
Separation efficiency of alumina particles in Al melt under high frequency magnetic field
GUO Qing-tao(郭庆涛)1, CAO Zhi-qiang(曹志强)2, ZHANG Zhong-tao(张忠涛)2,
LI Ting-ju(李廷举)2, JIN Jun-ze(金俊泽)2, HONG Chun-pyo1
1. Center for Computer-Aided Solidification Processing, Metallurgical System Engineering, Yonsei University,Seoul 120749, Korea;
2. School of Materials Science and Engineering, Dalian University of Technology, Dalian 116024, China
Received 4 January 2009; accepted 20 May 2009
Abstract: The effects of separation time and magnetic induction intensity on the separation efficiency of alumina particles with diameters varying from 30 to 200 μm in aluminum melt were investigated. The experimental results show that the particle- accumulated layer is formed in the periphery of the solidified specimen when the diameter of the separated molten metal, the magnetic induction intensity and the separation time are 10 mm, 0.04 T and 1 s, respectively. When the separation time is 2 s, the particle-accumulated layer can be observed obviously and the separation efficiency is about 80%. There are few alumina particles in the inner of the solidified specimen when the separation time is 3 s. The separation efficiency higher than 85% can be achieved when the separation time is longer than 3 s. When the magnetic induction intensity is 0.06 T, the visible particle-accumulated layer can be formed in 1 s and the separation efficiency is higher than 95%. The experimental results were compared with the calculated results at last.
Key words: Al melt; alumina particles; electromagnetic separation; high frequency magnetic field; separation efficiency
1 Introduction
The application of electromagnetic force to separate particles has been proposed by ALEMANY et al[1-2] in 1980. ASAI et al have measured the migration velocity of polystyrene particles in a sodium chloride aqueous solution, in which DC current and DC magnetic field were simultaneously imposed. They found that the direction of migration of the particles is opposite to that of the electromagnetic force and the migration velocity agrees well with values calculated from the theory of KOLIN and LEENOV[3-4]. The process of applying high frequency magnetic field to the separation of particles from melt was first developed by EI-KADDAH et al[5-6], and the maneuverability of applying the electromagnetic field induced in a coil on the particle separation was theoretically demonstrated by YAMAO and SASSA[7] and AFSHAR et al[8]. Compared with the other electromagnetic separation methods, high frequency magnetic separation shows great advantages. Firstly, there is no contamination on the molten metal due to without the electrodes immerged. Secondly, with high frequency magnetic field, the micro-sized particles can be removed with high intensity electromagnetic force. However, more accurate experimental results are necessary to demonstrate the relationship between the various parameters and the separation efficiency under high frequency magnetic field.
In this work, the separation efficiency of alumina particles with the diameter of 30-200 μm in the molten aluminum was examined experimentally. The experimental results were also compared with the calculated results on high frequency magnetic field separation to provide more accurate evidences for high frequency magnetic separation.
2 Experimental
The melt of commercial pure aluminum was superheated at 700 ℃ for 20 min. The alumina particles with different diameters varying from 30 to 200 μm acted as the simulative particles in the molten aluminum. In order to get the uniform distribution of the particles, the molten aluminum was stirred for 30 min intermittently.
Fig.1 shows a schematic diagram of the experimental apparatus used in the present study. The 9-turn induction coil is made up of copper tubes with 10 mm in diameter. The inner diameter of the induction coil is 66 mm and its length is 140 mm. The induction coil is powered by an IGBT-type electrical source power which is capable of producing the alternating electric current with a frequency of 20 kHz. The ceramic tubule with 10 mm in inner diameter is placed in the center of the induction coil. The temperature of the separated aluminum melt kept constant with the both function of forced air-cooling and joule heat.
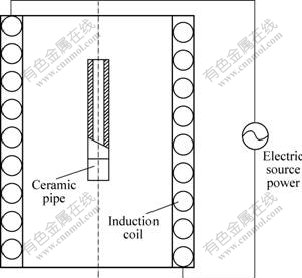
Fig.1 Schematic diagram of high frequency magnetic separation
In order to compare the experimental results, the cross section of the specimen was divided into five annular areas with the equal width notated by the numbers 1-5, as shown in Fig.2. Here the particle-accumulated layer (PAL) is defined as follows: in this layer the alumina particles get together and the number of alumina particles in the unit area is more than that in other areas due to the electromagnetic pinch force. The thickness of the PAL can be changed with the variety of the number of the alumina particles and the electromagnetic force.
In the experiments, the separation efficiency (ηexp) is calculated as
(1)
where Nsep is the number of the alumina particles in the PAL, and Ntotal is the total number of alumina particles in the cross section of the specimen.
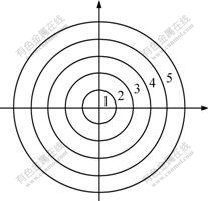
Fig.2 Schematic view of five areas for comparison of separation efficiency
3 Results
3.1 Effects of separation time
In the experiments, the molten aluminum which contained the alumina particles was poured into the ceramic tubule and the 0.04 T electromagnetic field was applied for 0, 1, 2, 3, 4, 5 and 10 s, respectively. Fig.3 shows the macrostructures of the specimens with various separation time. From Fig.3(a) it can be seen that without electromagnetic separation, the distribution of alumina particles in the specimen is almost uniform. When the molten metal is separated for 1 s under high frequency magnetic field, as shown in Fig.3(b), the particle- accumulated layer can be formed in the periphery of the specimen and the distribution of alumina particles is not uniform anymore. In the case of 2 s, there are few alumina particles in the area 2 but many in the area 1, as shown in Fig.3(c). In the areas 3 and 4, the number of alumina particles in the unit area is also lower than that in the area 1. In the area 5 of Fig.3(c), the particle-accumulated layer can be seen more significantly than that in Fig.3(b). Furthermore, when the separation time is longer than 3 s, there are almost no alumina particles in the center of the specimens as shown in Figs.3(d), (e) and (f). Fig.3(g) shows a macroscopic annular particle-accumulated layer in the case of the separation time of 10 s.
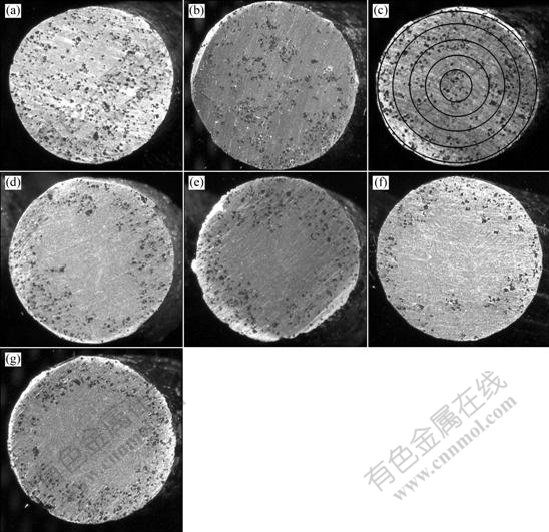
Fig.3 Effect of separation time on distribution of alumina particles in specimen (B0=0.04 T): (a) 0 s; (b) 1 s; (c) 2 s; (d) 3 s; (e) 4 s; (f) 5 s; (g) 10 s
Fig.4 shows the SEM micrographs of the specimen when the separation time is 3 s. In the area 1, there are no alumina particles bigger than 30 μm. However, in the area 5, many alumina particles get together due to the electromagnetic pinch force.
Fig.4 SEM micrographs of specimen in case of separation time of 3 s (B0=0.04 T): (a) Area 1; (b) Area 5
3.2 Effects of magnetic induction intensity
The magnetic induction intensity plays an important role in electromagnetic separation[9-12]. It affects not only the separation efficiency but also the flow in the molten metal[13-17]. Fig.5 displays the effect of magnetic induction intensity on the distribution of alumina particles in the specimen when the separation times are 1, 2 and 5 s, respectively. Fig.5(a) shows that there are many alumina particles in the center of the specimen when the magnetic induction intensity and separation time are 0.04 T and 1 s, respectively. However, when the magnetic induction intensity is 0.06 T, there are few alumina particles in the center of the specimen, as shown in Fig.5(d). Fig.5(b) shows that the visible PAL starts to emerge when the magnetic induction intensity and separation time are 0.04 T and 2 s, respectively. But there are still some alumina particles in the center of the specimen. When the magnetic induction intensity is increased to 0.06 T, as shown in Fig.5(e), there are almost no alumina particles in the center of the specimen. From Figs.5(c) and (f), it also can be seen that the separation efficiency is increased with the increase of the magnetic induction intensity.
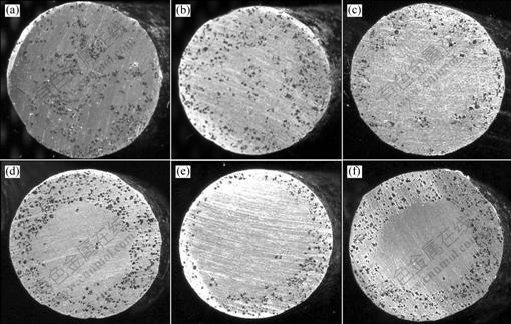
Fig.5 Distribution of alumina particles in specimen with various magnetic induction intensities and separation time: (a) 0.04 T, 1 s; (b) 0.04 T, 2 s; (c) 0.04 T, 5 s; (d) 0.06 T, 1 s; (e) 0.06, T 2 s; (f) 0.06 T, 5 s
4 Discussion
According to the previous report[18], the separation time (t) and the separation efficiency (ηcal) can be calculated as follows:
(2)
(3)
where a is the radius of the molten metal; upr is the velocity of the particle; R is the dimensionless number; S0 is the cross section area; and Se is the separated area. All material parameters used in the calculation are given in Table 1.
Table 1 Material parameters used in calculation (700℃)
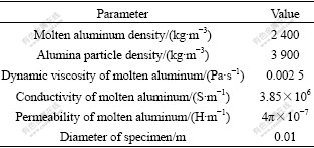
Fig.6 shows the comparison of the calculated and experimental separation efficiencies. From the calculated results, it can be seen that when the separation time, the size of the particles and the magnetic induction intensity are 1 s, 30 μm and 0.04 T, respectively, the separation efficiency is about 70%. When the separation time increases to 2 s, the separation efficiency reaches 80%. When the separation time is longer than 3 s, the separation efficiency is higher than 87%. The experimental results show that when the separation time is 1 s, the PAL can be formed but the separation efficiency is about 50%. When the separation time is 2 s, as shown in Fig.3(c), the separation efficiency is about 80%. Furthermore, when the separation time is longer than 3 s, there are few alumina particles in the center of the specimen and the separation efficiency is about 90%. Both the experimental and calculated results show that the separation efficiency is increased with the increase of separation time and can achieve more than 85% when the separation time is longer than 3 s. With the previous calculation[18], we know that the electromagnetic pinch force decreases with the increase of the distance between the particle position and the molten metal surface, and reaches zero in the center of the cylindrical specimen. Fig.7 shows the effects of the distance, between the particle position and the molten surface, and the magnetic induction intensity on the separation time. It can be seen that the separation time increases with the increase of the distance. When the distance between the particle position and the molten metal surface, the magnetic induction intensity and the size of the particles are 4.5 mm, 0.04 T and 30 μm, respectively, the separation time is about 11.8 s. Even when the magnetic induction intensity is increased to 0.06 T, it also needs more than 5 s for the particle migrating to the surface of the molten metal. It means that the particles with the distance longer than 4.5 mm cannot be separated in 5 s. While the experimental results show that when the separation time and the magnetic induction intensity are 5 s and 0.06 T, respectively, there are almost no alumina particles in the inner of the specimen. The reason for the difference is due to the flow in the molten metal induced by the nonuniform electromagnetic force.
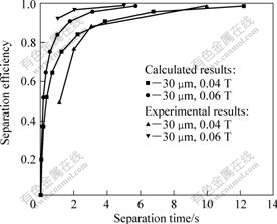
Fig.6 Comparison of calculated and experimental separation efficiencies
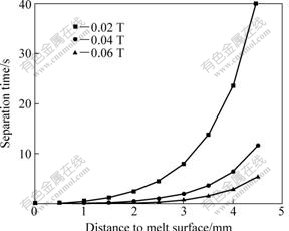
Fig.7 Effects of distance and magnetic induction intensity on separation time when sizes of particles and diameter of molten are 30 μm and 10 mm, respectively
The calculated results also show that the separation efficiency is increased with the increase of the magnetic induction intensity. When the size of the alumina particles, the separation time and the magnetic induction intensity are 30 μm, 1 s and 0.06 T, respectively, the separation efficiency is about 84%. The experimental result shows that the efficiency is higher than 95%. Both of them show that the separation efficiencies are higher than those when the magnetic induction intensity is 0.04 T. Thus, in order to get high separation efficiency, the magnetic induction intensity should be increased properly.
5 Conclusions
1) Both the experimental and calculated results show that the separation efficiency is increased with the increase of the separation time, and the separation efficiency higher than 85% can be achieved when the size of the particles, the separation time and the magnetic induction intensity are 30 μm, 3 s and 0.04 T, respectively.
2) The separation efficiency can be increased with the increase of the magnetic induction intensity. The visible particle-accumulated layer can be formed in 1 s and the separation efficiency is higher than 95% when the magnetic induction intensity is 0.06 T in the experiment. The separation efficiency at 0.06 T is higher than that at 0.04 T. It shows the same tendency with the calculated results.
References
[1] ALEMANY A, ARGOUS J P, BARBET J, WANES M, MOREAU R, POINSOT S. Electromagnetic device for the separation of inclusions contained in an electrically conductive fluid: EP0017588 (A1)[P]. 1980-10-15.
[2] MARTY P, ALEMANY A. Theoretical and experimental aspects of electro-magnetic separation//[C]Proceedings Symposium of IUTAM. London: The Metal Society, 1984: 245-259.
[3] KOLIN A. An electromagnetokinetics phenomenon involving migration of neutral particals [J]. Sciences, 1953, 117(6): 134-137.
[4] LEENOV D, KOLIN A. Theory of electromagnetophoresis(I): Magnetohydrodynamic forces experienced by spherical and symmetrically oriented cylindrical particles [J]. Journal of Chemical Physics, 1954, 22(4): 683-688.
[5] EI-KADDAH N. A comprehensive mathematical model of electromagnetic separation of inclusion in molten metals [J]. IEEE on Industrial Applications, 1988, 2: 1162-1165.
[6] EI-KADDAH N, PATEL A D, NATARAJAN T T. The electromagnetic filtration of molten aluminum using an induced current separator [J]. JOM, 1995, 47: 46-49.
[7] YAMAO F, SASSA K. Separation of inclusions in liquid metal using fixed alternating magnetic field [J]. Journal of the Iron and Steel Institute of Japan, 1997, 83: 30-35.
[8] AFSHAR M R, ABOUTALEBI M R, ISAC M, GUTHRIE R I L. Mathematical modeling of electromagnetic separation of inclusions from magnesium melt in a rectangular channel [J]. Materials Letters, 2007, 61: 2045-2049.
[9] LI K, SUN B D, LI T X, SHU D, DING W J, ZHOU Y H. Application of high frequency magnetic field to separate inclusion particles in aluminum melt [J]. Acta Metallurgica Sinica, 2001, 37(4): 405-410. (in Chinese)
[10] SHU D, SUN B D, LI K, LI T X, XU Z M, ZHOU Y H. Continuous separation of non-metallic inclusions from aluminum melt using alternating magnetic field [J]. Materials Letters, 2002, 55: 322-326.
[11] ZHANG B W, REN Z M, WU J X. Continuous electromagnetic separation of inclusion from aluminum melt using alternating current [J]. Trans Nonferrous Met Soc China, 2006, 16(3): 33-38.
[12] ASHOK S, RANJAN G, ISHWAR K P. Magnetic separation from superparamagnetic particle suspensions [J]. Journal of Magnetism and Magnetic Materials, 2009, doi: 10.1016/j.jmmm.2009.01.034.
[13] GUO Q T, LI T J, WANG T M. Effect of turbulent flow on electromagnetic elimination with high frequency magnetic field [J]. Trans Nonferrous Met Soc China, 2006, 16: 1141-1147.
[14] XU G J, JIN Y J, LIU G L. Discussion of the effects on electromagnetic separation efficiency of aluminum melts [J]. Foundry Technology, 2006, 27: 629-632.
[15] LIU H C, CAO Z Q, ZHANG H L. Fabrication of Al matrix gradient composites in high frequency magnetic field [J]. Special Casting & Nonferrous Alloys, 2008, 28: 242-244. (in Chinese)
[16] LIU H C, CAO Z Q, ZHANG H L. Fabrication of Al-Mg2Si-Al2O3 graded composite material under high frequency magnetic field [J]. Journal of Functional Materials, 2008, 39: 216-219. (in Chinese)
[17] SONG C J, XU Z M, LI J G. In-situ Al/Al3Ni functionally graded materials by electromagnetic separation method [J]. Materials Science and Engineering A, 2007, 445/446: 148-154.
[18] GUO Q T, JIN J Z, LI T J. Mathematical model of electromagnetic elimination in tubule with high frequency magnetic field [J]. Trans Nonferrous Met Soc China, 2006, 16(1): 47-51.
Foundation item: Projects(50474055, 50274018) supported by the National Natural Science Foundation of China; Project (20052176) supported by the Natural Science Foundation of Liaoning Province, China
Corresponding author: GUO Qing-tao; Tel: +82-10-4198-6449; E-mail:guoqing0660@sina.com
DOI: 10.1016/S1003-6326(09)60113-8
(Edited by YUAN Sai-qian)