
Effect of yttrium on microstructures and mechanical properties of
hot rolled AZ61 wrought magnesium alloy
FANG Xi-ya(方西亚), YI Dan-qing(易丹青), WANG Bin(王 斌), LUO Wen-hai(罗文海), GU Wei(顾 威)
School of Materials Science and Engineering, Central South University, Changsha 410083, China
Received 23 December 2005; accepted 29 April 2006
Abstract: The microstructures and mechanical properties of the rolled AZ61 alloys containing different contents of Y (0, 0.5, 0.9, 1.4%Y respectively) were studied. Phase analysis was performed by X-ray diffraction(XRD). Microstructures of experimental materials were observed by optical microscope(OM) and scanning electron microscope(SEM) equipped with energy dispersive spectrometer(EDS). The results show that the alloys with variable Y contents all contain a second-phase Al2Y. The amount of Al2Y increases with the increasing of Y content while that of Mg17Al12 decreases. Moreover, Y refines the microstructures of as-cast and rolled alloys. The finest average grain size is obtained in the alloy containing 0.9%Y with the best mechanical properties. When the Y content is up to 1.4%, Al2Y phase in the alloy coarsens, which leads to the drop of tensile strength.
Key words: AZ61 alloy; rare earth; yttrium; hot rolling; microstructure; mechanical properties
1 Introduction
Magnesium alloys are attractive materials due to their good specific properties such as low density, good damping characteristics, good thermal conductivity and high elastic modulus[1,2]. Recently, there has been significant increase in the usage of magnesium alloy for automobile, aerospace components, computer, mobile phones, etc[3]. However, the applications of magnesium alloys are still limited due to some undesirable properties. The strength and ductility of magnesium alloys are generally poorer than those of common steel and aluminum alloys. The mechanical properties of wrought magnesium alloy are much better than those of the casting counterpart by plastic deformation processing and heat-treatment. In order to overcome these disadvantages, efforts are being devoted to developing wrought magnesium alloys with good mechanical properties[4,5].
Some researches show that rare earth elements are important for magnesium alloys[6]. LUO et al[7] have studied the strengthening effects of rare earth on wrought Mg-Zn-Zr-RE alloys. ZHOU et al[8, 9] have reported that Nd, La and Ce have effects on both microstructures and mechanical properties of cast AZ61 alloy. WANG et al [10] have reported the effects of RE on the microstructure and properties of cast AZ91 alloy. How- ever, few researches were reported on the deformation behavior and mechanical properties of wrought Mg-Al- Zn alloys with Y additions. In this study, hot rolling was carried out on four kinds of Mg-Al-Y-Zn alloys with variable Y contents. The effects of Y on both micro- structural evolution and mechanical properties were investigated.
2 Experimental
The chemical compositions of the studied alloys are listed in Table 1. The treatment procedures are described as follows. The cast ingots were homogenized at 400 ℃ for 16 h. Then, these plates were heated up to 350 ℃ and kept for 1 h, followed by rolling with heating rollers which were maintained at 100 ℃. 20 mm starting thickness of these plates was reduced down to 1mm, corresponding to the compressive deformation ratio of 95%. Multiple passes and re-heating between them were required. The standard tensile specimens were made from the as-rolled sheets. The rolled sheets were annealed at 250℃ for 1h, and the tensile properties of annealed sheets were also tested. Phase analysis was performed by XRD. Microstructures of experimental materials were observed by optical microscope(OM) and scanning electron microscope(SEM) equipped with energy dispersive spectrometer(EDS)
Table 1 Chemical composition of Mg-Al-Y-Zn alloys (mass fraction, %)
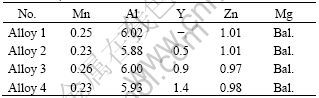
3 Results and discussion
3.1 Effect of Y on microstructures of AZ61 alloys
Fig.1 shows the optical micrographs of as-cast alloys. The second-phase in AZ61 alloy is Mg17Al12 phase according to the result of microanalysis by EDS. Mg17Al12 phase shows network shape and distributes mainly at grain boundaries. Also, a black dot-like phase dispersed in grains sparsely can be observed, which is proved to be Al-Mn compound by EDS. It can be seen from Fig.1 that by adding Y, α-Mg dendrites and Mg17Al12 phase can be refined, and the finest average grain size is obtained in alloy 3. Meanwhile, there are more precipitate phase particles dispersing in the grains of Y-containing alloys. The SEM image and line scanning result of this second-phase are shown in Fig.2. It is found that the contents of Al and Y in this phase are much higher than those of the matrix and the atomic ratio of Al to Y is about 2?1. The X-ray diffraction patterns of as-cast alloys are shown in Fig.3, from which the Y-containing phase is verified as Al2Y.
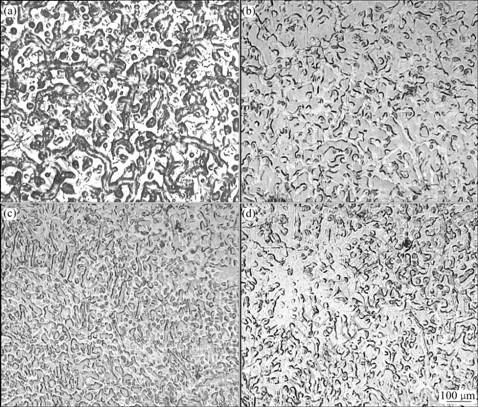
Fig.1 Optical micrographs of as-cast alloys: (a) Alloy 1; (b) Alloy 2; (c) Alloy 3; (d) Alloy 4
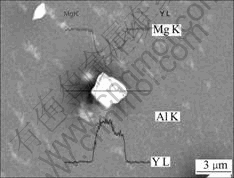
Fig.2 SEM image and line scanning result of Y-containing phase
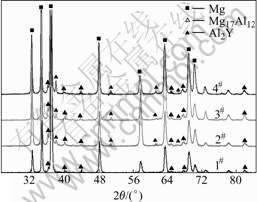
Fig.3 XRD patterns of as-cast alloys
The SEM images and Y map distributions of as-cast alloys are shown in Fig.4. It is suggested that with the increase of Y content, the amount of white and bright Y-containing phases becomes large and Mg17Al12 phase gets refined greatly. It can be observed that in alloy 4, Al2Y phase particles become coarsening.
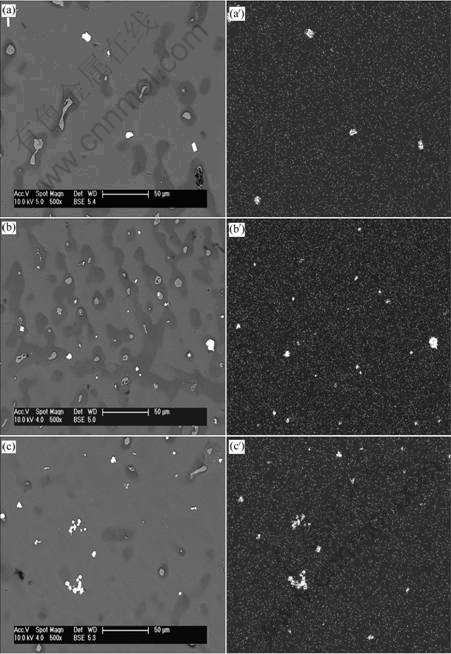
Fig.4 SEM images and Y map distributions of cast Mg-Al-Y-Zn alloys: (a), (a′) Alloy 2; (b), (b′) Alloy 3; (c), (c′) Alloy 4
Fig.5 shows the SEM images of the homogenized alloy 1 and alloy 3. After homogenization treatment, Mg17Al12 phase is dissolved in α-Mg matrix, so the amount of the second-phase decreases sharply. When Y is added into the alloy, Al2Y particles with high melting point and elevated temperature stability are almost not dissolved in the α-Mg matrix and they remain the same shape.
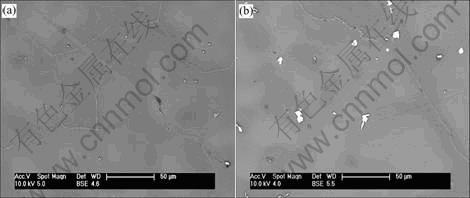
Fig.5 SEM images of homogenized AZ61 alloy (a) and AZ61+0.9%Y alloy (b)
The SEM images of hot-rolled alloys are shown in Fig.6. Comparing these images with Fig.4 the effects of hot rolling process on the microstructures of the alloys can be studied. It can be seen that the second-phases are broken into small particles and disperse in the matrix after hot rolling process. According to the EDS results, besides a few Al-Mn phase particles (sign A), these particles are Al2Y phase. Conglomeration and coarsening of Al2Y phase in alloy 4 are observed clearly.
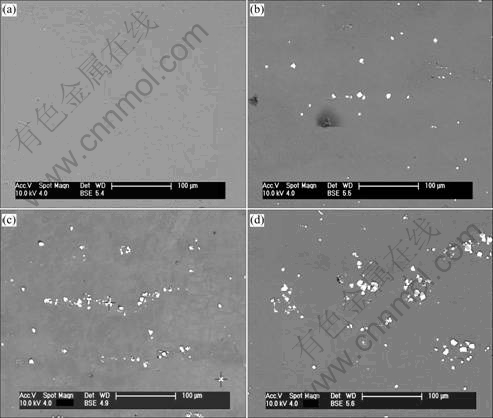
Fig.6 SEM images of alloys rolled at 350 ℃: (a) Alloy 1; (b) Alloy 2; (c) Alloy 3; (d) Alloy 4
Fig.7 shows the optical micrographs of rolled alloys. Because the rolling temperature is much higher than the recrystallized temperature of AZ61 alloy and the compressive deformation ratio is high to 95%, the dynamic recrystallization(DRX) process occurs in all of the alloys. It is observed that Y refines the rolled microstructures of alloys. Especially in alloy 3, the size of DRX grains is even smaller. This may be ascribed to the fact that the pinning effect of broken second-phase particles can suppress the growth of DRX grains.
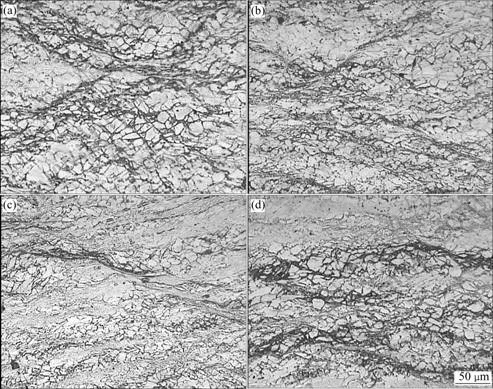
Fig.7 Optical micrographs of alloys rolled at 350 ℃ (longitudinal section): (a) Alloy 1; (b) Alloy 2; (c) Alloy 3; (d) Alloy 4
3.2 Mechanical properties of alloys
The results of the tensile tests of the rolled alloys at room temperature are shown in Table 2. It can be seen that the tensile strength and elongation of the AZ61 alloys are improved with the addition of Y either 0.5% or 0.9%, and AZ61 alloy has the best properties when the content of Y is 0.9%. However, by adding 1.4% Y, the tensile properties are reduced. After the rolled sheets were annealed at 250 ℃ for 1 h, the tensile properties of the annealed alloys were also tested. It can be seen that after annealing the strengths of alloys decline while the elongation increases greatly.
Table 2 Tensile properties of alloys at room temperature

3.3 Discussion
When Y is added into the AZ61 alloy, great effects of Y on the microstructures, solution treatment and hot rolling behavior of AZ61 alloy were observed. Alloys with variable Y contents all contain a phase of Al2Y with cubic structure. The lattice parameter of Al2Y phase is 0.786 nm [11]. Obviously, during the solidification process this phase is hard to act as nucleating core. The crystallizing temperature of Al2Y (980 ℃)[12] is much higher than the eutectic reaction temperature of L→ α-Mg+Mg17Al12 (451 ℃). So, Al2Y will form first and concentrate at the front edge of α-Mg phase, thus constitutional under-cooling appears ahead of the solid/liquid interface[7], which can promote the nucleation in the under-cooling zone. Meanwhile, the concentration of solute atoms at the solid/liquid interface can restrict the grain growth since the diffusion of atoms occurs slowly. Therefore, the as-cast microstructure can be refined by the Y addition. Also, due to the combination of Al and Y, the volume and size of Mg17Al12 phase decrease.
Some researchers have reported that grains can be refined by dynamic recrystallization(DRX) in magnesium alloys, and the mechanical properties are improved by grain refinement according to the Hall-Petch relationship [13-15]. In this study, for the alloys with Y addition, the stable Al2Y phases are the barrier for the slip of dislocation during rolling, and make more dislocations concentrate around it. This is beneficial to the nucleation of DRX. Moreover, the pinning effect of fine broken second-phase particles can suppress the growth of DRX grains. So, for the rolled alloy, the grains can be refined and the mechanical properties can be improved by certain Y addition. But when the Y addition is high to 1.4%, Al2Y phase becomes coarsening. After rolling, the coarsening and concentration of Al2Y phase in alloy 4 are more easily observed. This non-homogeneous microstructure is likely to cause stress concentration. Also, the coarse brittle intermetallic phase particles have influence on the micro-cracks formation and the flaws propagation, so the mechanical properties of alloy is decreased at room temperature.
4 Conclusions
1) The AZ61 alloys with variable Y contents all contain a phase of Al2Y. Y refines the microstructures of as-cast alloys.
2) During the rolling process, the pinning effect of fine broken Al2Y phase particles can suppress the growth of DRX grains, and the microstructures of rolled alloys are refined.
3) The tensile tests at room temperature indicate that the best comprehensive properties are obtained when the addition of Y is 0.9%. When the Y content is up to 1.4%, Al2Y phase coarsens, which leads to the drop of tensile strength.
References
[1] MORDIKE B L, EBERT T. Magnesium: Properties-applications- potential [J]. Mater Sci Eng A, 2001, 302: 37-45.
[2] KOJIMA Y. Platform science and technology for advanced magnesium alloys [J]. Mater Sci Forum, 2000(3): 350-351.
[3] AGHION E, BRONFIN B, ELIEZER D. The role of the magnesium industry in protecting the environment [J]. Journal of Materials Processing Technology, 2001, 117: 381-385.
[4] BARNETT M R, NAVE M D, BETTLES C J. Deformation microstructures and textures of some cold rolled Mg alloys [J]. Mater Sci Eng A, 2004, 386: 205-211.
[5] AGNEW S R, MEHROTRA P, LILLO T M, STOICA G M, LIAW P K. Crystallographic texture evolution of three wrought magnesium alloys during equal channel angular extrusion [J]. Mater Sci Eng A, 2005, 408: 72-78.
[6] PETTERSEN G, HOIER R, WESTENGEN H, LOHNE O. Microstructure of a pressure die cast magnesium-4wt.% aluminum alloy modified with rare earth additions [J]. Mater Sci Eng A, 1996, 207: 115-120.
[7] LUO Z P, SONG D Y, ZHANG S Q. Strengthening effects of rare earths on wrought Mg-Zn-Zr-RE alloys [J]. J Alloys Comp, 1995, 230: 109-114.
[8] ZHOU H T, ZENG X Q, DING W J, MA C J, ZHU Y P. Effect of La and Nd on microstructures and mechanical properties of AZ61 wrought magnesium alloy [J]. Trans Nonferrous Met Soc China, 2004, 14(1): 67-70.
[9] ZHOU H T, ZENG X Q, LIU W F, DING W J, ZHU Y P. Effect of Ce on microstructures and mechanical properties of AZ61 wrought magnesium [J]. Trans Nonferrous Met Soc China, 2004, 14(1): 99- 104.
[10] WANG Q D, LU Y Z, ZENG X Q, DING W J, ZHU Y P. Effects of RE on microstructure and properties of AZ91 magnesium alloy [J]. Trans Nonferrous Met Soc China, 2000, 10(2): 235-239.
[11] MA T H. The Structure and Performance of Nonferrous Alloys [M]. Beijing: Science and Technology Press, 1999.
[12] MASSALSKI T B. Binary Phase Diagrams [M]. Ohio: ASM, 1986.
[13] SITDIKOV O, KAIBYSHEV R. Dynamic recrystallization in pure magnesium [J]. Mater Trans, 2001, 42: 1928-1932.
[14] KAIBYSHEV R, GOTTSTEIN G. Correlation of plastic deformation and dynamic recrystallization in magnesium alloy ZK60 [J]. Acta Mater, 2001, 49: 1199-1207.
[15] TAN J C, TAN M J. Dynamic continuous recrystallization characteristics in two stage deformation of Mg-3Al-1Zn alloy sheet [J]. Mater Sci Eng A, 2003, 339: 124-132.
Foundation item: Project(2004GK1008-2) supported by the Science and Technology Program of Hunan Province, China; Project supported by the Key Laboratory for Nonferrous Metal of Education Department of Hunan Province, China
Corresponding author: YI Dan-qing; Tel: +86-731-8830263; E-mail: yioffice@mail.csu.edu.cn
(Edited by YUAN Sai-qian)