J. Cent. South Univ. (2012) 19: 1196-1201
DOI: 10.1007/s11771-012-1128-9
Microstructure and mechanical properties of 8YSZ ceramics by liquid-phase sintering with CuO-TiO2 addition
ZHOU Hong-ming(周宏明), GUO Yan-jun(郭雁军), LI Jian(李荐), YI Dan-qing(易丹青), XIAO Lai-rong(肖来荣)
School of Materials Science and Engineering, Central South University, Changsha 410083, China
? Central South University Press and Springer-Verlag Berlin Heidelberg 2012
Abstract: The 8% (mass fraction) yttrium-partially-stabilized zirconia (8YSZ) ceramic was fabricated via liquid phase sintering at 1 200-1 400 ℃ by adding different mass ratios of CuO-16.7%TiO2 (molar fraction) as sintering aid. Relative density, microstructure, Vickers hardness and bending strength as a function of sintering temperature and additive content were investigated. The experiment results show that liquid phase sintering at low temperature can be realized through adding CuO-16.7% TiO2 to 8YSZ. The Vickers hardness and bending strength of samples with sintering aid are generally much higher than those of samples without sintering aid for all sintering temperatures, and increase with the increase of sintering temperature. When the addition content of CuO-16.7% TiO2 is beyond 0.5%, the relative density, Vickers hardness and bending strength decrease with the increase of the mass ratio of sintering aid. Low additions of sintering aid are beneficial to aiding densification; high additions of sintering aid are detrimental to the sintered properties mainly due to greater amounts of pores generated by the volatilization of oxygen with the eutectic reaction between copper oxide and titanium dioxide. It is found that the fine grain size and high relative density are two main reasons of the high bending strength and Vickers hardness of the materials.
Key words: liquid-phase sintering; 8YSZ ceramic; microstructure; mechanical properties; CuO-TiO2
1 Introduction
Typically, 8% (mass fraction) yttrium-partially- stabilized zirconia (8YSZ) is widely utilized to prepare thermal barrier coatings [1-2], due to their excellent thermal resistance, good mechanical properties such as high bending strength and fracture toughness. Plasma spraying and chemical/physical vapor deposition [3-4] are currently common industrial methods to prepare 8YSZ thermal barrier coatings. However, they all have limitations for handling axially symmetric and complex-shaped substrates, and require expensive equipment. Colloidal processing coating techniques, such as slurry spraying/dip coating [5-6] and electrophoretic deposition (EPD) [7-8], provide the potential to fabricate ceramic coatings with the advantages of less restriction in substrate shape, controlled chemical composition and microstructure, simple equipment requirements and low cost. However, the main problem associated with these processes is the difficulty in sintering of the coatings. Commonly, the sintering temperatures of 8YSZ are above 1 400 ℃. High temperature may damage metal substrates and metal coatings.
In order to lower the sintering temperature of 8YSZ, two main methods are proposed. First, the sintering temperature of 8YSZ is lowered to 1 100 ℃ by using nano-powder of 8YSZ [9]. Second, liquid phase or activated sintering is applied to lowering the sintering temperature of 8YSZ [10-11]. Many studies were reported concerning the effects of additives on sintering, e.g. SiO2 [12], Bi2O3 [13], B2O3 [14], CAS glass [15], CuO, TiO2, or MnO2 [16-18]. The addition of 1%-3% (molar fraction) Bi2O3 [13] lowers the sintering temperature from 1 600 to 1 080 ℃ while making ceramics have a comparable density (92%-95%) and the grain size decrease from 90 to 5 μm. HUANG et al [19] got densified 3% (molar fraction) Y2O3-ZrO2 composite at temperature below 1 400 ℃ by doping small amount of TiO2-MnO2. ZHANG et al [20] found that, by adding small amount of CuO-TiO2, the alumina ceramic reached above 98% of the theoretical density at 1 250 ℃.
In most cases, the presence of additives resulted in improved densification of the ceramics via liquid-phase sintering. Figure 1 shows the binary phase diagram of CuO-TiO2 [21]. It is indicated that when the content of TiO2 is 16.7% (molar fraction), liquid phase will be generated at 919 ℃. However, liquid-phase sintering of 8YSZ with CuO-16.7%TiO2 (molar fraction) addition has received little attention, and the effect of additives on the mechanical properties of the composite remains unknown.
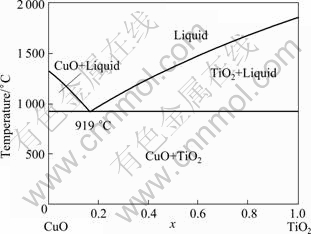
Fig. 1 Binary phase diagram of CuO-TiO2 [21]
The purpose of the present work is to densify 8YSZ composite by pressureless sintering with CuO-16.7% TiO2 (molar fraction) additives and to investigate the effect of the additive on the sintering, microstructure and mechanical properties of the composite.
2 Experimental
2.1 Materials processing
High purity commercial 8YSZ (>99.9%, mass fraction) and analytical-reagent-grade CuO and TiO2 were used as raw materials. The phase composition of 8YSZ powder was analyzed by XRD, and the pattern is shown in Fig. 2.
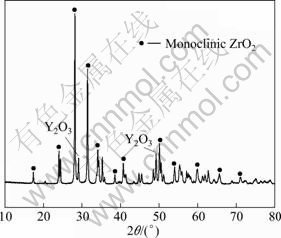
Fig. 2 XRD patterns of 8YSZ powders
Figure 2 indicates that the 8YSZ powder consists of monoclinic phase ZrO2 and a small volume fraction of Y2O3, but the tetragonal and cubic phase ZrO2 could not be discerned. The compositions of 8YSZ composite powder are listed in Table 1.
For the final product of 8YSZ composite, high purity commercial 8YSZ and additives (CuO-16.7%TiO2) were weighed and milled according to the composition listed in Table 1. The 8YSZ and additives powder were mixed in absolute ethyl alcohol via ball milling for 24 h, using a zirconia jar and high-purity ZrO2 balls, then dried and sieved through 100-mesh screen. After adding 2% (mass fraction) PVA and sieving through a 40-mesh screen, the mixtures were pressed uniaxially to rectangular bars at 60 MPa, followed by cold isostatic pressing at 200 MPa.
Table 1 Compositions of 8YSZ composite powders (mass fraction, %)
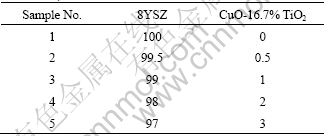
Samples were heated at rate of 5 ℃/min to 400 ℃, held for 60 min to detach PVA, and then heated at rate of 5 ℃/min to the final sintering temperature (1 200-1 400 ℃), held for 4 h, and finally cooled in the furnace.
2.2 Characterization of materials
Crystal-phase identification and phase compositions of the synthesized sample were determined by X-ray diffractometry (XRD) with Ni filtered Cu Ka radiation at a scan rate of 4 (°)/min. The fraction of monoclinic ZrO2 is calculated using the following equation [17]:
(1)
where Im(111),
and It+c(111) represent the intensities of the diffraction patterns from m(111),
and (t+c)(111) reflections, respectively.
The bulk density (D) of the sample was measured by the Archimedes method with an immersion medium of deionized water. The bulk theoretical density (d) was calculated on the basis of the theoretical densities of 5.90 (d1), 6.32 (d2) and 4.25 g/cm3 (d3) for 8YSZ, CuO and TiO2, according to the following equation [17]:
(2)
where X, Y and Z represent the mass fractions of 8YSZ, CuO and TiO2, respectively. Then, the relative density (R) is calculated by
R=D/d (3)
The sintered samples were polished and thermal- etched at temperatures below 100 ℃ of sintering temperature for 30 min. The microstructures were examined by scanning electron microscopy (SEM). Rectangular bars for bending strength tests (3 mm×4 mm in cross section and 35-45 mm in length) were cut, and three-point bending strength measurements were carried out with an inner span of 30 mm and a crosshead speed of 0.5 mm/s at room temperature using an Instron-1195 Universal Test Machine. At least five specimens were tested per composition. Vickers hardness (Hv) was measured on polished samples using the Vicker’s indentation method at loads up to 588 N. The Vickers hardness was obtained using the standard equation for Vickers geometry [18]:
Hv=1.854 4F/d2 (4)
where F is the indentation load, and d is the indent diagonal. Six measurements were made for each sample and average values were obtained.
3 Results and discussion
3.1 Relative density of 8YSZ
The relative densities of samples with different additive contents sintered between 1 200 and 1 400 ℃ are shown in Fig. 3.
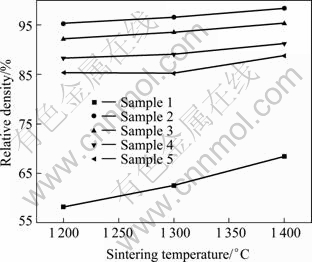
Fig. 3 Relative density of 8YSZ as function of additive content and sintering temperature
It can be seen from Fig. 3 that, the additive has advantages in lowering sintering temperature and enhancing densification. This is due to the change of sintering behavior of the samples. When sintering additive is added, liquid phase can be generated at the sintering temperature, and the sintering behavior is changed from solid-phase sintering to liquid-phase sintering. Liquid phase influences the compactness of 8YSZ in two aspects [22]. First, in the initial stage of sintering, the liquid phase can exert a significant capillary pressure capable of pulling the particles together. And in the case that the liquid phase wets the particles, it can envelop and collapse the pores, resulting in densification. Second, since the solid is to some extent soluble in the liquid [23], the liquid phase can also aid densification by providing a rapid diffusion path-way between the particles in which mass transfer occurs by a dissolution-reprecipitation mechanism. Figure 3 also indicates that the relative densities of all samples increase gradually with elevating the sintering temperature.
Figure 3 indicates that, at the same sintering temperature, the sample with 0.5% additives has the highest relative density, and it decreases gradually with the increase of additive content. The reason for this phenomenon has not been fully understood. This may be attributed to a large amount of liquid phase aggregated at triple grain boundaries and contracted when the sample is cooled, thus many pores are generated, and then the relative density of sample is decreased.
3.2 Microstructure
Figure 4 shows SEM images of polished and thermal-etched surface of samples with different additive contents sintered at 1 300 ℃ for 4 h.
It can be seen from Fig. 4 that, porous structure is observed on the surface of the sample without additive, and the 8YSZ powder particles are just bonded together. However, after 0.5% additives are added into 8YSZ, its surface becomes very compact, and pores and second-phases are not observed; moreover, the grain size of 8YSZ is very fine, about 0.2 μm. When the content of the additive is 1%, the grain size of 8YSZ increases rapidly to about 1 μm, and a small amount of second phases are observed at the grain boundaries. With the content of additive increasing to 3%, the grain size of 8YSZ keeps almost unchanged, about 1 μm, but a large amount of second phases are generated at triple grain boundaries, which is in good agreement with the changing trend of relative density.
Figure 5 shows SEM images of polished and thermal-etched surface of samples with 0.5% additives sintered at different temperatures for 4 h.
It can be seen from Fig. 5 that, with the sintering temperature increasing from 1 200 ℃ to 1 400 ℃, the grain size of 8YSZ increases gradually from 0.2 μm to 0.6 μm. Surfaces of samples sintered at different temperatures are very compact, and pores and second-phases are not observed.
The X-ray diffraction patterns on the polished surface of 8YSZ samples with different additive contents sintered at 1 300 ℃ for 4 h are given in Fig. 6. And the mass fractions of monoclinic ZrO2 in these samples are calculated in Table 2.
Figure 6 and Table 2 indicate that, after sintering in air at 1 300 ℃ for 4 h, the XRD spectra of 8YSZ show peaks of monoclinic zirconia (53.8%) and tetragonal zirconia, which means that large amounts of monoclinic zirconia (46.2%) in 8YSZ powder are transformed into tetragonal zirconia during the sintering process. The high tetragonal phase content in the sintered sample of 8YSZ without sintering additive can be attributed to the fact that high sintering temperature (>1 300 ℃) could allow greater stability of tetragonal phase to room temperature.
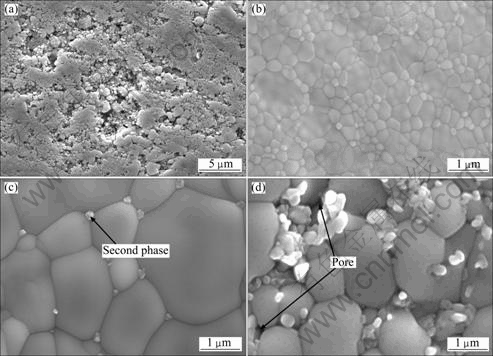
Fig. 4 SEM images of samples with different of additive contents sintered at 1 300 ℃ for 4 h: (a) 8YSZ; (b) 8YSZ+ 0.5%(CuO-16.7%TiO2); (c) 8YSZ+1%(CuO-16.7% TiO2); (d) 8YSZ+3%(CuO-16.7%TiO2)
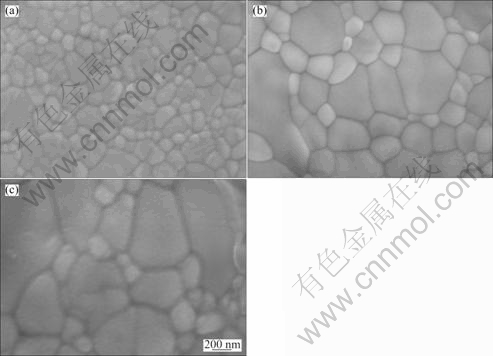
Fig. 5 SEM images of samples with 0.5% additives sintered at different temperatures for 4 h: (a) 1 200 ℃; (b) 1 300 ℃; (c) 1 400 ℃
It can also be seen from Fig. 6 and Table 2 that, XRD spectra of 8YSZ with 0.5% additives show peaks of tetragonal zirconia (94.8%) and a very small fraction of monoclinic zirconia (5.2%), and with the content of additive increasing to 3%, the fraction of monoclinic zirconia increases quickly, which means that the additions of sintering aid are observed to be either beneficial or detrimental to the stability of tetragonal phase in these 8YSZ specimens. For additive content of 0.5%, high tetragonal retention is due to smaller grains from improved sinterability at lower temperatures and to yttrium re-distribution from its dissolution in a Cu-Ti-rich liquid phase during sintering. According to Fig. 4, larger amounts of grain boundary liquid phases are generated from higher CuO-TiO2 contents, and incorporated at triple grain boundary junctions rather than distributed along grain edges. Therefore, the effect of this for the sample with 3% additives in the present work can be attributed to more yttria dissolution [24-25]. Of course, further examination is required to elucidate the compositions and formation mechanism of liquid phase.
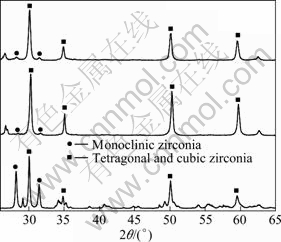
Fig. 6 XRD patterns on polished surface of 8YSZ samples with different additive contents sintered at 1 300 ℃ for 4 h: (a) 8YSZ; (b) 8YSZ+0.5%(CuO-16.7%TiO2); (c) 8YSZ+3% (CuO-16.7%TiO2)
Table 2 Monoclinic ZrO2 fraction in bulk samples
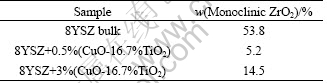
3.3 Mechanical properties
The variations of Vickers hardness with sintering temperature for 8YSZ samples with additive and without additive are shown in Fig. 7.
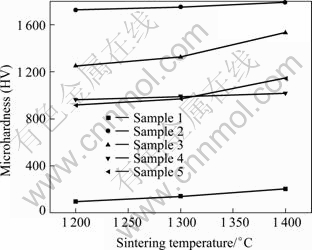
Fig. 7 Vickers hardness of 8YSZ samples as function of additive content and sintering temperature
The additions of CuO-16.7%TiO2 up to 0.5% exhibit the highest Vickers hardness among all the samples throughout the sintering regime employed. Typical values measured for sample with 0.5% additives varies from HV 1 720 to HV 1 796 and the measured Vickers hardness of all the samples reveals a similar trend, i.e. increasing with increasing sintering temperatures. The Vickers hardness of 8YSZ without additive is the lowest among all the samples, which is from HV 152 to HV 205, and is much lower than that of the samples with sintering aid. In general, the increase of Vickers hardness can be attributed to the increase in bulk density.
Figure 8 shows the bending strength of 8YSZ as a function of the sintering temperature and additive content.
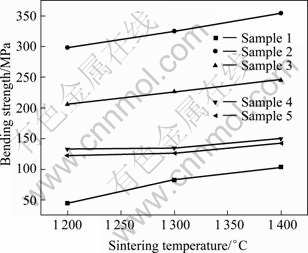
Fig. 8 Bending strength of 8YSZ samples as function of additive content and sintering temperature
It can be seen from Fig. 8 that, the bending strength of sintered samples increases with the increase of sintering temperature, which may be attributed to the increase of relative density. The sample with 0.5% additive has the highest bending strength of (352±10) MPa. When the additive content is beyond 0.5%, the bending strength of the samples will decrease gradually with the increase of additive content.
This microstructure (see Fig. 4) determines the high bending strength of 8YSZ with 0.5% additives. The average grain size is about 0.2 μm and there is little tetragonal phase transforming to monoclinic phase. Therefore, the superfine grain size is the main reason that leads to high bending strength of this material. For ceramics, the relation between grain size and bending strength can be expressed by Hall-Petch equation [26]:
(6)
where σ0 is the bending stress of unit crystal of infinitely great, k is a coefficient, and d is the grain size. According to this equation, the bending stress increases with decreasing grain size. Therefore, although the effect of phase transformation is not obvious in these samples, the bending stress is still high owing to their superfine grain size. In addition, with the decrease of relative density, the needed energy for the crack formation and propagation decreases greatly, which will lead to the decrease of bending strength.
4 Conclusions
1) Liquid phase sintering at low temperature can be realized through adding CuO-16.7% TiO2 (molar fraction) to 8YSZ. The sintering temperature of 8YSZ can be lowered from 1 400 ℃ to 1 200 ℃.
2) An optimum CuO-16.7% TiO2 (molar fraction) addition of <0.5% (mass fraction) exists above which there can be detrimental effects for 8YSZ ceramics, with relative low bulk densities and high monoclinic phase content.
3) The Vickers hardness and bending strength of samples with additive are generally much higher than those of the ceramics without additive, and increase with the increase of sintering temperature. The samples have high bending strength and Vickers hardness owing to their superfine grain size.
4) When the additive content is 0.5% (mass fraction), the sample has the highest Vickers hardness and bending strength. When the addition content of CuO-16.7% TiO2 (molar fraction) is beyond 0.5% (mass fraction), the Vickers hardness and bending strength will decrease with the increase of additive content.
References
[1] CLARKE D R, LEVI C G. Materials design for the next generation thermal barrier coatings [J]. Annual Review of Materials Research, 2003, 33: 383-417.
[2] PADTURE N P, GELL M, JORDAN E H. Thermal barrier coatings for gas turbine engine applications [J]. Science, 2002, 296(5566): 280-284.
[3] CHOY K L. Chemical vapour deposition of coatings [J]. Progress in Materials Science, 2003, 48: 57-170.
[4] BOONE D H. Physical vapor deposition processes [J]. Materials Science and Technology, 1986, 2(3): 220-224.
[5] WANG X, LAN W H, XIAO P. Fabrication of yttria stabilized zirconia coatings by a novel slurry method [J]. Thin Solid Films, 2006, 494: 263-267.
[6] JI C, LAN W H, XIAO P. Fabrication of yttria-stabilized-zirconia coatings using electrophoretic deposition: packing mechanism during deposition [J]. Journal of American Ceramic Society, 2008, 91(4): 1102-1110.
[7] CORNI I, RYAN M. P, BOCCACCINI A R. Electrophoretic deposition: From traditional ceramics to nanotechnology [J]. Journal of the European Ceramic Society, 2008, 28(7): 1353-1367.
[8] JI C Z, SHAPIRO I P, XIAO P. Fabrication of yttria-stabilized- zirconia coatings using electrophoretic deposition: Effects of agglomerate size distribution on particle packing [J]. Journal of the European Ceramic Society, 2009, 29: 3167-3175.
[9] LAN W H, XIAO P. Fabrication of yttria-stabilized-zirconia thick coatings via slurry process with pressure infiltration [J]. Journal of the European Ceramic Society, 2009, 29: 391-401.
[10] BOUTZ M M R, WINNUBST A J A, HARTGERS F, BURGGRAAF A J. Effect of additives on densification and deformation of tetragonal zirconia [J]. Journal of Materials Science, 1994, 29: 5374-5382.
[11] LAWSON S, GILL C. DRANSFIELD G P. The effects of copper and iron oxide additions on the sintering and properties of Y-TZP [J]. Journal of Materials Science, 1995, 30: 3057-3060.
[12] VERKERK M J, WINNUBST A J A, BURGGRAAF A J. Effect of impurities on sintering and conductivity of yttria-stabilized zirconia [J]. Journal of Materials Science, 1982, 17: 3113-3122.
[13] KEIZER K, BURGGRAAF A J, DE G W. The effect of Bi2O3 on the electrical and mechanical properties of ZrO2–Y2O3 ceramics [J]. Journal of Materials Science, 1982, 17: 1095-1102.
[14] BUCHANAN R C, SIRCAR A. Densification of calcia-stabilized zirconia with borates [J]. Journal of American Ceramic Society, 1983, 66(2): C20-C21.
[15] SUN Y, ZHANG Y, GUO J. Microstructure and bending strength of 3Y-TZP ceramics by liquid-phase sintering with CAS addition [J]. Ceramics International, 2003, 29: 229-232.
[16] ERKALFA H, MISIRLI Z, BAYKARA T. Densification of alumina at 1 250 ℃ with MnO2 and TiO2 additives [J]. Ceramics International, 1995, 21: 345-348.
[17] HUANG X W, WANG S W, ZHAO S K, HUANG X X. Effect of liquid-forming additives on the sintering and mechanical properties of Al2O3/3Y-TZP (30 vol. %) composite [J]. Materials Research Bulletin, 2002, 37(10): 1709-1719.
[18] RAMESH S, GILL C, LAWSON S. The effect of copper oxide on sintering, microstructure, mechanical properties and hydrothermal ageing of coated 2.5Y-TZP ceramics [J]. Journal of Materials Science, 1999, 34: 5457-5467.
[19] HUANG X W, WANG S W, HUANG X X. Influence of sintering aids on sintering and mechanical properties of 3Y-TZP/Al2O3 (40 vol. %) composite [J]. Journal of Materials Science Letters, 2002, 21: 1439-1443.
[20] ZHANG Bin, WANG Huan-ping, MA Hong-ping, XU Shi-qing, LI Deng-hao, ZHOU Guang-miao. Mechanism of lowering the sintering temperature of Al2O3 ceramic by the addition of CuO-TiO2 (I) [J]. Chinese Journal of Materials Research, 2009, 5: 534-540. (in Chinese)
[21] LU F H, FANG F X, CHEN Y S. Eutectic reaction between copper oxide and titanium dioxide [J]. Journal of the European Ceramic Society, 2001, 21: 1093-1099.
[22] KINGERY W D. Ceramic fabrication processes [M]. New York: Technology Press of Massachusetts Institute of Technology and John Wiley and Sons, Inc, 1958: 131-143.
[23] LU H Y, CHEN S Y. Sintering and compensation effect of donor- and acceptor-codoped 3mol% Y2O3-ZrO2 [J]. Journal of Materials Science, 1992, 27: 4791-4796.
[24] MECARTNEY M L. Influence of an amorphous second phase on the properties of yttria-stabilized tetragonal zirconia polycrystals (Y-TZP) [J]. Journal of American Ceramic Society, 1987, 70(1): 54-58.
[25] RAMESH S, MEENALOSHINE S, TAN C Y, KELCIN C W J, TENG W D. Effect of manganese oxide on the sintered properties and low temperature degradation of Y-TZP ceramics [J]. Ceramics International, 2008, 34(7): 1603-1608.
[26] ZHOU Yu, JIA De-chang, WEN Guang-wu. Science of ceramics [M]. Harbin: Harbin Institute of Technology Press, 1999: 22-35. (in Chinese)
(Edited by YANG Bing)
Foundation item: Project(200805331062) supported by the Research Fund for the Doctoral Program of Higher Education of China; Project(2010FJ4061) supported by the Science and Technology Program of Hunan Province, China
Received date: 2011-03-07; Accepted date: 2011-06-14
Corresponding author: ZHOU Hong-ming, Associate Professor, PhD; Tel: +86-731-88877173; E-mail: ipezhm@yahoo.com.cn