
Catalytic graphitization of polyacrylonitrile (PAN)-based carbon fibers with Fe-Cr2O3 composite coating
HUANG Zhen-hua(黄振华)1, ZHOU Hai-hui(周海晖)1, 2, PENG Qi-ling(彭奇龄)1,
CHEN Jin-hua(陈金华)1, 2, KUANG Ya-fei(旷亚非)1
1. College of Chemistry and Chemical Engineering, Hunan University, Changsha 410082, China;
2. State Key Laboratory of Chemo/Biosensing and Chemometrics, Hunan University, Changsha 410082, China
Received 28 July 2009; accepted 30 October 2009
Abstract: The process of electrodepositing Fe-Cr2O3 composite coating on polyacrylonitrile (PAN)-based carbon fibers and its catalytic graphitization were studied. Carbon fibers with and without electrodeposited Fe-Cr2O3 composite coating were heat treated at different temperatures and the structural changes were characterized by XRD, Raman spectroscopy and SEM. The results indicate that Fe-Cr2O3 composite coating exhibits a significant catalytic effect on graphitization of carbon fibers at low temperatures. When the Fe-Cr2O3-coated carbon fibers were heat treated at 1 300 °C, the interlayer spacing (d002) and ratio of relative peak area (AD/AG) reach 3.364 ? and 0.34, respectively. Whereas, the extent of graphitization of pristine carbon fibers is comparatively low even after heat treatment at 2 800 °C and the values of d002 and AD/AG are 3.414 ? and 0.68, respectively. The extent of graphitization of carbon fibers increases not only with the increase of the catalyst gross but also the Cr2O3 content in Fe-Cr2O3 coating. The catalytic effect of Fe-Cr2O3 composite coating accords with the dissolution-precipitation mechanism.
Key words: carbon fiber; catalytic graphitization; Fe-Cr2O3 coating
1 Introduction
It is well known that graphite has many unique properties, such as high electric conductivity, high thermal conductivity and high resistance to strong acid and alkali. These unique properties result in many potential applications, such as catalyst support[1-2], sensor[3-4], Li-ion battery[5] and electrochemical capacitor[6]. However, the properties of graphitic materials such as thermal and electric conductivities, and tensile modulus can be affected by the extent of graphitization. Therefore, extent of graphitization is an important parameter for the application of carbon materials. Usually, the heat treatment temperature (HTT) of carbon was above 3 000 °C. Adding catalysts could accelerate the graphitization of carbon[7-8], which is called catalytic graphitization. In previous works, transition metals have been used as catalyst precursors for graphitization. WEISWEILER et al[9] studied the graphitization of monolithic glasslike carbon with metals as catalysts, and they concluded that elements such as Co, Fe, Pt, Mo, Cr and B were highly effective in catalyzing the graphitization. Lately, MALDONADO-HODAR et al[10] used various kinds of metals to produce graphite and considered that Cr and Fe seemed to be the best catalysts for graphitization of carbon aerogels. Metallic coating was easily electrodeposited on the surface of PAN-based carbon fibers, and the electrodeposited metallic coating has an obvious catalytic effect on the graphitization of the carbon fiber after high temperature treatment[11]. In order to obtain carbon fibers with high extent of graphitization at low temperatures, in this work, the catalytic graphitization of carbon fibers with electrodeposited Fe-Cr2O3 composite coating was studied. Moreover, effects of the Cr2O3 content in Fe-Cr2O3 composite coating and the deposition time of the coating on the extent of graphitization of carbon fibers were investigated.
2 Experimental
2.1 Preparation of samples
Carbon fibers used in this study were PAN-based carbon fibers (T700-12K) produced by Toray Corporation in Japan. Fe-Cr2O3 composite coatings were electrodeposited on carbon fibers using ferrous sulfate and chromic oxide as the source of iron and chromium, respectively, boric acid as buffer, sodium chloride as conductive salt and sulfamic acid as complex agent. Before electrodeposition, the carbon fibers need some appropriate pretreatments. At first, the sizing material of carbon fibers was removed by immersing carbon fibers into acetone solution for 30 min. And then the carbon fibers were rinsed by double distilled water. Afterwards, alkaline liquid (NaOH 80 g/L, Na3PO4 30 g/L and Na2CO3 15 g/L) was used to treat carbon fibers for 15 min at 60 °C. Ultrasonic vibration was applied during this process and finally the carbon fibers were rinsed by double distilled water for 5 min. The content of Cr2O3 in the Fe-Cr2O3 composite coatings was altered by changing the concentration of Cr2O3 in electrolyte. Tables 1 and 2 list the bath composition and deposition parameters, respectively. Heat treatment of the bare carbon fibers and Fe-Cr2O3-coated carbon fibers was carried out in a graphite furnace under an argon atmosphere. Temperature was increased at the rate of 40 °C /min and kept for 120 min until it reached the targeted temperature.
Table 1 Bath composition used for electrodepositing Fe-Cr2O3 composite coating (g/L)
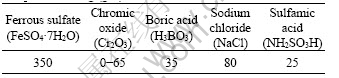
Table 2 Deposition conditions used for electrodepositing Fe-Cr2O3 composite coating
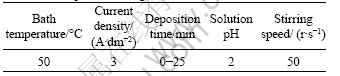
2.2 Characterization
The degree of graphitization of the fiber was investigated by powdered sample X-ray diffractometry (Cu Kα radiation, 40 kV, 200 mA). Silicon was used as a internal standard. The values of the interlayer spacing d002 and crystalline size Lc were calculated from the (002) diffraction peak according to the method described by Bragg law and Scherrer equation[12]. The degree of graphitization can be calculated by the following equation: G=(3.440-d002)/(3.440-3.354)[12-13], where G refers to the degree of graphitization (%), 3.440 represents the interlayer spacing of the fully non-graphitized carbon (?), 3.354 represents the interlayer spacing of the ideal graphite crystallite and d002
refers to the interlayer spacing derived from XRD pattern(?).
The structural changes of the PAN-based carbon fibers after high temperature heat treatment were observed by a JEOL JSM-5600LV scanning electron microscope (SEM) at an accelerating voltage of 15 kV. The extent of graphitization at different locations of the cross-section of the heat treated carbon fibers was obtained with a LABRAM-010 Raman microscopy (632.83 nm He-Ne laser, laser beam diameter 3 μm) in the rang of 1 100-1 880 cm-1.
In addition, the element distribution inside the Fe-Cr2O3-coated fiber after heat treatment was characterized by an energy dispersive analysis system of X-ray (EDS) attached to the JSM-5600LV SEM.
3 Results and discussion
3.1 Effect of heat treatment temperature
Fig.1 indicates the SEM images of bare carbon fiber and carbon fiber coated with Fe-Cr2O3. As shown in Fig.1(b), Fe-Cr2O3 composite coating can be observed on the carbon fiber substrate after electrochemical deposition. The surface of the Fe-Cr2O3 composite coating is very rough and the Cr2O3 particulates disperse in the coating. Fig.2 shows the XRD pattern and Raman spectra of carbon fibers without coating at different heat treatment temperatures (HTTs). In Fig.2(a), the (002)
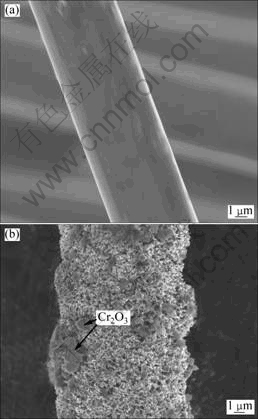
Fig.1 SEM images of bare carbon fiber (a) and carbon fiber coated with Fe-Cr2O3 composite coating (b)
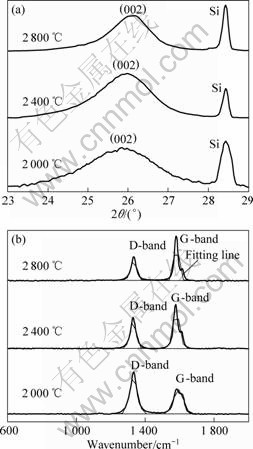
Fig.2 XRD patterns (a) and Raman spectra (b) of PAN-based carbon fibers heat treated at different temperature
peak shifts right distinctly when the HTT increases. Moreover, the broad profile gets sharper increasingly. Fig.2(b) exhibits two relatively broad Raman bands at about 1 350 cm-1 (D-band) and 1 580 cm-1 (G-band), which are responsible for the disordered carbon atoms and the graphitic phase, respectively[14-15]. As shown in Fig.2(b), the shapes of D and G bands become sharper and D-band is gradually weakened with increasing HTT. However, G-band is distinctly strengthened, because graphite component increases and disordered carbon decreases contrarily.
Table 3 lists the values of d002, the thickness of crystallized carbon layers (Lc) and Raman spectroscopic parameters of carbon fibers after heat treatment at different HTTs. The closer the value of d002 is to 3.354 ? and the larger the value of Lc is, the higher the extent of graphitization is. Moreover, a smaller value of the relative peak area (AD/AG) also means a higher extent of graphitization[15-16]. It can be seen from Table 3 that the ratio of AD/AG and the wavenumber of D and G bands all decrease with increasing HTT. From Fig.2 and Table 3, it is noted that the extent of graphitization increases with the increase of HTT. However, PAN-based carbon fibers without Fe-Cr2O3 composite coating are hard to be
Table 3 Effects of HTT on d002, Lc and Raman spectroscopic parameters of PAN-based carbon fibers
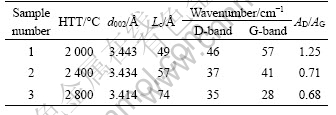
graphitized even being heat treated at 2 000 °C. When the HTT is raised to 2 800 °C, the value of Lc is just 74 ? and the value of d002 reaches 3.414 ?, for instance. The XRD patterns and Raman spectra of PAN-based carbon fibers coated with Fe-Cr2O3 (Cr2O3 20 g/L, 15 min) through heat treatment at different temperatures are shown in Fig.3. The (002) peak shifts right and becomes sharper as the HTT increases (Fig.3(a)). Raman spectroscopic parameters (wavenumber and the ratio of peak area) are obtained after curve fitting, as shown in Fig.3(b). Table 4 lists the values of d002, Lc and Raman spectroscopic parameters, and shows that the value of Lc increases with the increase of HTT, but the value of d002 decreases with the increase of HTT. When the HTT is raised to 1 300 °C, the value of Lc is 271 ? and the value of d002 reaches 3.364 ? that is very close to 3.354 ?
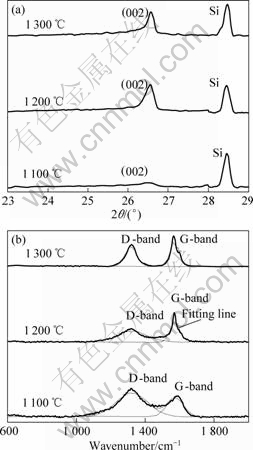
Fig.3 XRD patterns (a) and Raman spectra (b) of PAN-based carbon fibers coated with Fe-Cr2O3 composite coating
for hexagonal graphite. From Table 4, it can be found that the wavenumber and the ratio of peak area (AD/AG) in Raman spectra decrease with the increase of HTT. It is also noted that the G-band wavenumber for Fe-Cr2O3 coated carbon fibers heat treated at 1 300 °C is 22 cm-1, which is close to the value of highly oriented graphite (21 cm-1)[16]. Although the HTTs of Fe-Cr2O3 coated carbon fibers are lower than those of bare carbon fibers, Fe-Cr2O3 coated carbon fibers have higher extent of graphitization than bare carbon fibers, which shows that Fe-Cr2O3 coating has catalytic effect on graphitization of PAN-based carbon fibers.
Table 4 Effects of HTT on d002, Lc and Raman spectroscopic parameters of PAN-based carbon fibers coated with Fe-Cr2O3 composite coating
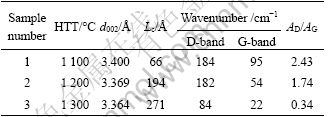
3.2 Catalytic effect of Cr2O3 content in Fe-Cr2O3 composite coating
The Cr2O3 content in the Fe-Cr2O3 composite coating was altered by changing the concentration of Cr2O3 in electrolyte. Table 5 lists the effects of Cr2O3 concentration on the values of d002, Lc and Raman spectroscopic parameters of Fe-Cr2O3 coated carbon fibers (electrodeposited for 15 min) heat treated at 1 200 °C. It is noted that d002 and AD/AG decrease with the increase of Cr2O3 concentration. A low Lc of 141 ? is obtained for carbon fibers coated with pure Fe. However, with the increase of Cr2O3 concentration in the electrolyte, there is an evident increase in Lc. Simultaneously, the wavenumbers of peaks decrease. As the Cr2O3 concentration rises to 50 g/L, Lc reaches 288 ?, d002 and AD/AG reach 3.357 ? and 0.60, respectively. The XRD and Raman spectra results show that much better graphitization can be achieved for carbon fibers with higher Cr2O3 content. In general, the catalyst content has a significant effect on graphitization at the same HTT[17-18]. Table 6 lists the changes of XRD data (d002 and Lc) and Raman spectroscopic parameters of carbon fibers with different Fe-Cr2O3 deposition time (Cr2O3 20 g/L) at HTT of 1 200 °C. It is clear that the catalytic graphitization effect of PAN-based carbon fibers increases with the increase of Fe-Cr2O3 deposition time, i.e. Fe-Cr2O3 content. Therefore, the extent of graphitization is related not only to Cr2O3 content but also to catalyst gross. As the deposition time increases from 5 min to 25 min, a decrease in AD/AG value, from 2.28 to 1.24, was found. However, a significant decrease of AD/AG from 1.79 to 0.60 was obtained, when the Cr2O3 concentration increases from 10 g/L to 50 g/L. Above experimental results show that Cr2O3 content has a much more prominent catalytic effect on the graphitization of carbon fibers.
Table 5 Effects of Cr2O3 concentration on d002, Lc and Raman spectroscopic parameters of Fe-Cr2O3-coated PAN-based carbon fibers heat treated at 1 200 °C
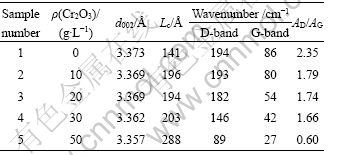
Table 6 Effects of deposition time on d002, Lc and Raman spectroscopic parameters of Fe-Cr2O3-coated PAN-based carbon fibers heat treated at 1 200 °C
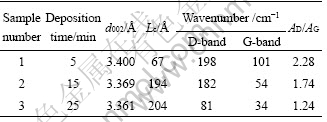
3.3 Catalytic mechanism for graphitization
Fig.4(a) and Fig.4(b) show the SEM images of the cross-section of original carbon fiber and carbon fiber coated with catalyst after heat treatment at 1 200 °C, respectively. The cross-section of the original carbon fiber has a flat surface. While being heat treated at 1 200 °C, the cross-section of fiber changes from a flat surface to a rough surface after catalytic graphitization. It is also observed that the outside of the carbon fiber is rougher than the center and the untransformed portion of the carbon fiber with a typical flat can be found in the centre (Fig.4(b)), indicating that the catalytic graphitization process is incomplete. It is speculated that Fe and Cr2O3 in the composite coating are responsible for the catalytic graphitization. In order to verify the effect of Fe and Cr2O3, the distribution location of Fe and Cr2O3 on cross-section of fiber is detected by energy dispersive spectrometry (EDS). A linear scanning was carried out along the arrow in Fig.4(b). The EDS results in Fig.4(c) show that Fe and Cr mainly remain in the rough layer, which implies that most of the coatings move towards the center of cross-section but Fe and Cr do not arrive at the center. The reason for these results is that the process of catalytic graphitization proceeds from the outer
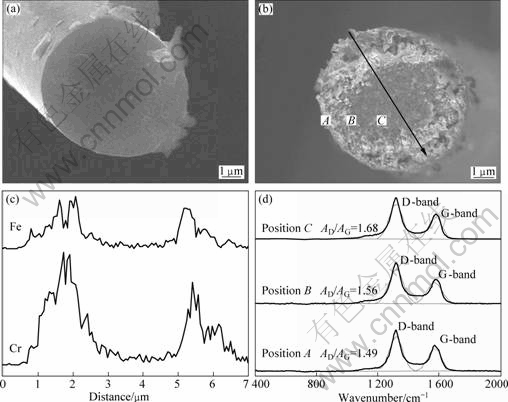
Fig.4 SEM images of cross-section of original carbon fiber (a), carbon fiber coated with Fe-Cr2O3 (b), corresponding EDS (c) and corresponding Raman spectra (d)
surface where the fiber contacts the catalyst[19]. Fe and Cr are ejected from the original composite coating and move towards the carbon region and a graphitic phase precipitates behind the catalyst following the well-known dissolution-precipitation mechanism. According to the mechanism, the extent of graphitization should become higher increasingly from the centre to the outer surface. Raman analysis was carried out on the, positions A, B and C, respectively, in Fig.4(b). The Raman spectra results are shown in Fig.4(d). The AD/AG values of 1.49, 1.56 and 1.68 corresponding to positions A, B and C, respectively, indicate that the outer portion has a higher extent of graphitization. Apparently, the phenomena are also consistent with the dissolution-precipitation mechanism.
4 Conclusions
1) The Fe-Cr2O3 composite coating was electrodeposited on the surface of PAN-based carbon fibers by galvanostatic deposition method. Much better graphitization can be achieved for carbon fibers at lower heat treatment temperature when the Fe-Cr2O3 catalyst is present. The Fe-Cr2O3-coated carbon fiber treated at 1 300 °C has a higher extent of graphitization than the bare carbon fiber treated at 2 800 °C, and their d002 values are 3.364 and 3.414 ?, respectively.
2) Not only the Cr2O3 content but also the catalyst gross (deposition time) has an important effect on the catalytic graphitization of the Fe-Cr2O3-coated carbon fiber. The extent of graphitization of the Fe-Cr2O3 coated carbon fibers increases with the increase of Fe-Cr2O3 deposition time at the same HTT. Moreover, the extent of graphitization of the Fe-Cr2O3-coated carbon fibers increases with the increase of Cr2O3 content in the Fe-Cr2O3 composite coating at the same HTT. As the Cr2O3 concentration in electrolyte rises from 0 to 50 g/L, the value of AD/AG decreases from 2.35 to 0.60, and the Lc rises from 141 to 288 ?.
3) The catalytic graphitization of Fe-Cr2O3 coating follows the dissolution-precipitation mechanism. The catalytic graphitization phenomenon first occurs along the outside of the fiber and the extent of graphitization becomes high increasingly from the centre to the outer surface.
References
[1] JAYASRI D, NSRAYANAN S S. Electrocatalytic oxidation and amperometric determination of BHA at graphite-wax composite electrode with silver hexacyanoferrate as electrocatalyst [J]. Sensors and Actuators B, 2006, 119(1): 135-142.
[2] JEONG S H, LIM D C, BOO J H, LEE S B, HWANG H N, HWANG C C, KIM Y D. Interaction of silver with oxygen on sputtered pyrolytic graphite [J]. Applied Catalysis A, 2007, 320: 152-158.
[3] SANTINI A O, OLIVEIRA J E, PEZZA H R, PEZZA L. A new potentiometric ibuprofenate ion sensor immobilized in a graphite matrix for determination of ibuprofen in tablets [J]. Microchemical Journal, 2006, 84(1/2): 44-49.
[4] PEREIRA-RODRIGUES N, COFRE R, ZEGAL J H, BEDIOUI F. Electrocatalytic activity of cobalt phthalocyanine CoPc adsorbed on a graphite electrode for the oxidation of reduced l-glutathione (GSH) and the reduction of its disulfide (GSSG) at physiological pH [J]. Bioelectrochemistry, 2007, 70(26): 147-154.
[5] NICOLAUS L R, PRASHANT N K. Synthesis and characterization of electrochemically active graphite-silicon-tin composite anodes for Li-ion applications [J]. Journal of Power Sources, 2007, 164(2): 829-838.
[6] WANG H Y, YOSHIO M. Graphite, a suitable positive electrode material for high-energy electrochemical capacitors [J]. Electrochemistry Communications, 2006, 8(9): 1481-1486.
[7] ZALDIVAR R J, RELLICK G S. Some observations on stress graphitization in carbon-carbon composites [J]. Carbon, 1991, 29 (8): 1155-1163.
[8] OYA A, OTANI S. Catalytic graphitization of carbons by various metals [J]. Carbon, 1979, 17(2): 131-137.
[9] WEISWEILER W, SUBRAMANIAN N, TERWIESCH B. Catalytic influence of metal melts on the graphitization of monolithic glasslike carbon [J]. Carbon, 1971, 9(6): 755-761.
[10] MALDONADO-HODAR F J, MORENO-CASTILLA C, RIVERA- UTRILLA J. Catalytic graphitization of carbon aerogels by transition metals [J]. Langmuir, 2000, 16(9): 4367-4373.
[11] ZHOU Hai-hui, YU Qiang, PENG Qi-ling, WANG Hua, CHEN Jin-hua, KUANG Ya-fei. Catalytic graphitization of carbon fibers with electrodeposited Ni-B alloy coating [J]. Materials Chemistry and Physics, 2008, 110(2/3): 434-439.
[12] ZOU Lin-hua, HUANG Bai-yun, HUANG Yong, HUANG Qi-zhong, WANG Chang-an. An investigation of heterogeneity of the degree of graphitization in carbon-carbon composites [J]. Materials Chemistry and Physics, 2003, 82(3): 654-662.
[13] AWASTHI S, WOOD J L. C/C composite materials for aircraft brakes [J]. Advanced Ceramic Materials, 1988, 3: 449-451.
[14] RUSOP M, SOGA T, JIMBO T, UMENO M, SHARON M. Semiconducting amorphous camphoric carbon nitride thin films [J]. Surface Review and Letters, 2005, 12(4): 587-595.
[15] WANG Y, ALSMEYER D C, MCCREERY R L. Raman spectroscopy of carbon materials: Structural basis of observed spectra [J]. Chem Mater, 1990, 2(5): 557-563.
[16] TZENG S S. Catalytic graphitization of electroless Ni-P coated PAN-based carbon fibers [J]. Carbon, 2006, 44 (10): 1986-1993.
[17] OYA A, YAMASHITA R, OTANI S. Catalytic graphitization of carbons by boron [J]. Fuel, 1979, 58(7): 495-500.
[18] MOCHIDA I, OHTSUBO R, TAKESHITA K, MARSH H. Catalytic graphitization of non-graphitizable carbon by chromium and manganese oxides [J]. Carbon, 1980, 18(2): 117-123.
[19] TZENG S S, LIN Y H. The role of electroless Ni-P in the catalytic graphitization of PAN-based carbon fibers [J]. Carbon, 2008, 46(3): 544-561.
(Edited by LI Xiang-qun)
Foundation item: Project(2006CB600903) supported by the National Basic Research Program of China
Corresponding author: KUANG Ya-fei; Tel: +86-731-88821874; Fax: +86-731-88713642; E-mail: yafeik@163.com
DOI: 10.1016/S1003-6326(09)60314-9