
Selective laser melting of Fe-Ni-Cr layer on AISI H13 tool steel
Byeong-Don JOO, Jeong-Hwan JANG, Jae-Ho LEE, Young-Myung SON, Young-Hoon MOON
School of Mechanical Engineering, Pusan National University, Busan, 609-735, Korea
Received 18 June 2008; accepted 10 March 2009
Abstract: An attempt to fabricate Fe-Ni-Cr coating on AISI H13 tool steel was performed with selective laser melting. Fe-Ni-Cr coating was produced by experimental facilities consisting of a 200 W fiber laser which can be focused to 80 μm and atmospheric chamber which can control atmospheric pressure with N2 or Ar. Coating layer was fabricated with various process parameters such as laser power, scan rate and fill spacing. Surface quality and coating thickness were measured and analyzed. Three different surface patterns, such as typeⅠ, typeⅡand type Ⅲ, are shown with various test conditions and smooth regular pattern is obtained under the conditions as 10 μm of fill spacing, 50-350 mm/s of scan rate and 40 μm of fill spacing, 10-150 mm/s of scan rate. The maximum coating thickness is increased with power elevation or scan rate drop, and average thickness of 10 μm fill spacing is lower than that of 40 μm fill spacing.
Key words: selective laser melting; laser coating; coating thickness; Fe-Ni-Cr layer; AISI H13 tool steel
1 Introduction
Selective laser melting is a non-conventional production process where layers of powder are molten with a laser heat source. It is widely used in various industries[1]. Depositing a coating by selective laser melting is a process which can provide surface properties different from those of the bulk material. Coating by selective laser melting has many advantages. First, surface properties such as hardness, abrasion resistance and corrosion resistance can be improved. Second, many kinds of materials such as polymers, ceramics, metals, and many types of composites can be used. And third, coating can be fabricated locally. Therefore, it can be used in aeronautical, automotive, marine and chemical industries[2-5].
Unfortunately, selective laser melting process is influenced by many factors such as laser, substrate, powder and surrounding. And together with these factors, the resulting properties are determined by many sub-processes such as laser systems, optic systems, heat transfer, absorption of energy, melting and solidification, related material transformations and fluid flows. Therefore, the process is too difficult to control[6].
Various lasers such as CO2 laser, Nd:YAG laser, diode laser, disk laser and fiber laser have been developed for selective laser melting. Recently, the fiber laser has been receiving attention due to its advantages of high power, high beam quality, simplicity, high electrical to optical efficiency, reliability, excellent thermal properties, robustness and low running cost[7].
In this research, coating was produced by fiber laser with various laser parameters such as laser power, scan rate and fill spacing. Surface behavior and coating thickness were measured and the effects of laser parameters on coated layer were investigated.
2 Experimental
2.1 Experimental equipment
The laser apparatus used in this experiment, as schematically shown in Fig.1, mainly consists of a fiber laser (IPG YLR-200) which has wave length of 1.07 μm and maximum output power of 200 W, a scanner system (SCANLAB hurrySCAN?20), an automatic powder delivery system, an atmospheric chamber which can control atmospheric pressure, and a computer system for process control.
The powder layer was leveled with a layer bar so as to obtain a flat powder surface. The processing parameters can be controlled: spot size of 80 μm, laser power up to 200 W, scan rate up to 350 mm/s and the minimum fill spacing of 10 μm.
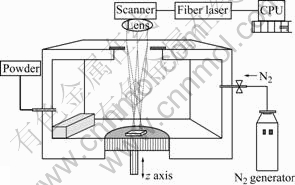
Fig.1 Schematic drawing of laser system
2.2 Experimental method
The substrate was AISI H13 tool steel which is hot rolled mold tool steel and its chemical composition is shown Table 1. The powder used in this study was Fe-Ni-Cr with the chemical composition given in Table 2 and the particle size of 20 μm. The layer was fabricated at laser power of 50-200 W, scan rate of 50- 350 mm/s and fill spacing of 10-80 μm. Other process parameters used in the experiment are shown in Table 3.
Table 1 Chemical composition of substrate

Table 2 Chemical composition of Fe-Ni-Cr powder

Table 3 Experimental conditions
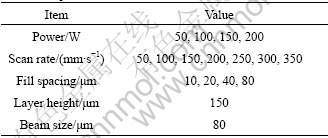
Surface properties which mainly result in layer properties were measured and the maximum and average coating thicknesses were measured and analyzed.
3 Results and discussion
3.1 Surface behaviors in various laser conditions
Fig.2 shows schematic drawing of three different surface patterns. The pattern which has balling and rough surface was defined as typeⅠ. Well connected pattern which has fine surface was defined as type Ⅱ. And type Ⅲ was not connected pattern. Surface morphologies produced in various laser conditions are shown in Fig.3.
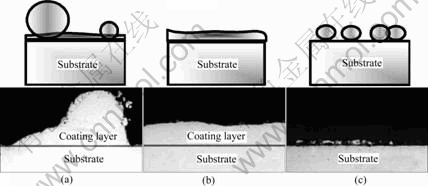
Fig.2 Schematic drawings of surface patterns: (a) TypeⅠ; (b) Type Ⅱ; (c) Type Ⅲ
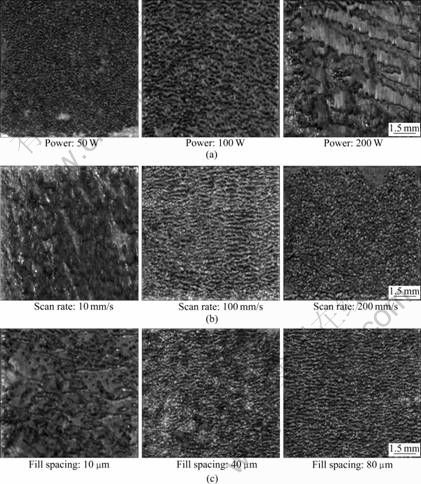
Fig.3 Morphologies of coating surface produced in various laser conditions: (a) Scan rate 100 mm/s, fill spacing 20 μm; (b) Power 200 W, fill spacing 80 μm; (c) Power 100 W, scan rate 50 mm/s
Type Ⅲ, typeⅡ and typeⅠwere observed with power increasing, scan rate decreasing and fill spacing decreasing. Fig.4 shows the surface behavior diagram at various laser powers, scan rate and fill spacing. The results suggest that typeⅠcan be avoided by lowering laser power, increasing scan rate and increasing fill spacing. And type Ⅲ can be avoided opposite to type . When fill spacing was 10 μm, type Ⅱ can be obtained at scan rate of 50-350 mm/s with power control and fill spacing of 40 μm. The range of scan rate which can obtain type Ⅱ was 10- 150 mm/s.
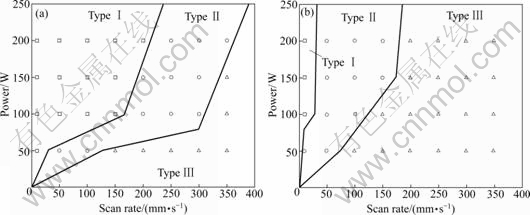
Fig.4 Process windows for various laser parameters: (a) Fill spacing 10 μm; (b) Fill spacing 40 μm
3.2 Coating thickness with various laser conditions
3.2.1 Maximum coating thickness
The maximum coating thickness is shown in Fig.5. When one or more layers should be fabricated, the maximum thickness determines the powder layer thickness which can be leveled to upper layer. The balling appeared with increasing power or lowering scan rate and then the maximum coating thickness increased smoothly. Rapidly increased coating thickness was observed with much higher power and much lower scan rate. Elevating power or lowering scan rate enhanced energy input and enhanced energy input led to higher temperatures and consequently particles around laser beam were melted. With much higher energy input, heat affected even larger zone and a lager amount of melting occurred and balls were formed with diminishing surfacing energy[8-9]. Consequently, the maximum coating thickness increased rapidly.
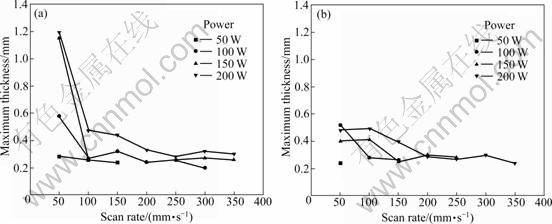
Fig.5 Maximum coating thickness for various laser parameters: (a) Fill spacing 10 μm; (a) Fill spacing 40 μm
3.2.2 Average coating thickness
Average coating thickness of type Ⅱ is shown in Fig.6. When fill spacing is 10 μm, type Ⅱ can be obtained at wide scan rate and fill spacing of 40 μm. The range of scan rate which can obtain typeⅡ was limited. Average coating thickness was 150-220 μm when fill spacing was 10 μm. And average coating thickness was 180-270 μm at fill spacing of 40 μm. The reason why average coating thickness at fill spacing of 10 μm was lower and more homogeneous than that of fill spacing of 40 μm is overlap rate. Lower fill spacing increased overlap rate and overlap rate increase caused laser polishing, which can improve surface quality of laser sintered or laser melted part[10]. When powders were melted by laser energy, pre-solidicated parts were affected by laser energy and their quality increased.
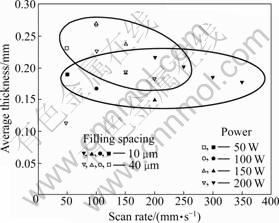
Fig.6 Average coating thickness for various laser parameters
4 Conclusions
1) Balling can be avoided by lowering laser power, increasing scan rate and increasing fill spacing and no connection can be avoided opposite to balling.
2) With elevating power or lowering scan rate, the maximum coating thickness increases smoothly, and much higher power and slower scan rate cause rapid increase of maximum coating thickness.
3) Average coating thickness at fill spacing of 10 μm is lower and more homogeneous than that at fill spacing of 40 μm.
Acknowledgement
This work was partially supported by the Korea Research Foundation Grant funded by the Korean Government (MOEHRD, Basic Research Promotion Fund) and grants-in-aid for the National Core Research Center Program from MOST/KOSEF.
References
[1] yadroitsev I, bertrand P h, Smurov I. Parametric analysis of the selective laser melting process [J]. Applied Surface Science, 2007, 253: 8064-8069.
[2] maeda K, childs T H C. Laser sintering (SLS) of hard metal powders for abrasion resistant coatings [J]. Journal of Materials Processing Technology, 2004, 149: 609-615.
[3] morgan R, Sutcliffe c j, o’neill w. Density analysis of direct metal re-melted 316L stainless steel cubic primitives [J]. Journal of Materials Science, 2004, 39: 1195-1205.
[4] jendrzejewski r, navas c, conde a, de damborenea j j, sliwinski g. Properties of laser-cladded satellite coatings prepared on preheated chromium steel [J]. Mater Design, 2008, 29: 187-192.
[5] Cheng y C, Guo Z x, Liu Y h, xie q q, wang z, hu j d, yao y. Characteristics of cobalt-based alloy coating on tool steel prepared by powder feeding laser cladding [J]. Optics & Laser Technology, 2007, 39: 1544-1550.
[6] van elsen m, al-bender f, kruth j p. Application of dimensional analysis to selective laser melting [J]. Rapid Prototyping Journal, 2008, 14(1): 15-22.
[7] Quintino L, costa a, miranda r, yapp d, kumar v, kong c j. Welding with high power fiber lasers—A preliminary study [J]. Mater Design, 2007, 28: 1231-1237.
[8] gu D d, shen y. Balling phenomena during direct laser sintering of multi-component Cu-based metal powder [J]. Journal of Alloys and Compounds, 2007, 432: 163-166.
[9] gusarov A V, yadroitsev i, bertrand p h, smurov i. Heat transfer modeling and stability analysis of selective laser melting [J]. Applied Surface Science, 2007, 254: 975-979.
[10] Lamikiz A, Sanchez j a, lopez de lacalle l n, arana j l. Laser polishing of parts built up by selective laser sintering [J]. International Journal of Machine Tools & Manufacture, 2007, 47: 2040-2050.
Corresponding author: Young-Hoon MOON; Tel: +82-51-5102472; E-mail: yhmoon@pusan.ac.kr
DOI: 10.1016/S1003-6326(08)60378-7
(Edited by YANG Bing)