the powder and the processing parameters, however not straightforward variation could be worked-out. XRD results suggested that i-phase in the powder was decomposed, and part of the elemental phase Sn reacted with Cu during laser cladding, forming β-CuSn phase. The microstructures were quite different from that of plasma sprayed coatings[15], in which Sn appeared as elemental phase and there was no significant change in the volume fraction of i-phase before and after plasma spraying. The difference in phases resulted from the different solidification mechanisms of these two techniques. During laser cladding, particles were fully melted and elements reacted with each other, causing new phases formation. While in plasma spraying, coatings were formed by the building-up of partially liquefied droplets flatten with high cooling rates onto a substrate[17, 18]. The relatively lower temperatures and high solidification rates prevents the decomposition of the i-phase and reaction of Sn. The plasma sprayed coatings, therefore, always contain a high content of i-phase.
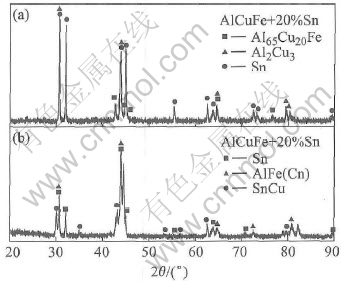
Fig.1 XRD patterns of Al-Cu-Fe/Sn composite powder and coatings with 20%Sn(CuKα)
Table 1 Processing parameters of laser cladding
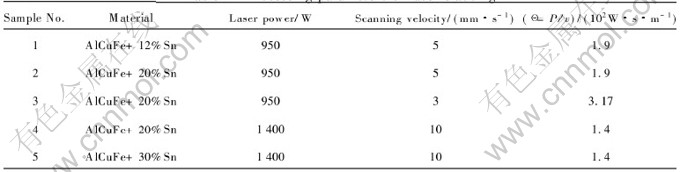
3.2 Microhardness
The microhardness values for the five samples were 337, 357, 383, 354 and 357Hv, respectively. The existence of hard phases such as β-CuSn(Hv 600-800[21]) and α-AlFe(Cu)(Hv 775[22]) formed during laser cladding together with the lower defect content resulted in harder coatings in comparison to plasma sprayed coatings[15]. Furthermore, the microhardness exhibited little dependency on the Sn content. The microhardness of the plasma sprayed coatings was found to decrease linearly with the Sn volume fraction from 376 to 147 Hv. In as-cast ingots and plasma sprayed coatings, the Sn existed as element phase, thus the addition of 10%Sn (soft phase) led to approximately 20% reduction in microhardness. In contrast, the variation of the microhardness of laser cladded coatings could be explained by the formation of β-CuSn(hard phase), owing to the lower cooling rate. The microhardness of laser cladded coatings is thus dependent on the processing conditions, and, for samples with the same Sn content(No.2, 3 and 4), the higher the Θ value, the harder the coating.
3.3 Friction and wear test
The time-dependence of the friction coefficient of the laser cladded coatings is shown in Fig.2. The Al-Cu-Fe+20% Sn composite and the laser processing with low scanning velocity gave satisfying friction performance (samples No.2 and 3). But on the whole, the friction coefficient remains relatively stable and the influence of Sn addition on the friction coefficient is not significant. The frictional behavior of plasma sprayed coatings showed different results. The Sn in plasma sprayed coatings, existing as a ductile element phase, led to rising of friction coefficient because of the strong adhesion mechanism[15]. In laser cladded coatings, part of Sn existed in the form of β-CuSn. Although there was no i-phase observed, the dispersion of hard phases β-CuSn and α-AlFe(Cu) in the coatings resulted in the variation of the coefficient of friction. And for theses five samples, the hardest coatings correspond to the lowest friction coefficient. The laser cladded coatings show more satis fying wear resistance as viewed from slightly lower friction coefficients than that of the plasma sprayed coatings with same Sn addition.
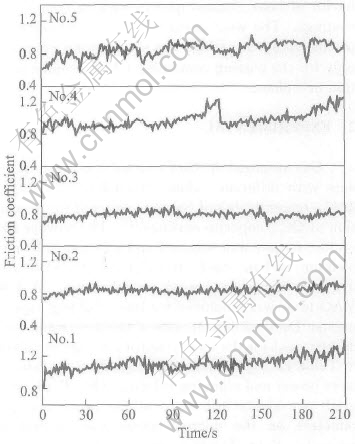
Fig.2 Variation of friction coefficient of laster cladded Al-Cu-Fe+Sn coatings
3.4 SEM and EDX analysis
The SEM images of the wear scar of the laser cladded Al-Cu-Fe+20%Sn composite coatings(sample No. 3) and of the counter ball are shown in Fig.3. The morphology of the wear scar of the coatings was obvious plastic flow. A thin layer covered the surface of counter ball. The EDX analyses on the wear scar and wear debris indicated that the material of transfer layer mainly came from the coatings. These suggested the wear mechanism was adhesive wear. Fleury et al[12] investigated the tribological behavior of thermal-sprayed quasicrystalline layers. It was found that the transfer film on Cr-coated disc(counterpart) was formed during dry sliding friction. Based on the above analysis, in the present investigation, the friction system under dry sliding conditions has evolved from quasicrystal against the hardened GCr15 steel disc into quasicrystal has much higher hardness compared to the plasma sprayed coatings. The other evidence that the wear resistance of plasma sprayed coating higher than that of the laser cladded one is that the width, height of the wear scar of laser cladding sample is smaller than that of the plasma sprayed coating with same Sn addition(the test time are both 500s), indicating that the wear resistance of laser cladded coatings is better than that of the plasma sprayed ones. For samples No.2 and 4 with 20%Sn addition produced by higher scanning velocity(not shown), the wear damage of the coating was more significant than that of sample No.3. The surface track exhibited narrow and shallow plowing grooves and plastic flow. The more pronounced wear damage was observed for samples No.1 and 5 with 12% and 30%Sn, respectively, which exhibited wear track morphologies characterized by deep plowing grooves and plastic flow. The worn debris was mainly composed of elements from the coating, as indicated by the EDX analyses. The wear mechanism of the four more damaged samples was a combination of plowing and adhesion phenomena. It also can be observed that the Al-Cu-Fe+20 %Sn composite and the laser processing with 3mm/s exhibits the best friction performance, based on the obvious reduction of worn degree of the coating.
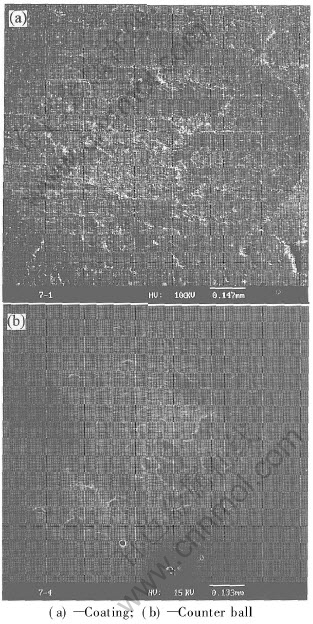
Fig.3 SEM micrographs of wear scar of couples
4 CONCLUSIONS
AlCuFe+Sn composite coatings were prepared by laser cladding. The microstructure of laser cladded coatings differed greatly from that of plasma sprayed coatings. The initial i-phase of the powder decomposed and a significantly high proportion of Cu reacted with element Sn during laser cladding. Both Sn content and processing parameters influenced the tribological behavior of the coatings. The major wear mechanism was identified as the adhesive wear caused by plastic flow. The Al-Cu-Fe+20%Sn composite coating prepared under suitable processing parameters exhibited the highest value of hardness and the best friction performance. The tribological behavior of laser cladded coatings is better than that of the plasma sprayed coatings.
REFERENCES
[1]Koester U, Liu W, Liebert H, et al. Mechanical properties of quasicrystalline and crystalline phases in Al-Cu-Fe alloys [J]. J Non-Crystall Sol, 1993, 153-154: 446-452.
[2]Wolf B, Bambauer K O, Paufler P, et al. On the temperature dependence of the hardness of quasicrystals [J]. Mat Sci Eng A, 2001, A298: 284-295.
[3]Rudiger A, Koester U. Corrosion behavior of Al-Cu-Fe quasicrystals [J]. Mater Sci Eng A, 2000, 294-296: 890-893.
[4]Dubois J M, Plaindoux P, Belin-Ferre E, et al. Surface energy of icosahedral quasicrystals [A]. Quasicrystals ICQ6 [C]. Singapore: World Scietific, 1997, 733-740.
[5]Archambault P, Janot C. Thermal conductivity of quasicrystals and associated processes [J]. MRS Bull. 1997, 22(11): 48-51.
[6]Feng L P, Shao T M, Jin Y J, et al. Temperature dependence of tribological properties of laser re-melted Al-Cu-Fe quasicrystalline plasma sprayed coatings [J]. Journal of Non-Crystalline Solids, 2005, 351: 280-287.
[7]Kang S S, Dubois J M, von Stebut J. Tribology properties of quasicrystalline coatings [J]. J Mater Res, 1993, 8(10): 2471-2481.
[8]Yust C S. Tribological behavior of whisker reinforced ceramic composite materials [A]. Friction and Wear of Ceramics [C]. New York: Marcel Dekker, 1994, 199-224.
[9]Hornbogen E. Description and wear of materials with heterogeneous and anisotropic microstructures [J]. Wear, 1986, 111(4): 391-402.
[10]Zhou C, Cai F, Kong J, et al. A study on the tribological properties of low-pressure plasma-sprayed Al-Cu-Fe-Cr quasicrystalline coating on titanium alloy [J]. Surface & Coatings Technology, 2004, 187: 225-229.
[11]Huttunen-Saarivirta E. Microstructure, fabrication and properties of quasicrystalline Al-Cu-Fe alloys: a review [J]. Journal of Alloys and Compounds, 2004, 363: 150-174.
[12]Fleury E, Lee S M, Kim J S, et al. Tribological properties of Al-Ni-Co-Si quasicrystalline coatings against Cr-coated cast iron disc [J]. Wear, 2002, 253: 1057-1069.
[13]Kragness E D, Amateau M F, Messing G L. Processing and characterization of laminated SiC whisker reinforced Al2O3 [J]. J Composite Mater, 1991, 25: 416-432 .
[14]Dubois J M, Brunet P, Costin W, et al. Friction and fretting on quasicrystals under vacuum [J]. Journal of Non-Crystalline Solids, 2004, 334-335: 475-480.
[15]Shao T M, Cao X K, Fleury E, et al. Tribological behavior of plasma sprayed Al-Cu-Fe+Sn quasicrystalline composite coatings [J]. Journal of Non-Crystalline Solids, 2004, 334-335: 466-470.
[16]Fleury E, Kim Y C, Kim D H, et al. The toughening of Al-Cu-Fe(-B) quasicrystals by Sn particles [J]. Journal of Non-Crystalline Solids, 2004, 334-335: 449-453.
[17]Wang Z, Kulkarni A, Deshpande S, et al. Effects of pores and interfaces on effective properties of plasma sprayed zirconia coatings [J]. Acta Mater, 2003, 51: 5319-5334 .
[18]Sordelet D J, Besser M F, Logsdon J L. Abrasive wear behavior of Al-Cu-Fe quasicrystalline composite coatings [J]. Mat Sci Eng A, 1998, A255: 54-65.
[19]Biswas K, Galun R, Mordike B L, et al. Laser cladding of quasicrystal forming Al-Cu-Fe on aluminum [J]. Journal of Non-Crystalline Solids, 2004, 334-335: 449-452.
[20]Yuan W D, Shao T M, Fleury E, et al. Microstructure and tribological properties of plasma sprayed Al-Cu-Fe quasicrystalline coatings after laser post-treatment processing [J]. Surface & Coatings Technology, 2004,185: 99-105.
[21]WEN Shi-zhu, HUANG Ping. Principle of Tribology [M]. Beijing: Tsinghua University Press, 2002. 339-342.
[22]Kster U, Liebertz H, Liu W. Plastic deformation of quasi-crystalline and crystalline phases in Al-Cu-Fe alloys [J]. Mater Sci Eng A, 1994, 181-182: 777-780.
Foundation item: Project(50075042) supported by the National Natural Science Foundation of China; Project(2003034143) supported by the Postdoctoral Foundation of China
Received date: 2004-11-20; Accepted date: 2005-01-18
Correspondence: FENG Li-ping, PhD; Tel: +86-10-62556053; Fax: +86-10-62781379;
E-mail: fenglp@lnm.imech.ac.cn
(Edited by LI Xiang-qun)