
Preparation and formation mechanism of Al2O3 nanoparticles by
reverse microemulsion
HUANG Ke-long(黄可龙), YIN Liang-guo(尹良果), LIU Su-qin(刘素琴), LI Chao-jian(李朝建)
School of Chemistry and Chemical Engineering, Central South University, Changsha 410083, China
Received 27 September 2006; accepted 22 December 2006
Abstract: Al2O3 nanoparticles were prepared by polyethylene glycol octylphenyl ether(Triton X-100)/n-butyl alcohol/cyclohexane/ water W/O reverse microemulsion. The proper calcination temperature was determined at 1 150 ℃ by thermal analysis of the precursor products. The structures and morphologies of Al2O3 nanoparticles were characterized by X-ray diffraction, transmission electron microscopy and UV-Vis spectra. The influences of mole ratio of water to surfactant on the morphologies and the sizes of the Al2O3 nanoparticles were studied. With the increase of surfactant content, the particles size becomes larger. The agglomeration of nanoparticles was solved successfully. And the formation mechanisms of Al2O3 nanoparticles in the reverse microemulsion were also discussed.
Key words: reverse microemulsion; Al2O3; nanoparticle; formation mechanism
1 Introduction
The synthesis of nanoparticles has been extensively investigated in the last two decades. Nano-sized materials exhibit novel and significant mechanical, electronic, magnetic and optical properties in comparison with their bulk counterparts[1-4]. Al2O3, because of its excellent capability shown in the mechanical, electronic, magnetic, chemistry and thermodynamic fields, can be considered as one of the high-function materials, and widely used in catalyst, fine ceramics, complex materials, fluorescent materials, waterish sensor and infrared- absorbing materials. Up to now, Al2O3 nanoparticles can be synthesized by many different methods, such as the co-precipitation, sol-gel[5], thermal decomposition[6]. But the descriptions concerning the preparation of Al2O3 nanoparticles by use of water-in-oil microemulsion have been limited[7].
The co-precipitation and sol-gel method are used to prepare metal oxide or composite metal oxide by chemical reaction. Their advantages lie in the fact of putting the homogeneous mixture of different ingredients into practice. But in their preparation period, an expensive alcoxide must be used as the primary metals and the treatment procedure for the samples is more complicated, which increases the complexity of preparing process and the costs of operation.
Thermal decomposition of organic compounds in an organic media is an available method for preparing inorganic material. But this method must be performed under a certain temperature and pressure, that is to say, an operation condition is somewhat critical.
Water-in-oil(W/O) microemulsions or reverse microemulsions technique is one of the most recognized methods due to its several advantages, for instance, soft chemistry, demanding no extreme pressure or temperature control, easy handling, and requiring no special or expensive equipment, and was invented as an effective process for preparing nanoparticles in 1980s[8]. W/O microemulsion is coexistence of an excess water phase and the surfactant molecules that aggregate in the oil phase in the form of reverse micelle. It is well known that these micelles can perform as nano-scaled reactors[9]. Once two microemulsions of that one contains the precursor and the other contains the precipitating agent are uniformly mixed, the reaction will occur in controlled manner in the micelles that have the size in order of nanometers, resulting in the formation of nanoparticle of controlled characteristics.
In this paper, Al2O3 nanoparticles were prepared in polyethylene glycol octylphenyl ether (Triton X-100)/ n-butyl alcohol/cyclohexane/water (W/O) reverse microemulsion. The morphological feature of the nanoparticles was investigated by TEM, and the influence of the calcination temperature on the size of particles was also discussed.
2 Experimental
2.1 Materials
The chemicals used were aluminum nitrate (Al(NO3)3·9H2O), ammonia (NH3·H2O), Triton X-100 (polyethylene glycol octylphenyl ether), n-butyl alcohol and cyclohexane. All chemicals were of analytical grade and used without further purification and the water used is deionized one.
2.2 Synthesis of Al2O3 nanoparticles
To prepare the microemulsion, cyclohexane, Triton X-100 and n-butyl alcohol were used as oil phase, surfactant and cosurfactant, respectively. Then the oil phase, surfactant and cosurfactant were mixed by magnetically stirring until the mixture became transparent, and the mole ratio of the surfactant to cosurfactant was 1?4. Divide the mixture into two parts, and the two different microemulsions were prepared as follows: the Al(NO3)3 aqueous was added into one of the parts and the mole ratio of water to surfactant(ωo) was respectively kept at 10, 15, 20, then the reverse microemulsion of Al(NO3)3 was obtained; the ammonia was added into the other part of mixture until the mixture became transparent, then the reverse microemulsion of ammonia was prepared. All experimental processes were under magnetic stirring.
The two reverse microemulsions were mixed and magnetically stirred until pH=8.5-9.0 at room temperature. Continue to stir for about 1 h and age for 24 h. The precipitates were repeatedly washed using ethanol by ultrasonic and followed by centrifugalization. The precursor powders were dried in microwave muffle at 60 ℃ for 1 h. Finally, the precursor was calcined at 1 150 ℃ for 2 h.
2.3 Characterization
Thermal analysis was carried out from 0 to 1 300 ℃ in atmosphere on SDT-Q600 V7.0 Build84 thermal analyzer. X-ray diffraction(XRD) was performed on an XD-98 X-ray diffractometer with graphite monochromatized Cu Kα radiation (l=0.154 178 nm), in a range from 10? to 80?. The UV-Vis spectra were recorded in a range form 190 to 1 100 nm using a UV- 3802 UV-Vis spectrophotometer. TEM was used to examine the morphology of the Al2O3 nanoparticles on a JEOL transmission electron microscope (model: JEM- 1230).
3 Results and discussion
3.1 Thermal analysis of precursor
In order to reveal the changes occurred during heat treatment of the precursor powder, TG—DTG and DTA analysis were carried out from 0 to 1 300 ℃ in atmosphere. According to the TG curve, the precursor shows rapid mass loss of about 22.5% from 20 to 200 ℃, DTA curve shows an endothermic peak at about 100 ℃, corresponding to the evaporation of the absorbed water. The mass loss slows down after 200 ℃, and DTA curve shows another endothermic peak at about 317 ℃, corresponding to the evaporation of the constitution water in the aluminum hydroxide and AlOOH. Two exothermic peaks appear at approximately 515 ℃ and 833 ℃ in the temperature range from 372 to 850 ℃, which might be associated with the conversion precursor of Al(OH)3→AlOOH→δ-Al2O3→θ-Al2O3[10]. According to the TG—DTG curve, the major part of mass loss seems to occur below 850 ℃, and that is to say, the metastable state Al2O3 is already formed before 850 ℃, the endothermic peak at 1 143 ℃ is corresponded to the transfer from θ-Al2O3 to α-Al2O3. Therefore, 1 150 ℃ is chosen as the proper calcination temperature.
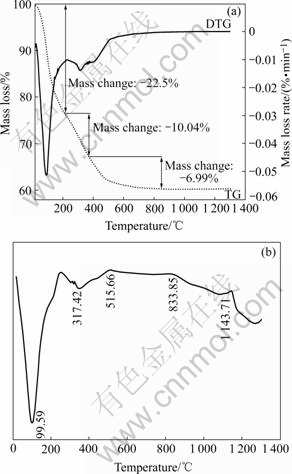
Fig. 1 TG—DTG (a) and DTA (b) curves of Al2O3 precursor
3.2 XRD analysis
A typical XRD plot is shown in Fig.2. The diffraction angle and relative intensity of the characteristic peak of the samples are well consistent with those of the standard JCPDS 10-0173. The sharp diffraction peaks in the pattern can be exactly indexed to a hexagonal structure of Al2O3, and the cell parameters are a=b=4.756 8 nm, c=13.019 nm, which mostly fit the standard cell parameters of a=b=4.758 5 nm, c=12.991 nm. No characteristic peaks of impurities or other precursor compounds are observed.
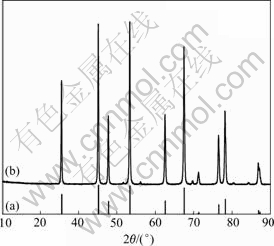
Fig.2 XRD spectra of standard JCPDS 10-0173 (a) and Al2O3 nanoparticles (b)
3.3 Reaction mechanism in microemulsion system
During the preparation of Al2O3 nanoparticles, the following reactions might occur:
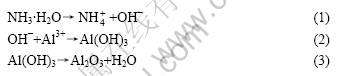
Reactions (1) and (2) take place in the microemulsion droplets. In the present case, microemulsion was used as the reaction media to prepare Al(OH)3 particles. The microdroplets in the microemulsion are in spherical shape with diameter from 10 to 100 nm and their sizes can be adjusted artificially. Due to the controllable water pool, reversed micelles are particularly favorable for the preparation of mono- disperse nanoparticles with various particle sizes. The general method for synthesizing nanoparticles using reverse micelles is schematically illustrated in Fig.3. This can be divided into three steps. The first step is the mixing of two reverse micelles D and E, and in this way, different reactants in different water nuclei exchange each other and the reaction takes place. Due to the coalescence of the reverse micelles, exchange of the materials in the water droplets occurs, which causes a reaction between the cores. Since the diameter of the water droplet is constant, nuclei in the different water cores cannot exchange each other. It is a precondition to accomplish the potential reaction that the reactants exchange via colliding between two water nuclei[11-12]. This is the second step. And in this step, instant dimmer G can be formed while the droplets collide each other. In the third step, the instant dimmer provides water channel for the two droplets to exchange reactants at this moment. The formation of instant dimmer changes the shape of surfactant membrane, so it has high energy and will separate in a very short time. Just during the uninterrupted combination and separation, the reaction takes place and then molecules engender. Several molecules aggregate as a nucleus that accelerates the reaction as a catalyst. More and more resultant molecules adhere on the nucleus to form a particle at last, and this is the last step.
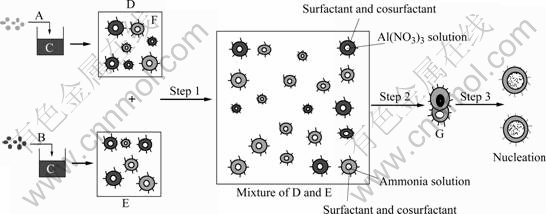
Fig. 3 Reaction mechanism in reverse microemulsion system (Step 1: Solublization and mixing of reactants; Step 2: Contact of different reactants; Step 3: Reaction, nucleation and growth of primary particles): A Al(NO3)3 solution; B Ammonia water;C Mixture of cyclohexane, surfactant and cosurfactant; F Cyclohexane; G Instant dimmer
Since the nucleus grows only in the microwater droplets of microemulsion and the droplets have a certain shape and size, the shape and size of resultant particles are determined by the water droplets. The resultant depositions almost consist of single spherical particle Al(OH)3. The surfactant and cosurfactant adhere to the surface of the nanoparticles served as a protective layer to prevent from collision and amalgamation of the droplets[13-17]. So ωo is a key parameter in determining the dimensions of microemulsion droplets. Diameter of the droplets increases with the increase of ωo, so the final particles size varies with ωo.
3.4 Effect of ωo
When the semiconductor particles are sufficiently small and the radius of the particle approaches that of the first excited-state orbital of the conduction band electrons, the quantum-size effects are observed[12-13]. With the reduction in particle size, the band gap of the semiconductor becomes large and there is a concomitant blue shift in the absorption spectrum. The UV-Vis spectra of Al2O3 nanoparticles are shown in Fig.4. The phenomenon of the blue shift is noticeable, with the decrease of ωo, which is an evidence of quantum confinement effect, indicating that with the increase of ωo the particles size becomes large. Fig.5 shows typical TEM images of the Al2O3 nanoparticles prepared at reverse microemulsion system with different mole ratios of water to surfactant ωo. It follows that the average size of the nanoparticles increases with increasing ωo. This is consistent with the UV-Vis spectra results.
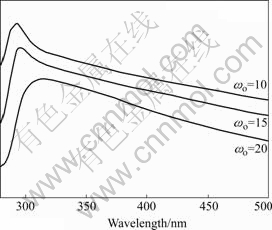
Fig.4 UV-Vis spectra at different mole ratios of water to surfactant (ωo)
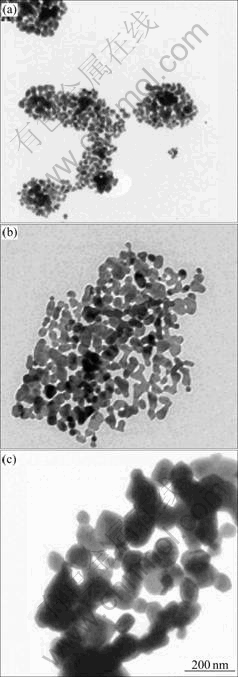
Fig.5 TEM images of α-Al2O3 at different mole ratios of water to surfactant: (a) ωo=10; (b) ωo=15; (c) ωo=20
4 Conclusions
1) Al2O3 nanoparticles were prepared by Triton X-100/n-butylalcohol/cyclohexane/water W/O reverse microemulsion.
2) Mole ratio of water to surfactant (ωo) plays an very important role in controlling the size of the Al2O3 nanoparticles. With increasing ωo, the particles size becomes larger.
3) Compared with other preparation methods, the surfactant and cosurfactant adhere to the surface of the nanoparticles serve as a protective layer to prevent from collision and amalgamation of the droplets. The reverse microemulsion has its own advantage and is subjected to wider use in preparing nanoparticles.
References
[1] HILINSKI E F, PATRICIA A L, WANG Y. A picosecond bleaching study of quantum-confined cadmium sulfide microcrystallites in a polymer film [J]. Journal of Chemical Physics, 1988, 89(6): 3435-3441.
[2] SIEGEL R W. Nanostructured materials—mind over matter [J]. Nanostructured Materials, 1993, 3(1/6): 1-18.
[3] BHARGAVA R N. Doped nanocrystalline materials: Physics and applications [J]. Journal of Luminescence, 1996, 70(1): 85-94.
[4] GOLDBURT E T, KULKARNI B, BHARGAVA R N, TAYLOR J, LIBERA M. Size dependent efficiency in Tb doped Y2O3 nanocrystalline phosphor [J]. Journal of Luminescence, 1997, 72/74: 190-192.
[5] HORIUCHI T, OSAKI T, SUGIYAMA T, SUZUKI K, MORI T. Maintenance of large surface area of alumina heated at elevated temperatures above 1 300 ℃ by preparing silica-containing pseudo- boehmite aerogel [J]. Journal of Non-Crystalline Solids, 2001, 291(3): 187-198.
[6] MA C C, ZHOU X X, XU X, ZHU T. Sythesis and thermal decomposition of ammonium aluminum carbonate hydroxide [J]. Materials Chemistry and Physics, 2001,72: 374-379.
[7] WANG X H, LU G Z, GUO Y, WANG Y S, GUO Y L. Preparation of high thermal-stable alumina by reverse microemulsion method [J]. Materials Chemistry and Physics, 2005, 90: 225-229.
[8] BOUTONNET M, KIZLING J, GIBERT P S. Preparation of monodisperse colloidal metal particles from microemulsions [J]. Colloids and Surfaces, 1982, 5(3): 209-225.
[9] KLABUNDE K J. Nanoscale Materials in Chemistry [M]. New York: Wiley-Interscience, 2001.
[10] LI J G, SUN X D. Synthesis and sintering behavior of a nanocrystalline α-Al2O3 powder [J]. Acta Mater, 2000, 48: 3103-3112.
[11] CLIFFORD Y T, LEE M H, WU Y C. Control of zirconia particle size by using two-emulsion precipitation technique [J]. Chemical Engineering Science, 2001, 56(7): 2389-2398.
[12] LEE M H, CLIFFORD Y T, LU C H. Synthesis of spherical zirconia by precipitation between two water/oil emulsions [J]. Journal of the European Ceramic Society, 1999, 19(15): 2593-2603.
[13] SUN L D, ZHANG Y X, ZHANG J, YAN C H, LIAO C S, LU Y Q. Fabrication of size controllable YVO4 nanoparticles via microemulsion-mediated synthetic process [J]. Solid State Communications, 2002, 124(1/2): 35-38.
[14] WELLER H, SCHMIDT H M, KOCH U, FOJTIK A, BARAL S, HENGLEIN A, KUNATH W, WEISS K, DIEMAN E. Onset of light absorption as a function of size of extremely small CdS particles [J]. Chemical Engineering Science, 2002, 124(6): 557-560.
[15] MEI Y, HAN Y B, LI Y, WANG W, NIE Z R. Measurement of microemulsion zone and preparation of monodispersed cerium oxide nanoparticles by W/O microemulsion method [J]. Materials Letters, 2006, 60(25/26): 3068-3072.
[16] HUANG Y, MA T, YANG J L, ZHANG L M, HE J T, LI H F. Preparation of spherical ultrafine zirconia powder in microemulsion system and its dispersibility [J]. Ceramics International, 2004, 30(5): 675-681.
[17] VIDAL V J, RIVAS J, LOPEA-OUINTELA M A. Synthesis of monodisperse maghemite nanoparticles by the microemulsion method [J]. Colloids and Surfaces A: Physicochemical and Engineering Aspects, 2006, 288(1/3): 44-51.
Foundation item: Project(2002AA323100) supported by the National High-Tech Research and Development Program of China
Corresponding author: LIU Su-qin; Tel: +86-731-8879850; E-mail: sqliu2003@126.com
(Edited by CHEN Wei-ping)