
Effect of annealing treatment on electrochemical property of
LiNi0.5Mn1.5O4 spinel
ZHANG Bao(张 宝), WANG Zhi-xing(王志兴), GUO Hua-jun(郭华军)
School of Metallurgical Science and Engineering, Central South University, Changsha 410083, China
Received 28 March 2006; accepted 25 September 2006
Abstract: LiNi0.5Mn1.5O4 was prepared under different cooling conditions. The electrochemical properties of LiNi0.5Mn1.5O4 prepared under different cooling conditions were investigated. The results show that LiNi0.5Mn1.5O4 synthesized with or without annealing treatment has similar X-ray diffraction patterns that can be indexed to cubic spinel structure. The mass loss occurring above 650 ℃ during the heating process can be mostly gained during the cooling process. LiNi0.5Mn1.5O4 synthesized with an annealing treatment exhibits almost one voltage plateau at around 4.7 V and higher capacity with a quick fading upon cycling, whereas LiNi0.5Mn1.5O4 synthesized without annealing treatment shows two voltage plateaus at around 4.1 and 4.7 V and superior capacity retention upon cycling both at rates of 1/7C and 1C, though the capacity is not high.
Key words: lithium ion battery; cathode material; LiNi0.5Mn1.5O4; annealing treatment; electrochemical property
1 Introduction
The exponential growth in the electronics industry has led to an increasing demand for light mass power sources with high energy density and power capability[1]. This demand has been satisfied largely by the advent of rechargeable lithium-ion batteries[2-4]. LiCoO2 is the first commercial cathode material for lithium ion batteries[5]. However, the poor resource and high-cost of cobalt has limited the application of LiCoO2 as a cathode material for lithium ion batteries for electric vehicles. Among various cathode materials, LiMn2O4 is the most promising one and has attracted wide attention because of its low cost, nontoxicity and potential good electrochemical performance. However, its quick capacity loss on cycling becomes an obstruction to practical use, probably due to the structural transition and Mn3+ dissolution into the electrolyte, which destroy the structure and surface of the electrode[6-7]. A number of investigations have been involved in order to improve the cycleability of LiMn2O4. Doping is often used[8-10].
Recently, many researches groups have investigated the transition-metal-substituted spinel materials (LiMxMn2-xO4, M=Cr, Co, Fe, Ni, Cu) with high-voltage
plateaus above 4.5 V[11-15]. Among these materials, LiNi0.5Mn1.5O4 is the most promising and attractive one because of its good cyclic property and relatively high capacity with a plateau at around 4.7 V[13,16].
It is proved that the strategy of slowly cooling or annealing treatment at low temperature is very necessary to optimize the oxygen content of LiNi0.5Mn1.5O4 because the oxygen loss of LiNi0.5Mn1.5O4 occurs when it is prepared at high temperature[14,16-17]. For example, OHZUKU et al[17] synthesized LiNi0.5Mn1.5O4 employing an annealing treatment at 700 ℃ in air after the high-temperature calcination at temperature as high as 1 000 ℃. WU et al[16] employed an annealing treatment at 600℃ to reduce oxygen loss from LiNi0.5Mn1.5O4 prepared by sol-gel method. They have both proved that the annealing process can greatly suppress the voltage plateau at around 4.1 V and the capacity of the plateau at around 4.7 V is enhanced. But the effect of annealing treatment on the electrochemical property such as cyclic performance has not been investigated. In this paper, LiNi0.5Mn1.5O4 was synthesized by solid-state reaction, and the effect of annealing treatment on the rate capability and cycling performance of LiNi0.5Mn1.5O4 was investigated.
2 Experimental
Appropriate amounts of Li2CO3, NiO and electrolytic MnO2 were initially ground in mortar, and then the mixture was thoroughly ball-milled. Subsequently the mixed precursors were calcined at 950℃ for 12 h, one sample was directly cooled to room temperature, the other sample was followed by an annealing treatment at 600 ℃ for 24 h in air.
The powder X-ray diffraction (XRD, Rint-2000, Rigaku) measurement using
radiation was employed to identify the crystalline phase of the synthesized material. Thermal gravimetric analysis of the LiNi0.5Mn1.5O4 powders was performed with a TA instrument (SDT Q600). The powders were heated and cooled at 2 ℃/min in a constant flow of extra dry air.
The electrochemical characterizations were performed using CR2025 coin-type cell. For cathode fabrication, the prepared powders were mixed with 20% (mass fraction) of carbon black and 10% (mass fraction) of polyvinylidene fluoride in N-methyl pyrrolidinone until slurry was obtained. And then, the blended slurries were pasted onto an aluminum current collector, and the cathode was dried at 80 ℃ for 1 d in vacuum. The test cell consisted of the cathode and lithium foil anode separated by a porous polypropylene film, and 1 mol/L LiPF6 in EC?EMC?DMC (1?1?1 in volume) was used as the electrolyte. The assembly of the cells was carried out in a dry Ar-filled glove box. The cells were charged and discharged over a voltage range from 3.5 to 4.9 V vs Li/Li+ electrode at room temperature.
3 Results and discussion
Fig.1 shows the XRD patterns of LiNi0.5Mn1.5O4 powders. The patterns of both samples with and without annealing treatment are similar. All peaks can be indexed to the cubic spinel structure with a space group of Fd3m. In addition, relatively wide and weak peaks of the sample without annealing treatment are clearly observed in Fig.1(a), while sharp and intensive peaks of the sample with annealing treatment are seen in Fig.1(b).
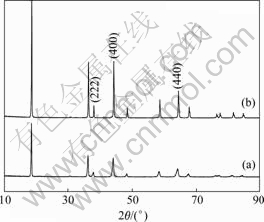
Fig.1 XRD patterns of LiNi0.5Mn1.5O4 powders calcined at 950℃: (a) Without annealing treatment; (b) With annealing treatment
The TG measurement of the sample calcined at 900℃ for 12 h followed by an annealing treatment at 600 ℃ for 24 h in temperature range from room temperature to 950 ℃ is shown in Fig.2. It can be seen that the mass loss during heating process can be mostly gained during cooling process. This result is in good agreement with that in Ref.[14] where ZHONG et al reported that LiNi0.5Mn1.5O4 would lose oxygen and disproportionate to a spinel and LixNi1-xO when heated above 650 ℃, which can be written as
LiNi0.5Mn1.5O4→aLixNi1-xO+bLiNi0.5-yMn1.5-yO4+cO2 (1)
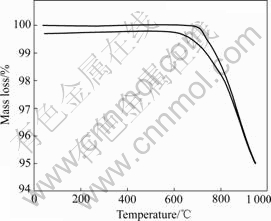
Fig.2 TG measurement on sample calcined at 900 ℃ for 12 h followed by annealing treatment at 600℃ for 24 h in air
Furthermore, this reaction can be reversibly proceeded during the slow cooling or annealing process after high-temperature calcination. It is also clear that the mass loss occurred above 650 ℃ and the mass gain stopped below 570 ℃, which suggest the annealing treatment temperature set at 600 ℃ is suitable to optimize the oxygen content of LiNi0.5Mn1.5O4 in our experiment.
Charge—discharge curves of LiNi0.5Mn1.5O4 cycled between 3.5 and 4.9 V at rates of 1/7C and 1C are shown in Fig.3. For the 1/7C rate, LiNi0.5Mn1.5O4 was charged and discharged at the same rate of 1/7C; in the case of the 1C rate, LiNi0.5Mn1.5O4 was charged at the rate of 1/7C and discharged at the rate of 1C. The annealing treatment has a significant effect on the electrochemical performance of LiNi0.5Mn1.5O4 powders. The sample synthesized with an annealing treatment almost exhibits one voltage plateau at around 4.7 V based on Ni2+/Ni4+ redox couple, whereas the sample synthesized without annealing treatment shows a plateau at around 4.1 V based on Mn3+/Mn4+ redox couple in addition to the 4.7 V plateau, and delivers much smaller capacity, which is due to the oxygen loss at high temperature, leading to the appearance of impurity NiO or LixNi1-xO and the decrease of manganese oxidation state from Mn4+ to Mn3+[14, 18], as clearly seen in Fig.3. The peaks of impurity which are usually placed close to the lines (222), (400) and (440) of the spinel are not observed in Fig.1(a), which is probably due to the overlap of peaks of spinel phase and impurity because of widening of peaks. However, the sample without annealing treatment exhibits better rate capability than that of the sample with an annealing treatment. When the sample without annealing treatment is discharged at 1C, the voltage plateau and capacity are only little reduced, when the sample with an annealing treatment is discharged at 1C, the voltage plateau is greatly reduced, and the capacity is drastically decreased from 128 to 108 mA?h/g. This observed behavior is probably attributed to higher conductivity of the sample without annealing treatment. The high electronic conductivity in oxides is most frequently observed in the mixed valency semiconductor [12,19]. For spinels such as Mn3O4[19-20], NiMn2O4[19] and LiMn2O4[21], fast electronic transport is attained by thermally activated, small polaron hopping between Mn3+ and Mn4+. In the case of the sample synthesized without annealing treatment, the oxygen loss results in the presence of Mn3+/Mn4+ so as probably to improve its conductivity. Besides, the behavior may also be associated with different structures of the samples that are hard to be differentiated based on the XRD patterns. According to Ref.[22], the annealing treatment will result in the transformation of an disorder structure to an order structure for LiNi0.5Mn1.5O4, and the disorder LiNi0.5Mn1.5O4 shows better electrochemical perfor- mance, especially for the rate capability.
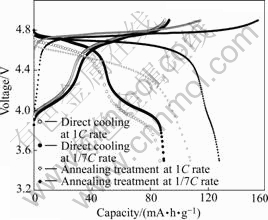
Fig.3 Charge—discharge curves of LiNi0.5Mn1.5O4 calcined at 950 ℃ and rates of 1/7C and 1C
Fig.4 shows the cycling performance of LiNi0.5Mn1.5O4 cycled between 3.5 and 4.9 V. As seen in Fig.4, the sample synthesized with an annealing treatment delivers higher capacity but with a quick fading at both rates of 1/7C and 1C. However, the sample synthesized without annealing treatment exhibits superior capacity retention upon cycling, though the capacity is low, and the discharge capacity remains invariable even after 47 cycles at the rate of 1/7C. Furthermore, for the sample without annealing treatment which is cycled at the rate of 1C after 47 cycles, shows superior capacity retention, though a little decrease of the capacity when the rate increases.
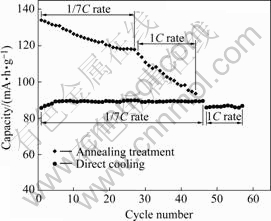
Fig.4 Electrochemical cycling performances of LiNi0.5Mn1.5O4 calcined at 950 ℃ and rates of 1/7C and then 1C
From the above results, it can be concluded that direct cooling can improve the rate capability and cycling performance of LiNi0.5Mn1.5O4, but reduce the capacity, especially the capacity of the voltage plateau at around 4.7 V because of the oxygen loss.
4 Conclusions
1) LiNi0.5Mn1.5O4 is prepared under different cooling conditions. X-ray diffraction(XRD) patterns show that LiNi0.5Mn1.5O4 synthesized under different cooling conditions has the same crystal structure. TG measurement indicates that the mass loss occurring above 650 ℃ during heating process can be mostly gained during cooling process.
2) The electrochemical property of LiNi0.5Mn1.5O4 prepared under different cooling conditions is evaluated. The capability of LiNi0.5Mn1.5O4 synthesized without annealing treatment is better than that of LiNi0.5Mn1.5O4 synthesized with annealing treatment. Meanwhile, LiNi0.5Mn1.5O4 synthesized with annealing treatment exhibits higher capacity but with a quick fading upon cycling, whereas LiNi0.5Mn1.5O4 synthesized without annealing treatment shows superior capacity retention upon cycling both at rates of 1/7C and 1C, though the capacity is not high and the 4.1 V plateau can be clearly seen.
References
[1] NISHI Y. Lithium ion secondary batteries: Past 10 years and the future [J]. J Power Sources, 2001, 100(1/2): 101-106.
[2] PARK S H, PARK K S, MOON S S, SUN Y K, NAHM K S. Synthesis and electrochemical characterization of Li1.02Mg0.01- Mn1.9O3.99S0.01 using sol-gel method [J]. J Power Sources, 2001, 92(1/2): 244-249.
[3] KIM Y S, KANOH H, HIROTSU T, OOI K. Chemical bonding of ion-exchange sites in spinel-type manganese oxides Li1.33Mn1.67O4 [J]. Mater Res Bull, 2002, 37(2): 391-396.
[4] CHEBIAM R V, KANNAN A M, PRADO F, MANTHIRAM A. Comparison of the chemical stability of the high energy density cathodes of lithium-ion batteries [J]. Electrochemistry Communications, 2001, 3(11): 624-627.
[5] TANAKA T, OHTA K, ARAI N. Year 2000 R&D status of large-scale lithium ion secondary batteries in the national project of Japan [J]. J Power Sources, 2001, 97/98: 2-6.
[6] THACKERAY M M, SHAO-HORN Y, KAHAIAN A J, KEPLER K D, SKINNER E, VAUGHEY J T, HACKNEY S A. Structural fatigue in spinel electrodes in high voltage (4V) Li/LixMn2O4 cells [J]. Electrochemical and Solid State Letters, 1998, 1(1): 7-9.
[7] THACKERAY M M, JOHNSON C S, KAHAIAN A J, KEPLER K D, VAUGHEY J T, SHAO-HORN Y, HACKNEY S A. Stabilization of insertion electrodes for lithium batteries [J]. J Power Sources, 1999, 81/82: 60-66.
[8] LI G H, IKUTA H, UCHIDA T, WAKIHARA M. The spinel phases LiMyMn2-yO4(M=Co, Cr, Ni) as the cathode for rechargeable lithium ion batteries [J]. J Electrochem Soc, 1996, 143(1): 178-182.
[9] WANG Z, IKUTA H, UCHIMOTO Y, WAKIHARA M. Preparation and electrochemical properties of stoichiometric and non- stoichiometric LiCoxMn2-xO4-δ [J]. J Electrochem Soc, 2003, 150(9): A1250-A1254.
[10] KANNAN A M, MANTHIRAM A. Surface/chemically modified LiMn2O4 cathodes for lithium-ion batteries [J]. Electrochemical and Solid-State Letters, 2002, 5(7): A167-A169.
[11] SIGALA C, GUYOMARD D, VERBAERE A, PIFFARD Y, TOURNOUX M. Positive electrode materials with high operating voltage for lithium batteries: LiCryMn2-yO4 (0≤y≤1) [J]. Solid State Ionics, 1995, 81(3/4): 167-170.
[12] KAWAI H, NAGATA M, TUKAMOTO H, WEST A R. A novel cathode Li2CoMn3O8 for lithium ion batteries operating over 5 V [J]. J Mater Chem, 1998, 8(4): 837-839.
[13] OHZUKU T, TAKEDA S, IWANAGA M. Solid-state redox potentials for Li[Me1/2Mn3/2]O4 (Me:3d-transition metal) having spinel-framework structures: A series of 5 V materials for advanced lithium-ion batteries [J]. J Power Sources, 1999, 81/82: 90-94.
[14] ZHONG Q, BONAKDARPOUR A, ZHANG M, GAO Y, DAHN J R. Synthesis and electrochemistry of LiNixMn2-xO4 [J]. J Electrochem Soc, 1997, 144(1): 205-213.
[15] EIN-ELI Y, HOWARD W F J R. LiCuxⅡCuyⅢMn[2-(x+y)]Ⅲ,ⅣO4: 5V cathode materials [J]. J Electrochem Soc, 1997, 144(8): L205-L207.
[16] WU X, KIM S B. Improvement of electrochemical properties of LiNi0.5Mn1.5O4 spinel [J]. J Power Sources, 2002, 109(1): 53-57.
[17] OHZUKU T, ARIYOSHI K, YAMAMOTO S. Synthesis and characterization of Li[Ni1/2Mn3/2]O4 by two-step solid state reaction [J]. J Ceram Soc Jpn, 2002, 110(5): 501-505.
[18] AMINE K, TUKAMOTO H, YASUDA H, FUJITA Y. Preparation and electrochemical investigation of LiMn2-xMexO4 (Me: Ni, Fe, and x=0.5, 1) cathode materials for secondary lithium batteries [J]. J Power Sources, 1997, 68(2): 604-608.
[19] KAWAI H, NAGATA M, TUKAMOTO H, WEST A R. High-voltage lithium cathode materials [J]. J Power Sources, 1999, 81/82: 67-72.
[20] DORRIS S E, MASON T O. Electrical properties and cation valencies in Mn3O4 [J]. J Am Ceram Soc, 1988, 71(5): 379-385.
[21] MASSAROTTI V, CAPSONI D, BINI M, CHIODELLI G, AZZONI C B, MOZZATI M C, PALEARI A. Electric and magnetic properties of LiMn2O4- and Li2MnO3-type oxides [J]. J Solid State Chem, 1997, 131(1): 94-100.
[22] ALCANTARA R, JARABA M, LAVELA P, TIRODO J L. Changes in the local structure of LiMgyNi0.5-yMn1.5O4 electrode materials during lithium extraction [J].Chem Mater, 2004, 16(8): 1573-1579.
Foundation item: Project supported by the Scientific Research Foundation for the Returned Overseas Chinese Scholar
Corresponding author: ZHANG Bao; Tel: +86-731-8836357; E-mail: zhangb@qianlong.com
(Edited by CHEN Wei-ping)