
Microstructure and thermal properties of recyclable Sip/1199Al composites
XIU Zi-yang(修子扬), CHEN Guo-qing(陈国钦), YANG Wen-shu(杨文澍),
SONG Mei-hui(宋美慧), WU Gao-hui(武高辉)
School of Materials Science and Engineering, Harbin Institute of Technology, Harbin 150001, China
Received 10 August 2009; accepted 15 September 2009
Abstract: Recyclable Sip/1199Al composites with high volume fraction of Si particles were fabricated by squeeze-casting method. The microstructure was observed and the thermal properties were tested and calculated by theoretical models. Sip/1199Al composites are all dense and macroscopically homogeneous without any particle clustering. The interface of Sip/1199Al is clean, smooth and free from any interfacial reaction products. Sip/1199Al composites have high thermal diffusivity (65.083 mm2/s) and thermal conductivity (168.211 W/(m? ℃)). The specific heat capacity of Sip/1199Al composites at constant pressure increases while the thermal diffusivity and thermal conductivity decrease with increasing temperature. Annealing treatment could improve the thermal properties. The results of Maxwell model and P.G. model are higher than those of experiment.
Key words: Sip/Al composite; interface; thermal conductivity
1 Introduction
With the rapid development of technology, the sole metal material cannot meet the demands of aviation and aerospace industry[1]. Fortunately, metal matrix composites have been examined and developed for the applications[2]. Especially, particulate reinforced aluminum matrix composites, which have low and designable thermal expansion property, excellent thermal conductivity and low density, have obtained extensive application in the aviation field[3-4]. Recently, the Sip/Al composites have been developed with low expansion ((7.4-9.0)×10-6 ℃-1), high thermal conductivity (100-180 W/(m?℃)), low density (2.4-2.6 g/cm3), excellent machining property and recyclability, which have received growing attention in the development of metal matrix composites[5-7].
In this work, high content Si particles (φp=65%) with the average diameter of 10 μm were introduced to reinforce 1199Al alloy by squeeze-casting method. The microstructure was observed, and the thermal properties were tested and calculated by theoretical models.
2 Experimental
The reinforcements used in this work were Si particles with average diameter of 10 μm, and the reinforcement volume fraction was 65%. The composites were fabricated by squeeze-casting method. And the specimens were divided into as-cast and as-annealed states. The composites were annealed at 340 ℃ for 3 h and then furnace cooled. OLYMPUS PMG3 optical microscope, transmission electron microscope (TEM) and high resolution transmission electron microscope (HRTEM) were used to examine the microstructure of Sip/1199Al composites. The thermal conductivity measurement was performed on JK2 Thermal Diffusivity Analysis apparatus made in Germany. The specimen size was d12.7 mm×3 mm, and both the ends of specimen were polished with sand paper. The tested temperatures were from 20 ℃ to 500 ℃ with a heating rate of 5 ℃/min.
3 Results and discussion
3.1 Microstructure
The representative morphology of the as-cast Sip/1199Al composite is shown in Fig.1(a). The Si particles of irregular shape with edges distribute uniformly without any particle clustering, and no apparent porosity or significant casting defects are observed in the composites. A dense microstructure could improve its thermal conductivity, dimensional stability as well as strength, which leads to an improvement in service life[8]. TEM observation reveals high density of dislocations in Al matrix around Si particles, as shown in Fig.1(b). Generally, high density of dislocations are always found in aluminium composites reinforced with micron particles because of large thermal mismatch stress generated by coefficient of thermal expansion (CTE) difference between the ceramic particles and matrix[9-10].
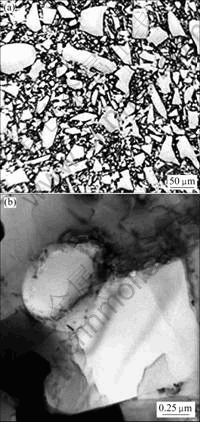
Fig.1 Microstructures of as-cast Sip/1199Al composite: (a) Optical morphology; (b) TEM image
A large amount of interfaces are introduced into composite, and these interfaces and the existing of interface effect are the important factors which can affect the properties of composite. Therefore, interfaces are always the focus of research in composite[11-12]. Fig.2 illustrates the typical Si/Al interfaces in annealed Sip/1199Al composite. It is obvious that the Si/Al interfaces are clean, smooth and free from interfacial reaction products.
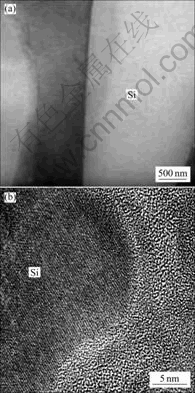
Fig.2 Si/Al interfaces in annealed Sip/1199Al composite: (a) TEM image; (b) HRTEM image
There are two ways for heat transfer in Sip/1199Al composite: free electron in Al matrix and phonon in Si particles. Both of their movement would be scattered by interfaces. Therefore, heat conduction in Sip/1199Al composite is dependent on Al matrix, Si particles and their interfaces[13]. Fortunately, the Si/Al interfaces in this work are clean and smooth, which are beneficial to the thermal properties of Sip/1199Al composite.
3.2 Thermal properties of Sip/1199Al composites
3.2.1 Specific heat capacity at constant pressure
Fig.3 shows the specific heat capacity at constant pressure of as-cast and annealed Sip/1199Al composites under different temperatures. It can be seen from Fig.3 that the specific heat capacity at constant pressure increases with temperature increasing for both as-cast and annealed Sip/1199Al composites.
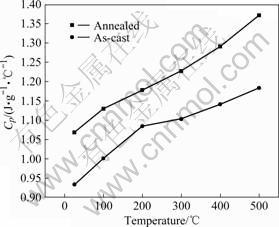
Fig.3 Specific heat capacity at constant pressure of as-cast and annealed Sip/1199Al composites under different temperatures
The known Debye law is as follows:
(1)
where CV is the specific heat capacity at constant volume, N the Avogadro constant, k the Boltzmann constant, T the absolute temperature, θD the Debye temperature. According to Eq.(1), at a lower temperature, CV is in direct proportion to T3. When the temperature is higher than Debye temperature, the CV tends to the constant 3R(R is the gas constant).
The relation between specific heat capacity at constant pressure Cp and specific heat capacity at constant volume CV is shown as:
(2)
where α is the bulk expansion coefficient, β is the compressive coefficient at constant temperature, and V is the atom volume.
It can be seen from Eq.(2) that the specific heat capacity at constant pressure increases with temperature increasing, indicating the thermal reservation capacity is increased with temperature increasing.
3.2.2 Thermal diffusivity and thermal conductivity
The thermal conductivity λ of composite is calculated by
λ=k?Cp?ρ (3)
where k is the thermal diffusivity, ρ is the density, Cp is the specific heat capacity at constant pressure.
Fig.4 shows the thermal diffusivity and thermal conductivity of as-cast and annealed Sip/1199Al composites.
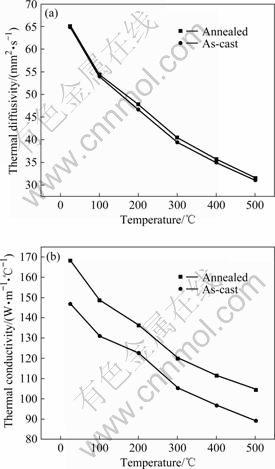
Fig.4 Variations of thermal conductivity properties of Sip/1199Al composites with temperature: (a) Thermal diffusivity; (b) Thermal conductivity
It can be seen that Sip/1199Al composites have high thermal diffusivity and thermal conductivity, which are65.083 mm2/s and 168.211 W/(m?℃) at room temperature, respectively. However, because the thermal properties of Si(thermal diffusivity and thermal conductivity are 89.2 mm2/s and 149 W/(m?℃), respectively) are far less than Al(thermal diffusivity and thermal conductivity are 589.9 mm2/s and 235.2 W/(m?℃), respectively), the addition of Si phase into Al would decrease the composite thermal conductivity properties. Moreover, Si/Al interfaces would also decrease the thermal conductivity properties [14]. Furthermore, annealing treatment could lessen the residual thermal stress, eliminate dislocations and lattice distortions in Al matrix maximally, which can reduce the free electron scattering in Al matrix and lead to improvement in thermal conductivity properties of Sip/1199Al composite[15].
3.2.3 Theoretical calculation of thermal conductivity
One significant advantage of composites is designable. The accurate prediction of performance of composites before fabrication or control of component to meet performance requirement, which would shorten cycle of design and fabrication of composites, attracts researchers on composite. However, accurate prediction must be based on the accurate theoretical foundation. The main theoretical prediction models for the thermal conductivity of composite include Maxwell model[16] and P.G. model[17]:
1) Maxwell model considered the effect of matrix conduction, particle conduction and particle fraction while simplified it as spherical particle, and it can be expressed as[16]:
(4)
where Kcom, Km, Kp are referred to thermal conductivities of composite, matrix and particle, respectively, and φp is the particle volume fraction.
2) P.G. KLEMENS[17] derived the relationship among composites’ thermal conductivity, matrix thermal conductivity, particle thermal conductivity and volume fraction as follows:

(5)
The models mentioned above were adopted to calculate the thermal conductivity of Sip/1199Al composites, and the theoretical and experimental values are listed in Table 1.
Table 1 Theoretical and experimental thermal conductivities of Sip/1199Al composites (W/(m?℃))
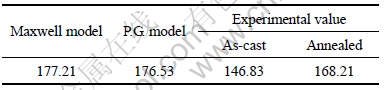
It can be seen that the thermal conductivities of both Maxwell model and P.G. model are higher than the experimental results because they consider the particles as spherical approximately, whereas in fact the particle shape is irregular geometric polyhedrons. Furthermore, they ignore the effects of particle size, stacking mode and interface thermal resistance on the thermal conductivity.
4 Conclusions
1) Sip/1199Al composites are all dense and macroscopically homogeneous without any particle clustering, and no apparent porosity or significant casting defects are observed. Si/Al interfaces are clean, smooth without any interfacial reaction products.
2) Sip/1199Al composites have high thermal diffusivity (65.083 mm2/s) and thermal conductivity (168.211 W/(m?℃)). The specific heat capacity at constant pressure of Sip/1199Al composites increases while the thermal diffusivity and thermal conductivity decrease with increasing temperature. Annealing treatment could improve thermal conductivity properties of Sip/1199Al composite.
3) The thermal conductivities by Maxwell model and P.G. model are higher than the experimental results.
References
[1] ZWEBEN C. Advances in composite materials for thermal management in electronic packaging [J]. Journal of the Minerals, Metals and Materials Society, 1998, 50(6): 47-51.
[2] ROSSO M. Ceramic and metal matrix composites: Routes and properties [J]. Journal of Materials Processing Technology, 2006, 175(1/3): 364-375.
[3] BAURI R, SURAPPA M K. Damping behavior of Al-Li-SiCp composites processed by stir casting technique [J]. Metallurgical and Materials Transactions A, 2005, 36(3): 667-673.
[4] RAMU G, BAURI R. Effect of equal channel angular pressing (ECAP) on microstructure and properties of Al-SiCp composites [J]. Materials and Design, 2009, 30(9): 3554-3559.
[5] CHIEN C W, LEE S L, LIN J C, JAHN M T. Effects of Sip size and volume fraction on properties of Al/Sip composites [J]. Materials Letters, 2002, 52(4/5): 334-341.
[6] FENG H K, YU S K, LI Y L, GONG L Y. Microstructure and properties of Sip/Al-Si surface composites prepared by ultrasonic method [J]. Materials and Design, 2009, 30(7): 2420-2424.
[7] XIU Zi-yang, WU Gao-hui, SONG Mei-hui, ZHU De-zhi. Thermo-physical properties of reproducible Sip/Al composites [J]. Trans Nonferrous Met Soc China, 2006, 16(s3): 1455-1459.
[8] ROMERO J C, WANG L, ARSENAULT R J. Interfacial structure of a SiC/Al composite [J]. Materials Science and Engineering A, 1996, 212(1): 1-5.
[9] HONG S K, WON C W, SHIN D H. Interfacial microchemistry and microstruture of Al-Mg-Si alloy matrix composites reinforced with Al2O3 particulates [J]. Scripta Materialia, 1997, 36(8): 883-889.
[10] ZHANG Q, XIU Z Y, SONG M H, WU G H. Microstructure and properties of a 70vol.% SiCp/Al-12Si composite for electronic packaging [J]. Materials Science Forum, 2005, 475/479: 881-884.
[11] INEM B, POLLARD G. Interface structure and fractography of a magnesium-alloy, metal-matrix composite reinforced with SiC particles [J]. Journal of Materials Science, 1993, 28(16): 4427-4434.
[12] GORGA R E, LAU K K S, GLEASON K K, COHEN R E. The importance of interfacial design at the carbon nanotube/polymer composite interface [J]. Journal of Applied Polymer Science, 2006, 102(2): 1413-1418.
[13] XIU Zi-yang, WU Gao-hui, ZHANG Qiang, SONG Mei-hui, TIAN Shou-fu. Microstructure and electric properties of Sip/Al composites for electronic packaging applications [J]. Trans Nonferrous Met Soc China, 2007, 17(sl): 1034-1038.
[14] MOLINA J M, NARCISO J, WEBER L. Thermal conductivity of Al-SiC composites with monomodal and bimodal particle size distribution [J]. Materials Science and Engineering A, 2008, 480(1/2): 483-488.
[15] XIE H Q. Thermal and electrical transport properties of a self-organized carbon nanotube pellet [J]. Journal of Materials Science, 2007, 42(10): 3695-3698.
[16] YOSHIDA K, MORIGAMI H. Thermal properties of diamond/ copper composite material [J]. Microelectronics Reliability, 2004, 44(2): 303-308.
[17] KLEMENS P G. The thermal conductivity of dielectric solids at low temperatures (Theoretical) [J]. Proceedings of the Royal Society of London Series A, 1951, 208(1092): 108-133.
Foundation item: Project(HITQNJS.2008.057) supported by Development Program for Outstanding Young Teachers in Harbin Institute of Technology, China
Corresponding author: XIU Zi-yang; Tel: +86-451-86402373-5056; E-mail: xiuzy@hit.edu.cn
DOI: 10.1016/S1003-6326(09)60047-9
(Edited by YUAN Sai-qian)