
Thermodynamic evaluation of new metallurgical refining processes for SOG-silicon production
Kazuki MORITA, Takeshi YOSHIKAWA
Institute of Industrial Science, The University of Tokyo, 4-6-1 Komaba, Meguro-ku, Tokyo 153-8505, Japan
Received 17 June 2010; accepted 15 August 2010
Abstract: With the aim of developing a new silicon refining process for production of solar grade silicon, a low-temperature refining technique referred to as “solidification refining of silicon with a Si-Al solvent at low temperature” was studied. The refinability of silicon by the partial solidification from a Si-Al solvent was discussed with thermodynamic evaluation for the impurity segregation between solid silicon and a Si-Al solvent. Impurity segregation ratios were measured by using temperature gradient zone melting method for phosphorus and boron and were estimated by the thermodynamic calculation for metallic impurities. The excellent refinability was clarified from the extremely small segregation ratios of impurities at lower temperature and was also confirmed by the test refining with the partial solidification under the induction heating. Furthermore, silicon crystal growth was studied by directional solidification experiments of a Si-Al alloy, and was estimated to be diffusion controlled.
Key words: silicon; Si-Al solvent; purification; solar cells; segregation; thermodynamics; solidification
1 Introduction
The production of crystalline silicon solar cell has increased significantly during this decade. However, unstable supply of silicon feedstock and sudden increase in feedstock price have been caused especially in this five years. This feedstock problem started from its strong dependence on expensive, off-spec semiconductor-grade silicon (SEG-Si). Although construction of silicon production plants has been accelerated worldwide, the development of a low-cost silicon production is essential for the further spreading of silicon solar cells.
Purification of silicon through the metallurgical treatments may be a promising way for producing solar grade silicon (SOG-Si) from metallurgical grade silicon (MG-Si). Solidification of silicon is a well known process for removing metallic impurities such as iron and titanium due to their strong segregation tendencies. However, the removal of phosphorus and boron, which are important dopants in silicon, is practically impossible by solidification because their segregation coefficients are not adequately small (kP=0.35, kB=0.8[1]). Vacuum melting and oxidation treatment are often employed to remove phosphorus[2] and boron[3]. These processes are used in combination to convert MG-Si into SOG-Si. Vacuum and oxidation processes require high temperatures and long durations, and thus a new low cost process for manufacturing silicon is crucial to ensure a stable supply of SOG-Si.
Retrograde solubilities of major impurities in solid silicon have been reported[1], indicating their relative instability in silicon at lower temperature. Therefore, the low temperature refining of silicon by the partial solidification technique with a Si-Al solvent was investigated[4-12]. The process was; 1) alloying MG-Si with aluminum to form a Si-Al solvent, 2) partial solidification of silicon from the solvent around 1 273 K and 3) collection of the refined silicon crystals by acid leaching. We expected the effective removal of impurities by exploiting the enhanced solid/liquid segregation tendency of impurities at low temperature.
In this work, the refinability of silicon by the partial solidification from a Si-Al solvent was discussed with thermodynamic evaluation for the impurity segregation between solid silicon and the Si-Al solvent. The high refinability was clarified from the extremely small segregation coefficients of impurities at lower temperature and was confirmed through the refining test by the partial solidification of silicon from the Si-Al solvent under the induction heating. Furthermore, crystal growth of silicon was studied by directional solidification xperiments of a Si-Al alloy, aiming at the optimum refining condition.
2 Thermodynamic evaluation of impurities removal during partial solidification of silicon from Si-Al solvent
During the partial solidification of silicon from a Si-Al solvent, impurity elements are subject to a solid/ liquid segregation. In order to know the refinability during the partial solidification process, knowledge of the segregation ratios of impurities between solid silicon and Si-Al solvent is essential. The segregation ratio, ki, is defined as the molar ratio of solid silicon to solvent.
(1)
We measured the segregation ratios of phosphorus (1 173-1 373 K) and boron (1 273-1 473 K) by the temperature gradient zone melting (TGZM) method[5, 8]. An Al-P or Al-B foil was initially located between two single crystalline silicon plates, and was placed in the temperature gradient region in the horizontal resistance furnace. The foil formed the Si-Al melt zone and migrated in solid silicon toward the high temperature direction, leaving solidified silicon equilibrated with the melt behind. An example of the sample after the TGZM experiment is shown in Fig.1. By analyzing the phosphorus or boron concentration in solid silicon and in the melt zone after quenching the sample, segregation ratios could be determined. The measured segregation ratios of P and B are shown in Fig.2, together with their segregation coefficients between solid and liquid silicon
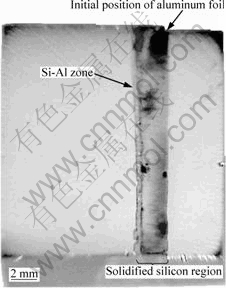
Fig.1 Example of cross section of sample after TGZM experiment (Solidified silicon region was colored after etching with HF-HNO3 acid)
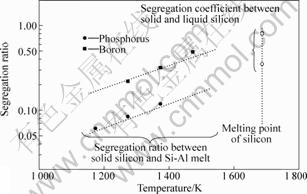
Fig.2 Temperature dependence of segregation ratios of phosphorus and boron between solid silicon and Si-Al solvent
at its melting points. Segregation ratios of both elements decrease as the temperature decreases from the melting point of silicon. The removal of phosphorus and boron by the partial solidification of silicon from a Si-Al solvent was thus found to be effective at lower temperature by using smaller segregation ratios.
It is difficult to measure the segregation ratios of metallic impurities between solid silicon and Si-Al solvent because of their extremely small contents in solid silicon. The segregation behavior was thus evaluated by the following thermodynamic approach[6]. At the equilibrium between solid silicon and Si-Al melt, the chemical potential of an impurity element is identical in two phases as expressed in Eq.(2) or (3).
(2)
(3)
where
and ai are the chemical potential at the standard state and the activity of the impurity element i, respectively. The segregation ratio can be expressed as Eq.(4) by rearranging Eq.(3).
(4)
where
denotes the Gibbs free energy change for fusion of the impurity element. The excess Gibbs free energy of the impurity element in a Si-Al solvent,
, is estimated from the Gibbs free energies of the binary Si-Al, Si-i and Al-i melts[6] with the aid of the Gibbs-Duhem integration method proposed by TOOP[12]. The excess Gibbs free energy of the impurity element in solid silicon,
is determined from its solid solubility[13]. The segregation ratios calculated by Eq.(4) are summarized in Table 1. The segregation ratios of all investigated impurities were found to be much smaller than the segregation coefficients between solid and liquid silicon at its melting point. In addition, their values decrease with the decrease of temperature.
Table 1 Estimated segregation ratios of metallic impurities between solid silicon and Si-Al solvent at 1 073 and 1 273 K with segregation coefficients between solid and liquid silicon
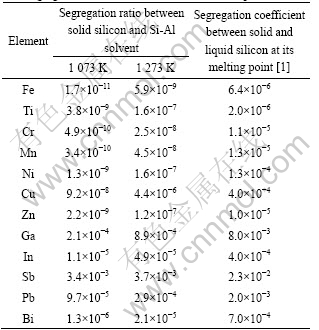
In Table 1, difference in the refinability between the partial solidification with a Si-Al solvent at low temperature and the ordinary silicon solidification is discussed. The change in the contents of typical impurity elements in silicon, “lifetime killer” elements, iron and titanium, and major dopants, phosphorus and boron is compared. The impurity contents of MG-Si are taken as follows: Fe 2×10-3 (mass fraction), Ti 1×10-4, P 2×10-5, and B 2×10-5. For the partial solidification from a Si-Al solvent, MG-Si is assumed to be alloyed with pure aluminum to be the composition of Si-55.3%Al (molar fraction) and silicon is solidified partially at 1 273 K. If impurity contents can be reduced to the initial contents of solidified silicon,
, at equilibrium solidification, impurity contents after refining, ci, are expressed as
(5)
The estimated impurity contents of silicon after solidification refining are shown in Fig.3, with allowable contents for SOG-Si[14]. Iron and titanium can be reduced below the allowable contents by the low temperature solidification, whereas phosphorus and boron are decreased at a certain level but inadequate for SOG-Si. Hence, impurities can be effectively removed through the partial solidification of silicon from the Si-Al solvent by using the enhanced segregation tendency of impurities at low temperature.
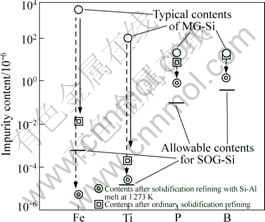
Fig.3 Comparison of impurity contents of silicon after low temperature solidification refining with a Si-Al solvent at 1 273 K and ordinary silicon solidification refining of MG-Si
3 Test refining of silicon by partial solidification from Si-Al solvent under induction heating
Test refining was carried out under induction heating. When a Si-Al alloy is melted and cooled down under induction heating, the solidified silicon is agglomerated to either top or bottom side, as shown in Fig.4[7]. This solidification allows us to collect silicon crystals selectively from the alloy and to suppress the loss of aluminum and silicon considerably during the acid leaching.
Synthesized MG-Si, whose composition is shown in Table 2, was alloyed with pure aluminum to prepare
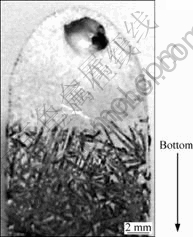
Fig.4 Cross section of solidified Si-55.3% Al alloy under induction heating
Table 2 Impurity contents of refined silicon and removal fractions in parenthesis after test refining
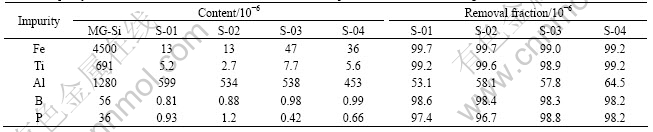
Si-55.3%Al alloy for S-01, 02 and Si-64.6%Al alloy for S-03, 04. Liquidus temperatures of the alloys are 1 273 K and 1 173 K, respectively. After heating to and holding at 1 323 K for S-01, 02 and at 1 223 K for S-03, 04 under the induction heating, those alloys were cooled to 873 K. The silicon agglomeration part in the sample (Fig.4) was extracted and crashed into the particles less than 840 μm. The refined silicon was collected after acid leaching of the particles with aquaregia and was subjected to the chemical analysis. Impurity contents are summarized in Table 2[7]. High removal fractions of iron and titanium were obtained although these were not sufficient in view of the extremely small segregation ratios in Table 2. Removal fraction of boron is larger than that expected from the evaluation of directional solidification with the segregation ratio (solidified from 1 273 K, removal fraction of 97.4%)[8]. This is attributed to a decrease in the boron content of the Si-Al solvent due to TiB2 formation with soluble titanium in the alloy[9]. The removal fraction of phosphorus agreed fairly well with the calculated value (solidified from 1 273 K, removal fraction of 94.7%)[5]. Accordingly, high purification was achieved by the partial solidification of silicon from a Si-Al solvent under the induction heating. However, the aluminum content is larger than the solidus composition of silicon, which is caused by the entrapped solvent in silicon as shown in Fig.5. In order to obtain the optimal refining condition by preventing the solvent entrapment during the partial solidification, silicon crystal growth was studied in the following section.
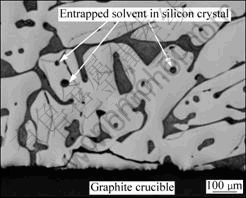
Fig.5 Microstructure of bottom of solidified Si-55.3% Al alloy in test refining
4 Morphology of silicon crystal grown from Si-Al solvent by directional solidification
Directional solidification was carried out to obtain bulk silicon crystals from a Si-Al solvent[11]. Si- 55.3% Al alloy was melted uniformly in a graphite crucible at 1 323 K. The sample was then cooled to 1 173 K by lowering it with the rate of 0.02-0.08 mm/min at a temperature gradient of 1.5-4.0 K/mm. The solidified sample was polished at the vertical cross section, and the shape of the silicon crystals and that of the solidification interface were observed.
Fig.6 shows the vertical cross sections of the samples obtained at various cooling rates and temperature gradients. Larger silicon crystals were found to grow near the bottom of the sample. A silicon phase was also observed in the upper part of sample. A similar phenomenon was observed in the air-cooled Si-Al alloy. Therefore, these silicon crystals are expected to be precipitated during cooling after the experiment, from the uniform alloy. The samples (a), (b) and (c) in Fig.6 were obtained at the identical temperature gradient with different cooling rates. It is clear that as the cooling rate decreases, the interface of the silicon crystals at the bottom of the sample becomes flat. The same tendency is observed at high temperature gradients (Fig.6(d)-(f)). The solid fractions of samples (c) and (f), in which the crystal-melt interface is flat, could be roughly estimated from the phase diagram of the Si-Al binary system[13].
From Fig.6, the growth condition for the facetted growth of silicon is discussed. We have discussed the growth rate of silicon from the alloy solvent under the diffusion control in Ref.[6]. Under the assumption that silicon crystal growth is diffusion controlled, the growth rate, v, is expressed as follows on the basis of the steady-state diffusion equation for silicon.
(6)
where,
,
, and x denote the diffusion coefficient of silicon, silicon content at the growth interface, and distance in the crystal growth direction, respectively. In this calculation, the difference
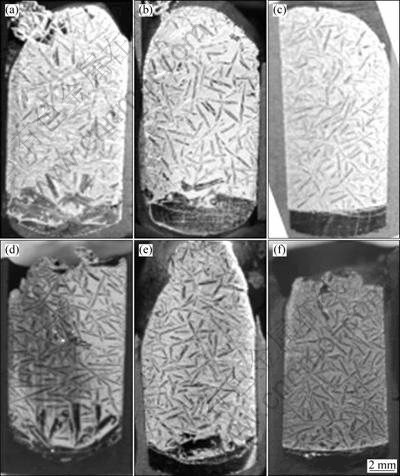
Fig.6 Cross sections of directionally solidified Si-55.3% Al alloys at temperature gradients of 1.5 K/min (a, b, c) and 4 K/min (d, e, f) and different descent rates: (a), (d) 0.07 mm/min; (b), (e) 0.05 mm/min; (c), (f) 0.02 mm/min
between the molar volumes of solid silicon and the Si-Al solvent is ignored. The growth rate of silicon is rewritten as Eq.(7) with the temperature gradient,
.
(7)
When the temperature gradient is assumed to be constant, the maximum value of
is the liquidus slope at the growth temperature corresponding to solidification at the growth interface but not in the melt. From Eq.(7), the growth rates of the silicon crystals at 1273 K are calculated to be 1.5×10-3 and 4.0×10-3 mm/min at the temperature gradients of 1.5 K/mm and 4.0 K/mm, respectively. The faceted growth rates of the samples (c) and (f) in Fig.6 were 8.7×10-4 and 2.3×10-3, respectively, which are estimated from the thickness of the silicon crystal and the cooling duration. As the experimental growth rate is roughly in accordance with that calculated above, the faceted growth of silicon from the Si-Al solvent is found to be controlled by the diffusion of silicon. Silicon diffusion in the melt must be thus accelerated to achieve high-efficiency crystal growth of silicon.
5 Conclusions
1) The impurity segregation ratios between solid silicon and a Si-Al solvent were measured by temperature gradient zone melting method for phosphorus and boron and were estimated by the thermodynamic calculation for metallic impurities. Since the segregation ratios of all investigated impurities were found to be much smaller than the segregation coefficients between solid and liquid silicon at its melting point, impurities can thus be effectively removed through the partial solidification of silicon from a Si-Al solvent at low temperature.
2) The test refining was carried out by the partial solidification of silicon from a Si-Al solvent under the induction heating. As removal fractions over 99% for iron and titanium and 95% for phosphorus and boron were obtained, the excellent refinability of the low temperature solidification was clarified.
3) Silicon crystal growth was studied through the directional solidification of a Si-Al alloy. The faceted growth of silicon was obtained at a certain solidification condition and was estimated to be diffusion controlled.
Acknowledgement
The present research is partly supported by a Grant-in-Aid for Scientific Research on Priority Area (B) from the Ministry of Education, Culture, Sports, Science and Technology of Japan.
References
[1] TRUMBORE F A. Solid solubilities of impurity elements in germanium and silicon [J]. Bell System Technical Journal, 1960, 39: 206-233.
[2] HANAZAWA K, YUGE N, KATO Y. Evaporation of phosphorus in molten silicon by an electron beam irradiation method [J]. Materials Transactions, 2004, 45(3): 844-849.
[3] NAKAMURA N, BABA H, SAKAGUCHI Y, KATO Y. Boron removal in molten silicon by a steam-added plasma melting method [J]. Materials Transactions, 2004, 45(3): 858-864.
[4] YOSHIKAWA T, MORITA K. Solid solubilities and thermodynamic properties of aluminum in solid silicon [J]. Journal of Electrochemistry Society, 2003, 150(8): G468-471.
[5] YOSHIKAWA T, MORITA K. Removal of phosphorus by the solidification refining with Si-Al melts [J]. Science and Technology of Advanced Materials, 2003, 4(6): 531-537.
[6] YOSHIKAWA T, MORITA K. Thermodynamics on the solidificational refining of Si with Si-Al melts [C]//EPD Congress 2005, TMS Annual Meeting. San Francisco, 2005: 549-558.
[7] YOSHIKAWA T, MORITA K. Solidification refining of Si with Si-Al melt using electromagnetic force [J]. ISIJ international, 2005, 45(7): 967-971.
[8] YOSHIKAWA T, MORITA K. Removal of B from Si by the solidification refining with Si-Al melts [J]. Metallurgical and Materials Transactions, 2005, 36B: 731-736.
[9] YOSHIKAWA T, ARIMURA K, MORITA K. B Removal by Ti addition in the solidification refining of Si with Si-Al melt [J]. Metallurgical and Materials Transactions, 2005, 36B: 837-842.
[10] YOSHIKAWA T, MORITA K. Continuous solidification of Si from Si-Al melt under the induction heating [J]. ISIJ International, 2007, 47(4): 582-584.
[11] NISHI Y, KANG Y, MORITA K. Control of Si crystal growth during solidification of Si-Al melt [J]. Materials Transactions, 2010, 51(7): 1227-1230.
[12] TOOP G W. Predicting ternary activities using binary data [J]. Transactions of the Metallurgical Society of AIME, 1965, 242: 850-855.
[13] YOSHIKAWA T, MORITA K, KAWANISHI S, TANAKA T. Thermodynamics of impurity elements in solid silicon [J]. Journal of Alloys and Compounds, 2010, 490: 31-41.
[14] DAVIS J R, ROHATGI A, HOPKINS R H, BLAIS P D, RAI-CHOUDHURY P, MCCORMICK J R, MOLLENKOPF H C. Impurities in silicon solar cells [J]. IEEE Transactions of Electrodevices, 1980, ED-27(4): 677-687.
一种新的精炼太阳能级多晶硅工艺的热力学分析
Kazuki Morita, Takeshi Yoshikawa
Institute of Industrial Science, The University of Tokyo, 4-6-1 Komaba, Meguro-ku, Tokyo 153-8505, Japan
摘 要:提出了一种新的硅精炼工艺生产太阳能级多晶硅,即Si-Al熔体低温凝固精炼硅技术。通过杂质在固相硅和Si-Al熔体中的分离热力学分析研究了采用Si-Al熔体分区凝固精炼硅的可行性。用温度梯度区域熔炼法来测定磷和硼的分离比,采用热力学计算金属杂质的分离比。新工艺具有很小的杂质低温分离比,表明其有很好的精炼能力。采用感应加热分区凝固实验进行验证;对Si-Al合金定向凝固中硅晶体生长进行了研究,结果表明,硅晶体的生长过程是受扩散控制的。
关键词:硅;Si-Al熔体;精炼;太阳能电池;偏析;热力学;凝固
(Edited by YANG Hua)
Corresponding author: Kazuki MORITA; Tel/Fax: +81-3-5452-6328; E-mail: kzmorita@iis.u-tokyo.ac.jp
DOI: 10.1016/S1003-6326(11)60766-8