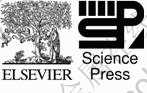
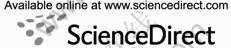
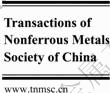
Trans. Nonferrous Met. Soc. China 22(2012) 1028-1034
Microstructural evolution of AZ91 magnesium alloy during extrusion and heat treatment
LI Jing-yuan1, XIE Jian-xin1, JIN Jun-bing2, WANG Zhi-xiang3
1. School of Materials Science and Engineering, University of Science and Technology Beijing, Beijing 100083, China;
2. China Aviation Planning and Construction Development Co., Ltd., Beijing 100120, China;
3. School of Materials Science and Engineering, Jiangxi University of Science and Technology,Ganzhou 341000, China
Received 10 February 2012; accepted 12 March 2012
Abstract: Microstructural evolution of AZ91 magnesium alloy was investigated during homogenizing annealing treatment, hot extrusion and ageing treatment, respectively. The results exhibited that both the divorced eutectic β-Mg17Al12 and the precipitated β-Mg17Al12 phases appeared in the as-cast alloy. The β-Mg17Al12 phase dissolved into α-Mg matrix mostly and the structure kept fine after the optimized homogenization treatment at 380 ℃ for 15 h. Dynamic recrystallization and consequent grain refinement occurred during extrusion. The banded β-Mg17Al12 precipitates paralleled to the extrusion direction were observed after ageing treatment. The banded precipitation should be attributed to the solidification segregation which was elongated during the subsequent extrusion. Furthermore, the effects of temperature, holding time of homogenization and ageing treatment, and extrusion processing parameters on the microstructural evolution of AZ91 alloy were also discussed in details according to the experimental results.
Key words: AZ91 alloy; microstructural evolution; Mg17Al12 phase; heat treatment; extrusion
1 Introduction
Magnesium alloy is gradually becoming important since it has low density, high specific strength, high dimensional stability, and is recyclable [1]. As the lightest material for structural applications, magnesium alloy is a potential candidate for replacing steel and aluminum alloy, especially in some automotive areas [2,3]. Therefore, researches and applications on magnesium alloys have surged in recent years [4,5].
Nowadays, most magnesium alloy products are manufactured by industrial die casting or semi-solid forming, while the plastic work processing is seldom employed because of the limited ductility in the hexagonal close-packed (HCP) structure [6]. At room temperature, magnesium and its alloys deform essentially by basal slip and twinning, which limit their formability. Consequently, thermomechanical processing is frequently carried out at high temperature [7,8]. It is generally considered that additional slip systems (prismatic and pyramidal) contribute significantly to deformation when the temperature is higher than 300 ℃, namely, when the associated critical resolved shear stresses are comparable [9]. Nevertheless, it has been also suggested that twinning may still be active even at high temperature [10].
Research on magnesium tends to focus on improving the plastic formability due to increased interest in application in the transportation industry for improving fuel efficiency through vehicle weight reduction [11,12]. HSIANG and KUO [13] investigated the mechanical properties of the hot extruded magnesium alloys AZ31 and AZ61 under the optimal processing conditions. YU e al [14] reported the stress—strain curve of AZ31 alloy obtained under various strain rates and temperatures, and established constitutive relationship of the alloy. NODA et al [15] investigated the transition of the deformation mechanism of AZ31 alloy during the high-temperature uniaxial tensile deformation and considered that the stable deformation behaviour was dominated by grain boundary sliding.
However, it is still largely unknown about the microstructural evolution of AZ91 magnesium alloy during the whole forming process, which includes previous homogenization, extrusion and subsequent ageing treatment [16,17]. Since the morphology of β-Mg17Al12 phase exerts a great influence on the ductility of Mg-Al alloys, the present study aims to explore the morphologies of β-Mg17Al12 obtained in the stated processes above.
2 Experimental
The AZ91 alloy, used in the present study, with composition of 8.4%Al, 0.88%Zn and 0.34%Mn, was supplied by General Research Institute for Nonferrous Metals, China. The alloy was provided in the form of 93 mm-diameter bars. The as-cast bars were cut to 30 mm in diameter and 45 mm in length so as to be extruded.
This study was performed in three experimental procedures which were homogenization, extrusion and ageing treatment, respectively. The homogenization followed by air cooling was carried out at 350, 380, 420 and 450 ℃ for 5, 10, 15 and 24 h, respectively, in a tubular resistance furnace. The optimized homogenization temperature was determined after analyzing the microstructures to be 380 ℃ and the time was 15 h [18]. The extrusion without lubricant was performed at 320, 350, 380 and 400 ℃ with extrusion ratio of 10 and 40, respectively, after being applied to homogenization treatment with the optimized parameter. The exit speed of extrusion was 1.6 m/min. An ageing treatment of the extruded bar was carried out at 200 ℃ for 2, 5, 10 and 15 h, respectively.
The microstructures of the specimens applied to heat treatment and deformation were observed by optical microscopy and scanning electron microscopy. The specimens were etched in an acetic glycol solution (20 mL acetic acid, 1 mL HNO3, 60 mL ethylene glycol and 20 mL water) before microstructure observation. The average grain sizes of the specimens were measured by the linear intercept method.
3 Results and discussion
3.1 Phases in as-cast AZ91 magnesium alloy
The Mg-Al binary equilibrium phase diagram indicates the various solid solubility of aluminum in magnesium matrix at various temperatures [19]. The maximum solid solubility of aluminum is w(Al)=12.7% at the eutectic temperature of 437 ℃, while the minimum solid solubility is about 2% at room temperature. Therefore, the microstructure of AZ91 alloy with aluminum composites of about 9% can be deduced to be a mixture structure of α-Mg solid solution matrix and precipitate of Al-Mg.
Furthermore, since the real cooling rate is impossible to be slow enough to keep the equilibrium solidification, the real solidification will deviate from the phase diagram. In other words, both the eutectic temperature and the critical hypoeutectic content of aluminum will become lower under real condition than those at equilibrium state. The real solidification can be described using the dashed line in the non-equilibrium phase diagram, as shown in Fig. 1. Based on the statement, eutectic reaction will occur at the end of solidification of AZ91 alloy although its content of aluminum is lower than 12.7%.
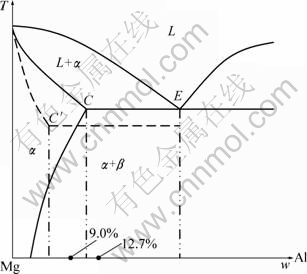
Fig. 1 Parts of Mg-Al binary non-equilibrium phase diagram
The as-cast microstructure with an average grain size of 100 μm is shown in Fig. 2. It can be seen that α-Mg grains are surrounded by coarse and reticular β-Mg17Al12 phase which is black in Fig. 2(a) but white in Fig. 2(b). The scanning electron micrograph shown in Fig. 2(b) exhibits four phases: primary α-Mg, divorced eutectic α-Mg, divorced eutectic β-Mg17Al12 and precipitate β-Mg17Al12. As stated above, the cooling rate is too high to keep aluminum homogeneous during solidification. So, the remaining liquid was quite rich in aluminum at the end of solidification. Then the liquid with aluminum content close to the eutectic composition, transformed to the divorced eutectic (α+β). In the further cooling procedure, β-Mg17Al12 precipitates into α-Mg matrix, especially in the divorced eutectic α-Mg matrix for its high aluminum content. The formation of the phases can be deduced as follows:
L → L + primary α → remaining L + primary α-Mg → divorced eutectic (α+β) + primary α-Mg → divorced eutectic (α+β) + precipitate β + primary α-Mg
3.2 Solution of β-Mg17Al12 during homogenization
Mg17Al12 phase is very brittle and its morphology, size, quantity and distribution have a great influence on the plasticity of Mg-Al alloys. The as-cast structure of α-Mg grains surrounded by coarse and reticularβ-Mg17Al12 phase leads to the limited ductility during deformation. In contrast, a structure of fine, homogenous and equiaxial β-Mg17Al12 distributing in α-Mg matrix improves the formability of magnesium alloys significantly. In order to obtain such a perfect morphology of β-Mg17Al12 phase, several homogenization experiments were carried out on AZ91 alloy in this investigation. Since the solid solution point of AZ91 is about 360 ℃ and the solidus point is 437 ℃, the experimental temperature was determined to be 350, 380 and 420 ℃, and holding time was 5, 10, 15 and 24 h, respectively.
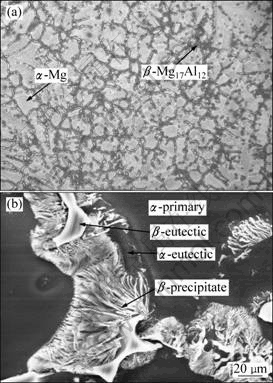
Fig. 2 Optical (a) and scanning electronic micrographs (b) of as-cast AZ91 alloy
The optical microstructures of the homogenized specimens are shown in Fig. 3, in which the light background corresponds to α-Mg matrix and the black regions or lines represent the remaining β-Mg17Al12 phase. It is obvious that the β-Mg17Al12 phase decreases as the temperature elevates and the holding time extends. As can be seen in Fig. 3(a1), no significant change is detected in the specimen after being treated at 350 ℃ for 5 h compared with the initial micrograph described in section 3.1. As the holding temperature extends at 350 ℃, the grains gradually transform from dendritic shape to equiaxial ones, which results from the solid solution of β-Mg17Al12 phase existing in interdendritic regions into α-Mg matrix. But until holding for 24 h, the β-Mg17Al12 phase keeps the reticular morphology, as shown in Fig. 3(a4).
When the thermal temperature is elevated to 380 ℃, β-Mg17Al12 phase dissolves more quickly than at 350 ℃, as seen in Figs. 3(b1-b4). Holding for 15 h at this temperature, the massive β-Mg17Al12 eliminates and changes to discontinuous linear existing at boundary of α-Mg grains. It suggests that most of the aluminum atoms dissolved into α-Mg matrix and the alloy became a supersaturated solid solution at room temperature. At this state, the grains with an average diameter of 130 μm still kept fine. However, when the holding time is extended to 24 h, the gains grow up to over 200 μm.
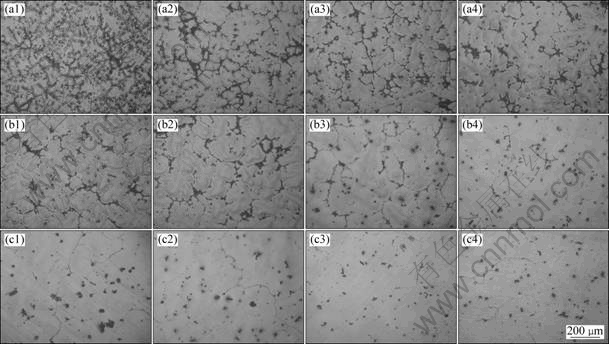
Fig. 3 Microstructures of AZ91 alloy homogenized at 350 ℃ (a1-a4), 380 ℃ (b1-b4) and 420 ℃ (c1-c4) for 5, 10, 15, 24 h, respectively
The third treatment temperature was 420 ℃, results of which are shown in Fig. 3(c1-c4). Due to the high temperature, almost all the β-Mg17Al12 phase dissolves after holding for only 5 h. However, the grain coarsening is very hard to avoid.
According to the results stated above, homogenization at 380 ℃ for 15 h exhibits the optimized microstructure morphology, the least β-Mg17Al12 phase and relatively fine structure, and high mechanical properties [20]. This treatment parameter was employed in the following extrusion test.
3.3 Grain refinement and incomplete recrystallization during extrusion
For magnesium alloys with HCP structure, the activation of slip systems greatly depends on the deformation temperature. Only the basal slip system is activated at room temperature; however, the slip systems (1011)[1120] and (1010)[1120] become activated when the temperature is beyond 300 ℃. In this study, extrusion tests of AZ91 magnesium alloy were carried out at 380 ℃ with various extrusion ratios after the optimized homogenization treatment at 380 ℃ for 15 h. The results demonstrate significant changes of microstructure. As shown in Fig. 4(a), although the extrusion ratio is only 10, grains have got extreme refinement, grain boundary became wary and clear, recrystallized grains have been visible along the initial grain boundary and the crystals had not been elongated. In addition, it can also be seen that the non-recrystallized grains mostly appear in the center of the extruded bar rather than at the edge because the deformation in the center was not sufficient for complete recrystallization. On the other hand, a finer and more equiaxial microstructure with an average grain size of 10 μm is obtained after extrusion at extrusion ratio of 40, as shown in Fig. 4(b).
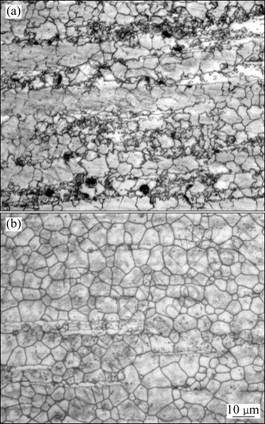
Fig. 4 Microstructures of AZ91 Mg alloys extruded at 380 ℃ with extrusion ratio of 10 (a) and 40 (b)
Figure 5 shows the microstructures of AZ91 alloys extruded at various temperatures with the same extrusion ratio of 40. A typical banded structure and elongated α-Mg grains with diameter of 5-10 μm are observed along the extrusion direction in the specimens extruded at 320 ℃ and 350 ℃. In addition, extremely fine recrystallized grains with diameter of about 1 μm appear at the initial grain boundary, as shown in Figs. 5(a) and (b). It should be attributed to incomplete recrystallization at low temperatures. When the extrusion temperature is elevated to 400 ℃, an equiaxial, homogeneous and completely recrystallized microstructure is obtained. However, the growth of new grain is observed and the average size of grain reaches 10 μm, as shown in Fig. 5(c).
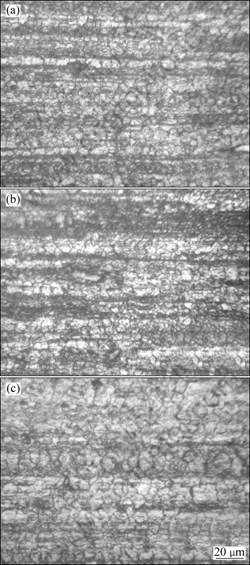
Fig. 5 Extruded microstructures of AZ91 alloys extruded at 320 ℃ (a), 350 ℃ (b), 400 ℃ (c) with extrusion ratio of 40
Many researchers reported that the severe shearing deformation, such as equal channel angular extrusion(ECAE) [20], high pressure torsion(HPT) [21], can lead to grain refinement in metal alloys. The results obtained in this investigation suggest that significant grain refinement was possible in magnesium alloys using the traditional forming method, extrusion. It attributes to the low stacking fault energy (SFE) of magnesium, which has a value of 50-78 mJ/m2 [22], compared with titanium (>300 mJ/m2), aluminum (200 mJ/m2) [23]. The SFE is a material property on a very small scale and it modifies the ability of a dislocation in a crystal to glide onto an intersecting slip plane. When the SFE is low, the mobility of dislocations in a material decreases. This means that the dynamic recrystallization is easy to occur during the hot working, even at a relatively low temperature and small deformation, eg., temperature of 320 ℃ and extrusion ratio of 10 in this investigation. Nevertheless, the recrystallization performed incompletely and a mixed crystal structure formed under the conditions of low temperature and small extrusion ratio. With elevating the working temperature or increasing the extrusion ratio, the fraction of recrystallized grains increased progressively. An equiaxial microstructure was obtained when the temperature and extrusion ratio reached 380 ℃ and 40, respectively. Consequently, the growth of the new grains occurred at the elevated temperature.
3.4 Banded precipitation during ageing treatment
After extrusion at 380 ℃ and extrusion ratio of 40 accompanied with air cooling, the specimens of AZ91 alloy were ageing treated at 200 ℃ for various time. Figure 6 shows the microstructural evolution of the specimens under the above conditions. Figure 6(a) shows that the block β-Mg17Al12 phase precipitates at grain boundary of AZ91 bar after ageing treatment for 2 h. In addition, the morphology is not homogeneous and some precipitation bands are observed. With increasing the holding time, the quantity of β-Mg17Al12 phases increases and they are parallel to each other gradually thereby a banded structure forms. Intense banded β-Mg17Al12 precipitates are observed after being treated at 200 ℃ for 15 h, as shown in Fig. 6(d).
The banded precipitation observed here should be attributed to the banded distributions of aluminum atoms, in other words, composition segregation remaining in the extruded bars is prior to the ageing treatment. The development of structure and precipitation can be deduced as follows. As stated in section 3.1, severe aluminum segregation and divorced eutectic, namely, aluminum element was rich at interdendritic while poor in the center of dendrite, occurred during solidification of AZ91 alloy. In this investigation, the as-cast AZ91 alloy was homogenizing annealing treated firstly. In this processing, the divorced eutectic β-Mg17Al12 phase dissolved into the α-Mg matrix and a supersaturated solid solution as obtained during the subsequent cooling. However, aluminum and other alloy elements were hard to homogenize wholly in the matrix because the diffusion of alloy elements is quite difficult in magnesium matrix. This means that the dendrite distribution of aluminum atoms is maintained in the homogenized billet, although this segregation is not obvious in the optical micrographs, as shown in Fig. 3. And then the interdendritic regions rich in aluminum, are elongated parallel to the deformation direction during the extrusion. In other words, a banded segregation of aluminum forms. And then the segregation regions become the nuclei of the second β-Mg17Al12 precipitation during the subsequent ageing treatment. Moreover, the growth of β-Mg17Al12 phase is along the bands rich in aluminum with extending the holding time. Thus, the intense banded β-Mg17Al12 phase forms in the α-Mg matrix.
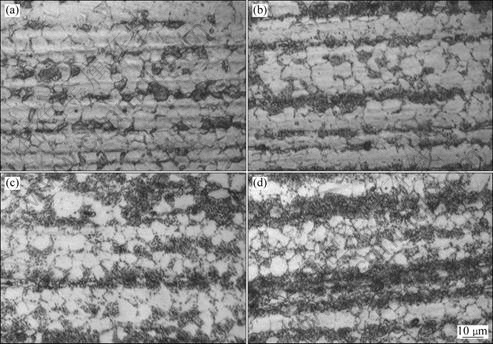
Fig. 6 Microstructures of AZ91 alloys after ageing treated at 200 ℃ for 2 h (a), 5 h (b), 10 h (c) and 15 h (d)
4 Conclusions
1) Both reticular divorced eutectic β-Mg17Al12 and precipitate β-Mg17Al12 are observed at the α-Mg grain boundary in the as-cast AZ91 alloy.
2) Homogenizing treatment may improve the microstructure and morphology of AZ91 alloy. A structure with average grain size of 100 μm, which is β-Mg17Al12 phase scattered at the α-Mg boundary, is obtained after the optimized homogenization at 380 ℃ for 15 h.
3) The recrystallization and refinement occur during extrusion. A mixed microstructure of remaining initial grains surrounded by fine recrystals is obtained when the extrusion temperature and ratio are 320 ℃, or 350 ℃ and 10, respectively. Homogenous, equiaxial and fine grains with size of 10 μm are obtained at 380 ℃, or 400 ℃ with extrusion ratio of 40.
4) Banded β-Mg17Al12 precipitates appear along the extrusion direction in α-Mg matrix during the ageing treatment. It can be deduced that the solidification segregation of aluminum element is elongated during extrusion, and the elongated segregation becomes the nuclei center and precipitates along growth direction of β-Mg17Al12. To lessen the segregation, divorced eutectic will be beneficial to eliminating banded structure and achieving a homogenous morphology.
References
[1] MAHALLAWY N A, SHOEIB M A, ABOUELENAIN M H. AZ91 magnesium alloys: Anodizing of using environmental friendly electrolytes [J]. Journal of Surface Engineered Materials and Advanced Technology, 2011, 1(2): 62-72.
[2] ZHAO Hong-liang, ZHOU Zhan-xia, LIU Xu-dong, GUAN Shao-kang. Influence of Mg3N2 powder on microstructures and mechanical properties of AZ31 Mg alloy [J]. Journal of Central South University of Technology, 2008, 15(4): 459-462.
[3] ELMORSY A W, KASEM A A. Tribological characteristics of deformed magnesium alloy AZ61 under dry conditions [J]. Journal of Tribology, 2011, 133(4): 603-610.
[4] YU Man-ye, LIN Ying. Finite element analysis of magnesium alloy automobile engine cylinder cover [J]. Machinery Design and Manufacture, 2010(10): 75-77. (in Chinese)
[5] XU Guan-qing, ZHAO Xiao-hong, FU Rong, GAO Hong-wei. Study on surface treatment technology of magnesium alloy auto parts [J]. Automobile Technology and Material, 2004(7): 57-59. (in Chinese)
[6] UEMATSU Y, TOKAJI K, FUJIWARA K, TOZAKI Y. Fatigue behaviour of cast magnesium alloy AZ91 microstructurally modified by friction stir processing [J]. Fatigue Fracture of Engineering Materials Structures, 2009, 32(7): 541-551.
[7] HARAI Y, KAI M, KANEKO K, HORITA Z. Microstructural and mechanical characteristics of an AZ61 magnesium alloy processed by high pressure torsion [J]. Materials Transactions, 2008, 49(1): 76-83.
[8] CIZEK L, GREGER M, PAWLICA L, DOBRZANSKI LA, TANSKI T. Study of selected properties of magnesium alloy AZ91 after heat treatment and forming [J]. Journal of Materials Processing Technology, 2004, 157-158: 466-471.
[9] AOYAMA K, FURUI M, IKENO S. Microstructure evolution in AZ31 magnesium alloy worked by torsion at warm temperature [J]. Materials Science Forum, 2010, 654-656: 727-730.
[10] HU Hong-jun, ZHANG Ding-fei, YANG Ming-bo, DENG Ming. Grain refinement in AZ31 magnesium alloy rod fabricated by extrusion-shearing severe plastic deformation process [J]. Transactions of Nonferrous Metals Society of China, 2011, 21(2): 243-249.
[11] HSIANG Su-hai, LIN Yi-wei, LAI Hong-wei. Optimization of hot extrusion process for AZ61 magnesium alloy carriers [J]. International Journal of Material Forming, 2011(4): 1-10.
[12] MARYAA M, HECTOR L G, VERMA R, TONG W. Microstructural effects of AZ31 magnesium alloy on its tensile deformation and failure behaviors [J]. Materials Science and Engineering A, 2006, 418: 341-356.
[13] HSIANG S H, KUO J L. An investigation on the hot extrusion process of magnesium alloy sheet [J]. Journal of Materials Processing Technology, 2003, 140: 6-12.
[14] YU Kun, CAI Zhi-yong, WANG Xiao-yan, SHI Ti, LI Wen-xian. Constitutive analysis of AZ31 magnesium alloy plate [J]. Journal of Central South University of Technology, 2010, 17(1): 7-12.
[15] NODA M, MORI H, FUNAMI K. Transition in deformation mechanism of AZ31 magnesium alloy during high-temperature tensile deformation [J]. Journal of Metallurgy, 2011(10): 1-10.
[16] KIM W C, NAM N D, KIM J G, LEE J I. Effect of strontium on corrosion properties of AZ91 magnesium alloy [J]. Electrochemical and Solid-State Letters, 2011, 14(11): C21-C24.
[17] RUAN Li-qun. Dynamic forming of AZ91 magnesium alloy using explosive energy [J]. Materials Science Forum, 2011, 673: 297-300.
[18] JIN Jun-bing, WANG Zhi-xue, LIU Xue-feng, XIE Jian-xin. Effect of homogenizing treatment on microstructures and properties of AZ91 alloy [J]. Acta Metallurgica Sinica, 2006, 42(10): 1014-1018. (in Chinese)
[19] DAHLE A K, LEE Y C, NAVE M D, SCHAFFER P L, STJOHN D H. Development of the as-cast microstructure in magnesium-aluminum alloys [J]. Journal of Light Metals, 2001, 1(1): 61-72.
[20] MOSS M, LAPOVOK R, BETTLES C J. The equal channel angular pressing of magnesium and magnesium alloy powders [J]. JOM Journal of the Minerals, Metals and Materials Society, 2007, 59(8): 54-57.
[21] SMIRNOVA N A, LEVIT V I, PILYUGIN V I. Evolution of structure of FCC single crystals during strong plastic deformation [J]. Physics of Metals and Metallography, 1986, 61(6): 127-134.
[22] ZHANG Ya, ZENG Xiao-qing, LU Chen, DING Wen-jiang. Deformation behavior and dynamic recrystallization of a Mg-Zn-Y-Zr alloy [J]. Materials Science Engineering A, 2006, 428: 91-97.
[23] WU X, TAO N, HONG Y, LIU G, XU B, LU J, LU K. Strain-induced grain refinement of cobalt during surface mechanical attrition treatment [J]. Acta Materialia, 2005, 53: 681-691.
挤压和热处理过程中AZ91镁合金的组织变化
李静媛1,谢建新1,金军兵2,王智祥3
1. 北京科技大学 材料科学与工程学院,北京 100083;
2. 中国航空规划建设发展有限公司,北京 100120;
3. 江西理工大学 材料科学与工程学院,赣州 341000
摘 要:对AZ91镁合金在均质化热处理、热挤压和时效处理中的组织演化规律进行研究。研究结果表明,在铸态组织中存在离异共晶和固态析出两种β-Mg17Al12相。在最佳的均质化处理(380 ℃,15 h)过程中,β-Mg17Al12相大部分溶解到α-Mg基体中,并且晶粒细小。在热挤压过程中发生动态再结晶以及由此产生的晶粒细化。而时效处理之后则出现平行于挤压方向的带状β-Mg17Al12相析出,这应该是由于在挤压过程中把原始铸造偏析挤扁拉长而造成的。另外,根据实验结果,详细地讨论了均质化和时效处理的温度、保温时间以及挤压工艺参数对AZ91镁合金的组织变化产生的影响。
关键词:AZ91镁合金;组织变化;Mg17Al12 相;热处理;挤压
(Edited by YANG Hua)
Foundation item: Project (2011A090200104) supported by Guangdong Science and Technology Special Projects, China
Corresponding author: LI Jing-yuan; Tel: +86-10-82376939; E-mail: jerranlee@yahoo.com.cn
DOI: 10.1016/S1003-6326(11)61279-X