Trans. Nonferrous Met. Soc. China 20(2010) s1029-s1032

SiC nanoparticles reinforced magnesium matrix composites fabricated by ultrasonic method
WANG Zhao-hui(王朝辉), WANG Xu-dong(王旭东), ZHAO Yu-xin(赵宇昕), DU Wen-bo(杜文博)
College of Materials Science and Engineering, Beijing University of Technology,
Beijing 100124, China
Received 13 May 2010; accepted 25 June 2010
Abstract: SiC nanoparticles reinforced magnesium matrix composites were fabricated by ultrasonic method. The AZ91 alloy and SiC nanoparticles with the average diameter of 50 nm were used as the matrix alloy and the reinforcement, respectively. The addition of nanoparticles was 0.1%, 0.3%, and 0.5% (mass fraction) of the composites. The results of microstructural evaluation and mechanical properties indicate that the nanoparticles can be dispersed into magnesium alloys efficiently and uniformly with the aid of ultrasonic vibration. As compared with the matrix alloys, the grains of composites were refined and the mechanical properties of composites were improved significantly. The SEM and DSC analyses show that the SiC nanoparticles can act as the heterogeneous nucleation of α-Mg. Also, the strengthening mechanism responsible for the composites reinforced with SiC nanoparticles was discussed.
Key words: magnesium matrix composites; AZ91 alloy; SiC nanoparticles; ultrasonic method
1 Introduction
Magnesium alloys are the lightest structural metallic materials, but the mechanical properties and creep resistant property of most common commercial magnesium alloys cannot meet the developing demands of the industries[1-5]. Hence, the magnesium matrix composites are attracted more attention because of their excellent properties, such as high specific modulus, high specific strength, low coefficient of thermal expansion and good wear resistance[6].
Recently, nano-sized ceramic particles, such as SiC, Al2O3, Y2O3 are used as reinforcement in magnesium matrix composites. The nanoparticles reinforced magnesium matrix composites show more excellent mechanical properties than those reinforced by micro-scale particles, but the key problem in the case of nano-sized particles is how to disperse them into the matrix alloys efficiently and uniformly. Among the efficient methods, there are ball-milling method[7-8], disintegrated melt deposition (DMD) method[9-10], ultrasonic dispersion method[11-12], semisolid process [13] and so on.
In the present work, AZ91 alloy and SiC nanoparticles were used as the matrix alloy and reinforcement, respectively. The reasons responsible for selecting SiC as reinforcement are due to its high hardness and strength and its heterogeneous nucleation effect in the molten magnesium alloys[6,14-15]. The composites were fabricated by ultrasonic method, the microstructure and mechanical properties of the composites were evaluated and the strengthening mechanism of the SiC nanoparticles was discussed.
2 Experimental
The commercial AZ91D magnesium alloys (8.94% Al, 0.70% Zn, 0.22% Mn, 0.02% Si, balance Mg) (mass fraction) were used as the matrix alloys. The beta-SiC particles with the average diameter of 50 nm were used as the reinforcing particles. The transmission electron micrograph (TEM) of the SiC nanoparticles is shown in Fig.1.
The clean and preheated magnesium ingots of about 600 g were put into an Al2O3 crucible. Then the magnesium alloy was heated to about 650 ?C in a resistance furnace. After the alloy was melted, the treated SiC nanoparticles were added into the melting magnesium alloy, and then the ultrasonic probe was dipped in the melt about 20 mm with the output power of 0.6 kW for about 30 min (the ultrasonic wave was 20.0 kHz with the maximum output of 2.0 kW). After the ultrasonic treating, the composites were cooled down to about 630 ?C and cast into the permanent mold preheated to 200 ?C. The whole experiments were carried out with the protection of 0.5%SF6+N2. The addition of SiC nanoparticles was 0.1%, 0.3% and 0.5% (mass fraction) of the composites, respectively. The contrastive samples without nanoparticles were also prepared with the same procedures.
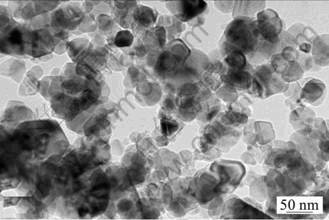
Fig.1 TEM micrograph of SiC nanoparticles
Specimens for micrograph observation were etched in acetic picral after being polished. The samples were observed by scanning electron microscopy (SEM) on a HITACH S3400N, and the high resolution transmission electron microscopy (HRTEM) with a JEOL 2010F after being ion milled. The DSC cooling curves of AZ91 and the composites with 0.5% SiC nanoparticles were obtained on a Netzsch STA 449C TG-DTA/DSC apparatus.
The tensile specimens with a gage diameter of 5 mm and a gage length of 25 mm were tested on the MTS 810 testing machine with the cross head speed of 1 mm/min at room temperature.
3 Results and discussion
Fig.2 shows the micrographs of AZ91 alloy and the composite with 0.5% SiC (mass fraction) nanoparticles. They are composed of primary α-Mg phase and β-Mg17Al12 phase. The α-Mg phase in the composite is finer and more uniform than that in the AZ91 alloy. The average size of α-Mg phase in the composite is about 60 μm, whereas it is about 90 μm in the AZ91 alloy. Besides grain refinement, the β-Mg17Al12 phase in the composite becomes discontinuous and fine in comparison with that in the AZ91 alloy.
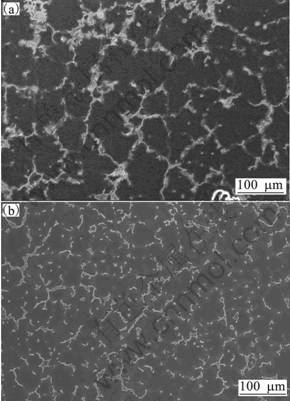
Fig.2 SEM micrographs of AZ91 (a) and composite with 0.5% SiC (b)
The difference in the microstructure was caused by the presence of SiC nanoparticles. The refinement of α-Mg phase in the composites should be due to the heterogeneous nucleation caused by the SiC nanoparticles. And the morphology change of the β-Mg17Al12 phase may be attributed to both the SiC nanoparticles and the ultrasonic vibration during the fabrication process. Therefore, the mechanical properties of the composite should be improved on the combination of the refined α-Mg phase and the β-Mg17Al12 phase.
In order to determine the heterogeneous nucleation caused by the SiC nanoparticles, the DSC cooling curves of AZ91 and the composite with 0.5% SiC nanoparticles were investigated. The DSC cooling curves of AZ91 alloys and the composite with 0.5% SiC nanoparticles are shown in Fig.3. The onset of solidification in AZ91 is 589.8 ℃, which is 4.6 ?C lower than that of the composites with 0.5% SiC nanoparticles. It is indicated that the SiC nanoparticles act as the heterogeneous nucleation of α-Mg phase in the magnesium alloy and the undercooling for solidification of α-Mg is reduced by the SiC nanoparticles.
It is because that the lattice constants of cubic β-SiC are a=b=c=0.4358 nm, α=β=γ=90°, the lattice mismatch between (111) crystal face of β-SiC and (0001) crystal face of α-Mg is only 3.96%. So, the SiC nanoparticles can act as the heterogeneous nucleation of α-Mg[15].
The distribution of SiC nanoparticles in the composite with 0.5% SiC nanoparticles is shown in Fig4. Besides few microclusters of SiC nanoparticles, the nanoparticles were dispersed well in the composite. It is shown that the ultrasonic method is an effective process for dispersing the nanoparticles in the magnesium alloys. It was reported that the ultrasonic cavitation in the molten melt could produce transient micro spots at temperature of about 5 000 ?C, pressure of above 100 MPa and heating and cooling rate about 107 K/s[16]. So, the nanoparticles clusters could be broken and the nanoparticles were dispersed well in the molten magnesium alloy. The well dispersed nanoparticles can both refine the microstructure of the magnesium alloys and act as the reinforcement of the composite effectively.
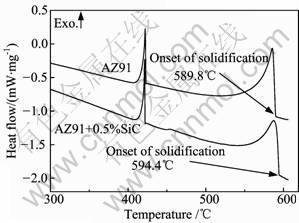
Fig.3 DSC cooling curves of AZ91 and composite with 0.5% SiC at cooling rate of 10 K/min
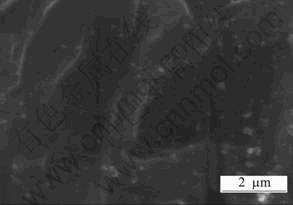
Fig.4 SEM micrograph of composites with 0.5% SiC
Fig.5(a) shows the HRTEM micrograph of the interface between a SiC nanoparticle and the magnesium matrix. The EDS spectrum of the interface is shown in Fig.5(b). The peaks of Si and C indicate the presence of SiC and the peaks of Mg, Al and Zn are attributed to the matrix of AZ91 alloy. According to Figs.5(a) and (b), it can be concluded that the SiC nanoparticle bonded well with the magnesium matrix without the intermediate phase or cracks between them. Considering the size of SiC in the present research is in the range of nanoscale, the ultrasonic method is an effective and viable way for fabricating the nanoparticles reinforced magnesium matrix composites.
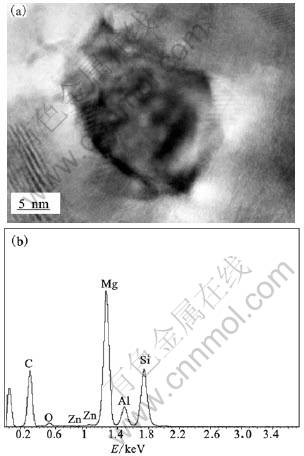
Fig.5 HRTEM micrograph (a) and EDS spectrum (b) of SiC nanoparticle in composite with 0.5% SiC
However, there are some “microclusters” of SiC nanoparticles occurred in the present research, which are shown in Fig.6. These microclusters consist of dozens of single nanoparticles. Most of these nanoparticles are not connected directly with each other. In fact, the microclusters are the mixture of nanoparticles and the magnesium alloy, and the most nanoparticles are separated by the magnesium alloy. This means that the microclusters of nanoparticles are unstable and possible to be broken and dispersed if the ultrasonic power is enough.
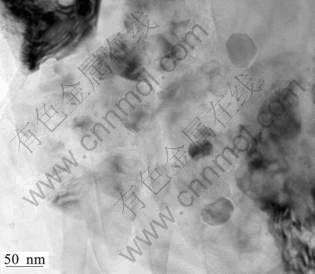
Fig.6 HRTEM micrograph of microcluster of SiC nanoparticles in composites
The mechanical properties of AZ91 and the composites are shown in Fig.7. The yield strength (YS), ultimate tensile strength (UTS) and elongation of AZ91 alloy are only 104 MPa, 174 MPa and 3.6%, respectively. With the addition of SiC nanoparticles, the YS, UTS and elongation of the composites have been increased significantly. The YS, UTS and elongation of the composite with 0.5% SiC nanoparticles are 124 MPa, 216 MPa and 6.6%, respectively. Compared with those of the matrix alloy, the YS, UTS and elongation of the composite with 0.5% SiC nanoparticles are improved by 19%, 24% and 83%, respectively.
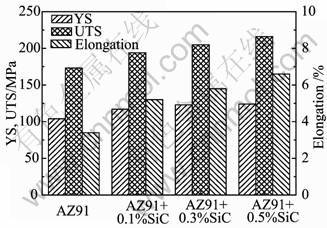
Fig.7 Mechanical properties of AZ91 and composites with 0.1%, 0.3% and 0.5% SiC
The improvement in mechanical properties of SiC nanoparticles reinforced composites is attributed to the grain refinement and the strengthening effect of SiC nanoparticles. The strengthening of the nanoparticles comes from the Orowan strengthening effects, enhanced dislocation density and loading bearing effects during the deforming course of the composites. Because the nanoparticle size in the present work is about 50 nm, the Orowan strengthening mechanism works more significantly than the micro-sized ceramic particles.
4 Conclusions
1) The ultrasonic method is an effective and viable process for dispersing the SiC nanoparticles in the magnesium alloy.
2) Because of the presence of SiC nanoparticles, the α-Mg phase is refined and the morphology of β-Mg17Al12 phase changes to discontinuous and fine. Microstructure and DSC analyses show that the β-SiC can act as the heterogeneous nucleation of α-Mg.
3) The improvement of mechanical properties of composites is attributed to the grain refinement and the strengthening effects caused by the SiC nanoparticles.
References
[1] Mordike B L, Ebert T. Magnesium: Properties—applications —potential [J]. Materials Science and Engineering A, 2001, 302: 37-45.
[2] Friendrich H, Schumann S. Research for a “new age of magnesium” in the automotive industry [J]. Journal of Materials Processing Technology, 2001, 117: 276-281.
[3] Schumann S. The paths and strategies for increased magnesium applications in vehicles [J]. Materials Science Forum, 2005, 488/489: 1-8.
[4] SHI C X, KE J, KAHN R W. Materials science and technology [M]. Beijing: Science Press, 1999. (in Chinese)
[5] LI N Y. Magnesium advances and applications in north America automotive industry [J]. Materials Science Forum, 2005, 488/489: 931-940.
[6] LUO A. Processing, microstructure, and mechanical behavior of cast magnesium metal matrix composites [J]. Metallurgical and Materials Transactions A, 1995, 26: 2445-2455.
[7] Ferkel H, Mordike B L. Magnesium strengthened by SiC nanoparticles [J]. Materials Science and Engineering A, 2001, 298: 193-199.
[8] Lukac P, Trojanova Z. Magnesium-based nanocomposites [J]. International Journal of Materials and Product Technology, 2005, 23(1/2): 121-137.
[9] Goh C S, WEI J, Lee L C, Gupta M. Properties and deformation behaviour of Mg-Y2O3 nanocomposites [J]. Acta Materialia, 2007, 55(15): 5115-5121.
[10] Nguyen Q B, Gupta M, Srivatsan T S. On the role of nano-alumina particulate reinforcements in enhancing the oxidation resistance of magnesium alloy AZ31B [J]. Materials Science and Engineering A, 2009, 500: 233-237.
[11] Cao G, Choi H, Oportus J, Konishi H, Li X. Study on tensile properties and microstructure of cast AZ91D/AlN nanocomposites [J]. Materials Science and Engineering A, 2008, 494: 127-131.
[12] CAO G, Konishi H, LI X. Mechanical properties and microstructure of SiC-reinforced Mg-(2,4)Al-1Si nanocomposites fabricated by ultrasonic cavitation based solidification processing [J]. Materials Science and Engineering A, 2008, 486: 357-362.
[13] Wang Z H, Kang Y L, Zhao H J, Xu Y. SiC nanoparticles reinforced magnesium alloys by semisolid process [J]. Solid State Phenomena, 2006, 116/117: 163-166.
[14] Gunther R, Hartig C, Bormann R. Grain refinement of AZ31 by (SiC)P: Theoretical calculation and experiment [J]. Acta Materialia, 2006, 54: 5591-5597
[15] LUO A. Heterogeneous nucleation and grain refinement in cast Mg(AZ91)/SiCp metal matrix composites [J]. Canadian Metallurgical Quarterly, 1996, 35(4): 375-383.
[16] ESKIN G I. Cavitation mechanism of ultrasonic melt degassing [J]. Ultrasonics Sonochemistry, 1995, 2(2): s137-s141.
(Edited by YUAN Sai-qian)
Foundation item: Project(2007CB613706) supported by the National Basic Research Program of China; Project(00900054R4001) supported by Innovation Project for Talents of BJUT; Project(00900054K4004) supported by the Science Foundation for Youths of BJUT.
Corresponding author: WANG Zhao-hui; Tel: +86-10-67392423; E-mail: wangzhaohui@bjut.edu.cn