
Evolution of microstructure and texture of AZ61 Mg alloy during net-shape pressing of extruded perform
CAO Feng-hong(曹凤红)1, LONG Si-yuan(龙思远)1, CAO Han-xue(曹韩学)1,PU Yi-bing(蒲怡冰)1, Gerald S. COLE2
1. College of Materials Science and Engineering, Chongqing University, Chongqing 400030, China;
2. Light Weight Strategies LLC, Chicago 60102, USA
Received 23 September 2009; accepted 30 January 2010
Abstract: The micro orientation theological behavior of AZ61 Mg alloy during net-shape forming of tensile specimens via close-die pressing of extruded preformed and the effect of the press deformation rate on the microstructure characteristics were characterized with electron back-scattering diffraction (EBSD) orientation imaging microscopy and metallography. The results indicate that the intensity distribution of basal {0001}<
> texture on the cross-section of the extruded perform is uniform and parallel to the extrusion direction. Subjected to pressing in extrusion direction, deformation shear stress leads to grain rotation and basal texture {0001}<
> deviation from the extrusion direction, spreading in the direction perpendicular to pressing direction. The texture intensity increases with the press deformation rate and reaches its peak value at 50%, which is considerably lower than the value reached in extrusion deformation. Then, the texture intensity decreases with the press deformation rate reversely.
Key words: AZ61 magnesium alloy; extrusion; pressing; microstructure; texture
1 Introduction
With the rapid development of automobile industry, advanced manufacturing technologies make automobile production to develop to the direction of high-quality, high efficiency, low consumption and cleanness. The application of magnesium alloy in automobiles can play an important role in reducing the emission and conserving the energy resources. At present, one of the urgent problems to be resolved in the applications of magnesium alloy is to improve its strength to enable to apply to structural parts with requirement of high strength. However, magnesium alloy products are mainly castings, among which more than 90% are die castings, while magnesium alloy forgings with high-performance are rarely applied[1-3]. The main reason is because magnesium alloy is difficult to be forged due to its low plasticity. The high-cost production limits the application and development of high-performance magnesium alloy forgings to some extent.
One of the differences between magnesium alloy and other materials such as aluminum alloy lies in the fact that magnesium cannot be forged too many times, because the strength will decrease with the time increasing of heating and forging, especially when the heating temperature before forging is high and the time of reserving is long. Therefore, the time of forging for magnesium alloy in the process of forging compression should be controlled as short as possible[4]. Extrusion perform closed molding forming process is a new near/net-shape technology developed based on the principles of plastic forming of magnesium alloy. On this basis, this work concerns the evolution of microstructure and texture of magnesium alloy during close net-shape pressing of extruded perform AZ61 magnesium alloy by using the technology of EBSD.
2 Experimental
2.1 Material preparation
The alloy used in the present study was AZ61 Mg alloy with the chemical composition listed in Table 1. The alloy was supplied in the form of semi-continuous
Table 1 Chemical composition of as-cast AZ61 magnesium alloy (mass fraction, %)
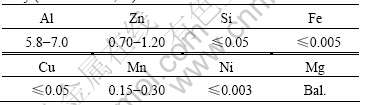
cast ingot with geometry of d 112 mm×250 mm.
2.2 Experimental methods
The ingot was homogenized at 385 ?C for 12 h, and then extruded by the XJ-800T horizontal extruder for the profiles, with the parameters of temperature 385 ?C, holding time 4 h, extrusion ratio 32 and extrusion speed 13-17 mm/s. The samples with a gauge geometry of 99.6 mm×9.0 mm for pressing were sectioned from the profiles by wire electric discharge machine, then were heated to the temperature of 400 ?C in an electric resistance furnace for 12 min. As the self-made die was preheated to the same temperature, the pressing process can be carried out on 200 t forging machine. The pressing direction is perpendicular to the section at the speed of 5-17 mm/s with deformation rates of 10%, 30%, 50%, 60%, respectively. The pressed samples were then air cooled and trimmed. The schematic diagram of the processes is shown in Fig.1.
The metallographic specimens of as-cast and heat treated states were sectioned from the billet at R/2 of its central line. They were firstly ground by abrasive papers, mechanically polished and chemically etched for 5-30 s using the solution of picric acid (3 g)+acetic acid (20 mL) +C2H5OH(50 mL) H2O (20 mL). The microstructure was observed by optical microscopy (OM). The specimens as-extruded and as-die pressed with different rates were sectioned as shown in Fig.2, then ground and electro- polished for EBSD test in the material testing centre at Chongqing University.
3 Results and analysis
3.1Microstructure evolution
The microstructures of as-cast and solution treated at 400 ?C AZ61 are represented in Figs.3(a) and (b) respectively. The as-cast AZ61 is characterized by α-Mg and intermetallic network phase β-Mg17Al12 distributed along the grain boundaries, which is produced by divorced eutectic phase. Such microstructure is typical in as-cast Mg-Al series, marked by image analysis as secondary dendrite arm with spacing of 35 μm. In Fig.3(b) uniform single phase is presented due to β-phase remelting into α-Mg after solution treatment at 400 ?C for 12 h, and its average grain size are 110 μm. The microstructures of as-extruded AZ61 and as-die pressed with different rates are shown in Fig.4. It can be seen from Fig.4(a) that significant dynamic recrystallization

Fig.1 Schematic diagram of extrusion and forging compound process for magnesium alloy AZ61 (extruded perform billet size 99.6 mm×9.0 mm×2.4 mm (gauge length), temperature of extruding and hot pressing 385-400 ?C, extrusion speed 13-17 mm/s, close die hot pressing speed 5.6 mm/s): (a) Ingot casting; (b) Extrusion performing; (c) Sectioning; (d) Net-shape pressing
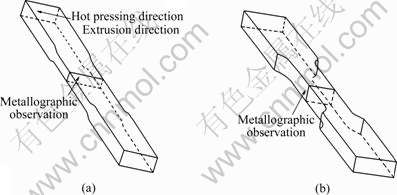
Fig.2 Schematic diagram of as-extruded and close-die hot pressed tensile sample: (a) Extruded perform billet; (b) Net-shape pressed tensile specimen
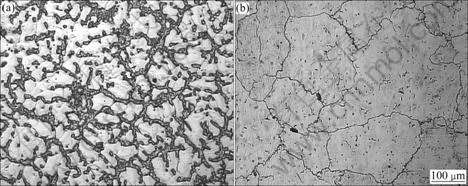
Fig.3 Microstructures of as-cast AZ61 and after heat treatment: (a) As-cast AZ61; (b) Heat treated
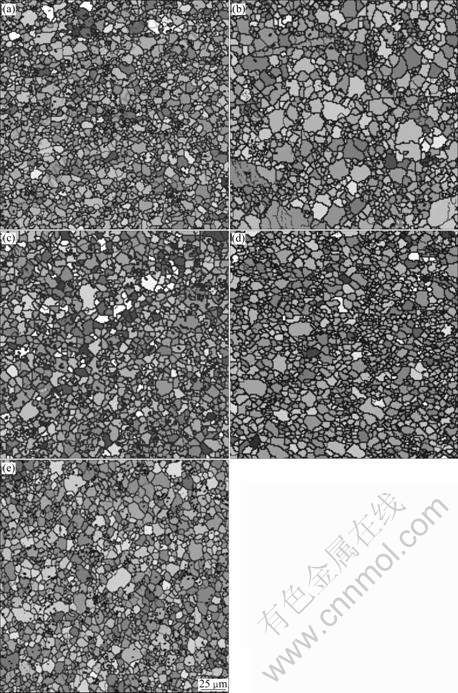
Fig.4 Microstructures of die forged AZ61 under different deformation rates: (a) As-extruded; (b) Deformation 10%; (c) Deformation 30%; (d) De- formation 50%; (e) Deformation 60%
(DRX) takes place during extrusion process, and coarse grain is replaced by finer equiaxed grain with average size of 3.5 μm. When the as-extruded sample is pressed in the die at 400 ?C with deformation rate 10%, the average size of finer equiaxed grain tends to increase to 6 μm. DRX is almost completed as the deformation rate increases, and the recrystallized grains are distributed parallel to the pressing direction and rotated along the boundaries. When the deformation rate is within 30%, the average grain size is 2-3 μm, whereas the rate reaches 50%-60%, the grain size is decreased to 1-2.5 μm, and the recrystallized grains of AZ61 magnesium alloy are elongated or crushed and present on “S” streamlines, as shown in Fig.5.
3.2 Microstructure analysis
At a certain deformation temperature and small deformation degree, distrotional energy of metal material is relatively small even without enough recrystallization energy, so the grain size of alloy does not change obviously, while the deformation rates arrive at a certain value (2%-10%)[5], the grain size tends to increase with small deformation rate (10%) under 400?C. The average recrystallized grain size can be expressed by d= K(G/N)1/4, where G is the linear velocity of growth, N is the nucleation rate, K is the proportional factor. The recrystallized grain size is determined by the ratio of G/N. The ratio of G/N is relatively small with small deformation rate, so the deformation rate of AZ61 alloy is 10%, which grows up apparently. With increasing deformation rate, distrotional energy of metal material raises too, G and N increase at the same time, but the increment rate of N is greater than the increment rate of G, which results in the rate of G/N reduce and grain size is refined, while deformation rate reaches up to 50%-60%, the increment rate of N and increment rate of G are basically analogous and the recrystallized grain size of alloys basically tends to be stable.
4 AZ61 microstructure evolutions at different deformation rates
4.1 Microtexture of AZ61
Texture and grain orientation of AZ61 magnesium alloy during as-extruded and as-die pressing of extruded preformed with different rates are illustrated in Figs.6(a)-(b). Grain boundary sliding has little influence on grain orientation, and texture formation is caused by intracrystalline plastic slip. Generally, the deformation during hot processing is also caused by texture, and various types of texture represent different deformation mechanisms[6]. Studies[7-11] have shown that a strong basal texture (0001) is formed as a result of basal slip and pyramidal twinning in magnesium alloys during rolling. Fig.6(a) is characterized by initial texture formed in extruded preforming, and the initial orientation is at basal texture {0001}<
> located in the centre of pole figure (Y0), while the basal texture is symmetrical and parallel to extrusion direction (ED), whereas minute quantity of grains is along prismatic plane (
) parallel to ED, and the maximum texture intensity is 15.37 and the peak value of misorientation of grain is 28?-38?. Texture and grain orientation vary significantly with deformation rate increment, whereas texture intensity decreases. It can be seen from Figs.6(b)-(c) that basal texture {0001}<
> of initial orientation deviates from Y0 with a certain angle, and texture intensity is 5.48, 6.84, 9.62, 9.30 respectively. The
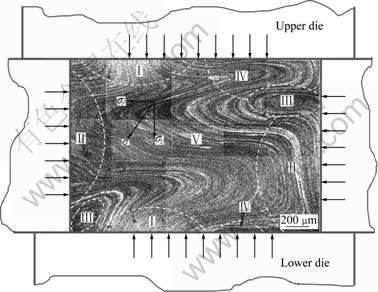
Fig.5 Forces schematic of sample test in die cavity
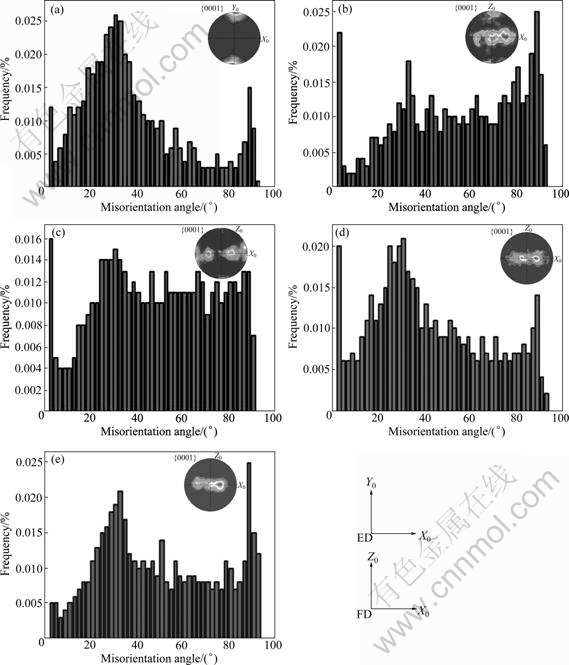
Fig.6 Texture and grain orientation of AZ61 magnesium alloy during extrusion-die forging compound: (a) As-extruded; (b) Deformation 10%; (c) Deformation 30%; (d) Deformation 50%; (e) Deformation 60%
maximum texture intensity can be obtained when deformation rate of pressing reaches up to 50%, and further deformation leads to texture intensity decrease.
4.2 Analysis and discussion
The deformation texture mechanism of magnesium alloys is caused by grain rotation under external stress, and differs as stress changes.
In the extrusion process, the state of plane stress is tensile in extrusion direction(ED) and compressed in normal direction (ND), and the initial basal texture {0001}<
> is parallel to ED, as shown in Fig.7(a).
Initial deformation for specimens in hot pressing process of AZ61 is similar to uniaxial compression, and only transverse deformation takes place, whereas longitudinal size remains the same. Further deformation leads to metallic flow in transverse direction under three-dimensional compressive stress. Because width/height ratio is 4, deformation instability occurred in initial pressing, and the shear stress incurs irregular metallic flow, which is illustrated in Ⅱ and Ⅲ zones in Fig.5. Meanwhile, the shear stress also leads to grain rotation along boundaries with certain angles, and basal texture and grain orientation are easily perceived with
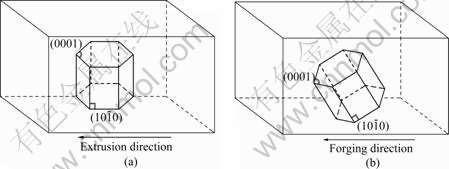
Fig.7 Schematic illustration of dominant texture during extrusion-die forging compound: (a) As-extruded; (b) Deformation of 60% after hot net-shape pressing
increasing deformation. The strong basal fiber texture formed during extrusion gives rise to weak basal texture intensity at the beginning of pressing process, so the basal slip and pyramidal twinning cannot effort under normal compressive stress. Therefore, further deformation can be easily conducted, which, in contrast, leads to fiber texture[12] intensified. Simultaneously, the basal preferential orientation deviates from extrusion direction and aligns with the main pressing and deformation direction under three-dimensional compressive stress, as shown in Fig.7(b).
4 Conclusions
1) The average size of as-extruded grain is 3.5 μm, while the grain after hot-pressing is significantly refined. And the average size of grains is 6 μm which tends to increase with small deformation. As deformation rate is 30%, the average size of grains is 2-3 μm, whereas the deformation rate reaches up to 50%-60%, the size is decreased to 1-2.5 μm and tends to be stable, but grain refined are not obvious.
2) The profiles of extruded preforming is dominantly on basal texture {0001}<
>, and the basal plane is parallel to extrusion direction (ED). As deformation continues, the inner shear stress gives rise to grain rotation along boundaries with certain angles and initial basal texture and grain orientation vary remarkably, though the texture intensity is weaker than that as-extruded. The basal preferential orientation deviates from extrusion direction and aligns with the pressing direction.
References
[1] KANG H T, OSTROM T. Mechanical behavior of cast and forged magnesium alloys and their microstructures [J]. Materials Science and Engineering A, 2008, 49(1/2): 52-56.
[2] GUAN S K, WU L H, WANG P. Hot forgeability and die-forging forming of semi-continuous casting AZ70 Mg-alloy [J]. Materials Science and Engineering A, 2009, 499(1/2): 187-191.
[3] WU Li-hong, GUAN Shao-kang, WANG Li-guo, LIU Jun. Wrought magnesium alloys and several key factors affecting the forging forming [J]. Forging Technology. 2006, 31(4): 7-10. (in Chinese)
[4] LONG Si-yuan, CAO Feng-hong, LIAO Hui-min. A compound forming method of magnesium alloys. CN 200810069225.7[P]. 2008-01-10.
[5] CUI Zhong-qi. Metallurgy and heat treatment [M]. 2006: 1.
[6] YANG Ping, REN Xue-ping, ZHAO Zu-de. Microstructures and textures in hot deformed and annealed AZ31 magnesium alloy [J]. Transactions of Materials and Heat Treatment, 2003, 12(4): 12-17. (in Chinese)
[7] MYAGCHILOV S, DAWSON P R. Evolution of texture in aggregates of crystals exhibiting both slip and twinning [J]. Modelling and Simulation in Materials Science and Engineering, 1999, 7: 975-1004.
[8] WAGNER L, HILPERT M, WENDT J. On methods for improving the fatigue performance of the wrought magnesium alloys AZ31 and AZ80 [J]. Materials Science Forum, 2003, 419/422: 93-102.
[9] KALIDINDI S R. Modeling anisotropic strain hardening and deformation textures in low stacking fault energy materials [J]. International Journal of Plasticity, 2001, 17: 837-860.
[10] CHRISTIANJ W, MAHAJAN S. Deformation twinning [J]. Progress in Materials Science, 1995, 39: 1-157.
[11] POSS R. Sheet metal production of magnesium [J]. Materials Science Forum, 2003, 419/422: 327-336.
[12] VALLE J A, PRADO M T, RUANO O A. Texture evolution during large strain hot rolling of the AZ61 Mg alloy [J]. Materials Science and Engineering A, 2003, 355: 68-78.
(Edited by LI Xiang-qun)
Foundation item: Project(CSTC2007AA4008) supported by the Scientific and Technological Project in Chongqing of China
Corresponding author: CAO Feng-hong; Tel: +86-13996048486; Fax: +86-23-65112626; E-mail: caofh2004@yahoo.com.cn