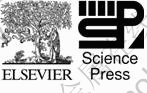
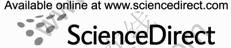
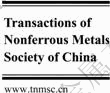
Trans. Nonferrous Met. Soc. China 22(2012) 1817-1823
Synthesis of Y2O3 particle enhanced Ni/TiC composite on TC4 Ti alloy by laser cladding
ZHANG Ke-min1, ZOU Jian-xin2, LI Jun1, YU Zhi-shui1, WANG Hui-ping1
1. School of Materials Engineering, Shanghai University of Engineering Science, Shanghai 201620, China;
2. Shanghai Engineering Research Center of Magnesium Materials and Applications,
School of Materials Science and Engineering, Shanghai Jiao Tong University, Shanghai 200240, China
Received 25 November 2011; accepted 11 June 2012
Abstract: A Y2O3 particle enhanced Ni/TiC composite coating was fabricated in-situ on a TC4 Ti alloy by laser surface cladding. The phase component, microstructure, composition distribution and properties of the composite layer were investigated. The composite layer has graded microstructures and compositions, due to the fast melting followed by rapid solidification and cooling during laser cladding. The TiC powders are completely dissolved into the melted layer during melting and segregated as fine dendrites when solidified. The size of TiC dendrites decreases with increasing depth. Y2O3 fine particles distribute in the whole clad layer. The Y2O3 particle enhanced Ni/TiC composite layer has a quite uniform hardness along depth with a maximum value of HV1380, which is 4 times higher than the initial hardness. The wear resistance of the Ti alloy is significantly improved after laser cladding due to the high hardness of the composite coating.
Key words: TC4 Ti alloy; Ni/TiC composite; Y2O3; laser cladding; hardness; surface modification
1 Introduction
Ti and Ti alloys have been widely used in aerospace, biomedical and automobile industries. The advantages of Ti alloys over other metallic materials lie in their good combination of mechanical and chemical properties, such as high stiffness, low density, good corrosion resistance, good biocompatibility. However, Ti alloys often suffer from poor wear resistance owing to their relatively low hardness [1-3]. Such a shortcoming prevents the further application of Ti alloys in many fields. Generally, the failure of industrial materials starts from their surfaces, especially under wear and corrosion environments. Therefore, it is essential to improve the wear resistance of Ti alloys using surface modification techniques with the aim of improving their global performance. Different surface treatment techniques, such as physical vapor deposition (PVD), chemical vapor deposition (CVD), sol-gel methods, anodic oxidation, have been attempted to improve the wear or corrosion resistance of Ti and Ti alloys [4,5]. However, these methods have their own limitations. For instance, the films deposited onto Ti alloys using PVD or CVD methods are often very thin and suffer from poor adhesion with the substrate.
Among different kinds of surface treatment techniques, laser surface cladding offers an optimum approach to obtain a thick layer onto a material with superior properties. Using laser cladding, different alloys or metal matrix composites can be easily produced onto treated material surfaces with strong metallurgical bonding between the clad layer and the substrate [3,6]. Laser cladding has been applied to Ti alloys with the aim of improving the wear and corrosion resistances. For this purpose, different metal matrix composites, such as Ti/TiC, Ti/TiB2, have been produced either in-situ or ex-situ on Ti alloys by using laser cladding technique [3,7]. It has been established that, minor addition of rare earth metal oxides, such as La2O3, Y2O3 and CeO2 into laser clad layers increases the hardness and homogeneity of the clad layers. As a result, the wear resistance of the laser clad layer can be significantly improved [8-10]. Considering that Y2O3 is an efficient additive to improve the property of laser clad TiC layer [8], the present work attempts to in-situ fabricate a metal matrix composite, Y2O3 particle enhanced Ni/TiC, onto a TC4 Ti alloy. The aim of this work is to produce a hardened surface layer on the Ti alloy with higher hardness and better wear resistance. The microstructure, composition and property modifications encountered in the laser clad layer are investigated in details.
2 Experimental
2.1 Sample preparation and laser treatment
The titanium alloy investigated in this work was the commercially available TC4 alloy, which was solution treated at 1100 °C and subsequently quenched in water. Its nominal chemical composition (mass fraction) is as follows: 6.5% Al, 4.26% V, and Ti balance. Samples for laser treatment were cut into small coupons (15 mm× 15 mm×10 mm) from a 10 mm-thick sheet. All the samples were ground with sand papers, polished down to diamond paste of 1.5 μm and ultrasonically cleaned in acetone prior to the laser treatments. The microstructure aspect of the initial material was given in Ref. [7], which contains a single fine lamellar (~2 μm in width) martensitic structure within very large parent β domains.
Prior to the laser treatment, mixed TiC, Ni and Y2O3 powders with a mass ratio of 1:3:0.08 were put onto the TC4 sample. The powder layer was then compressed in order to remove the gas between the powder particles. The total depth of the powder layer was around 500 μm. The laser cladding treatment was carried out using an HL-2000 type CO2 laser source. The beam cross section (shape and area), beam power and scanning speed were the main parameters to be adjusted. In the present study, the laser beam parameters were set as follows: beam diameter 5 mm, beam power 3500 W, scanning speed 5 mm/s.
2.2 Characterization of laser clad layer
The phases present on the surface of treated sample were analyzed by X-ray diffraction (XRD) using a Shimadzu D-6000 goniometer operating with Cu Kα radiation source. The microstructure of the treated samples was observed from cross section by optical microscopy (OM) and scanning electron microscopy (SEM). JEOL 6500F field emission gun SEM apparatus equipped with an electron dispersion X-ray spectroscopy (EDX) was used.
Modification of the composition within the laser clad layers was investigated by EDX analysis at different depth. The cross-section Vickers hardness of the samples was measured with 0.98 N load. The wear resistance of the initial and laser cladded samples were measured using a HT-600 multifunctional friction and wear system. The friction counterpart was a ceramic ball with diameter of 5 mm. The load was 10.29 N. The sliding speed was set at 5 mm/s and the total sliding time was 120 min.
3 Results
3.1 Cross-sectional microstructure and phase
Figure 1 gives the OM image illustrating the overall morphology of the laser clad layer from a cross-sectional view. The depth of the laser clad layer is around 1 mm. No cracks can be observed in the clad layer and at the melt/substrate interface. Unlike the laser clad Ti/TiC composite coating [7], the laser clad Ni/TiC composite layer has a quite flat surface. It has been established that the shape of laser clad layer depends mainly on the wettability of the laser clad layer with regard to the substrate [11,12]. Better wettability gives a depressed shape of the surface. Clearly, the Ti/TiC composite coating has better wettability with Ti substrate than Ni/TiC coating as Ti/TiC coating contains Ti. Within this laser clad layer, very fine dendritic structure can be clearly seen, which corresponds to the TiC phase [13].
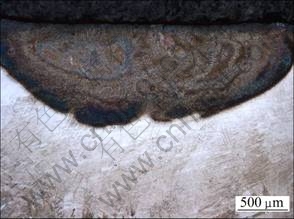
Fig. 1 Typical OM image showing cross-section morphology of laser clad layer on TC4 sample
Figure 2 shows the XRD pattern of the laser treated sample. The peaks corresponding to TiC, Ti and NiTi phases appear after the laser treatments. This means that a reaction between Ni and Ti forms NiTi phase during the laser cladding process. No peaks corresponding to Y2O3 are observed in the XRD pattern due to its low concentration in the clad layer.
Figure 3 shows the typical cross-sectional SEM images of the laser cladded TC4 sample at the top surface, in the middle, and at the bottom of the melted layer, respectively. These SEM images give more details of the microstructure present in the melted layer. It can be seen from Fig. 3(a) that, fine TiC dendrites are distributed homogeneously in the clad layer. Beside, there are also some fine particles/grains distributing between those TiC dendrites, as indicated by arrows in Fig. 3(a). These particles show different contrast with regard to the TiC dendrites, indicating that they are different phases. Similar morphology is also observed in the middle of the clad layer, as shown in Fig. 3(b). In Fig. 3(c), it is seen that the density of TiC dendrites decreases gradually when approaching the melt/substrate interface, showing a good metallurgical bonding between the clad layer and the substrate. Comparing Figs. 3(a), (b) and (c), it is clear that the size of the TiC dendrites deceases with the depth increasing.
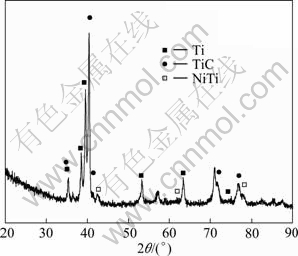
Fig. 2 XRD pattern of laser clad sample
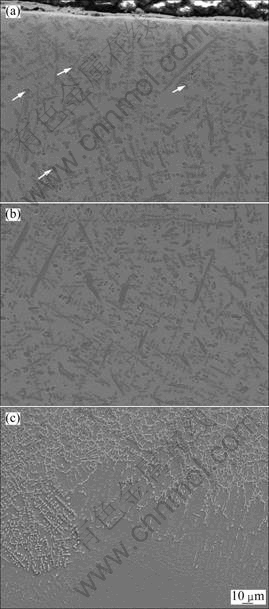
Fig. 3 Cross-sectional SEM images of laser cladded TC4 sample showing microstructures at the top surface (a), in the middle (b), and at the bottom (c) of melted layer
3.2 Composition distribution in laser clad layer
Figure 4(a) shows a SEM image taken at the top surface of the clad layer, and the corresponding element distribution maps of C, Ti, Al, V, Ni and Y are shown in Figs. 4(b-g). In Fig. 4(a), one can clearly see the dark dendrites and some grains with irregular shape. From Figs. 4(b-g), the distributions of different elements in the top surface layer are quite heterogeneous. From Fig. 4, several features can be observed: 1) the dendrites are rich in Ti and C and thus confirmed to be TiC; 2) those grains with irregular shape are rich in V and Ti; 3) the interdendritic areas are rich in Al and Ni; and 4) Y exists mainly within very fine particles having diameter in the range of several hundred nanometers to 2 μm. Figure 5 shows a similar set of images taken at the melt/substrate interface as Fig. 4. In Fig. 5(a), the fine TiC dendrites are present and no grains with irregular shape are observed. It can be found from Figs. 5(b-g) that, the distributions of different elements at the melt/substrate interface are more homogeneous than those in the top surface layer. There is less Ni at the bottom of the melt and Y still present as very fine particles.
3.3 Cross-sectional microhardness and wear resistance of laser clad layer
Figure 6 shows the microhardness profile along depth measured for the laser treated sample. The initial microhardness of the TC4 alloy is about HV 300, as seen on the profile when the depth is beyond 1000 μm. From this profile, it is clear that a significant hardening is obtained by laser cladding. The maximum hardness in the clad layer reaches about HV 1380, which is more than 4 times of the initial one. The hardness is fairly uniform in the clad layer, and decreases rapidly after approaching the substrate.
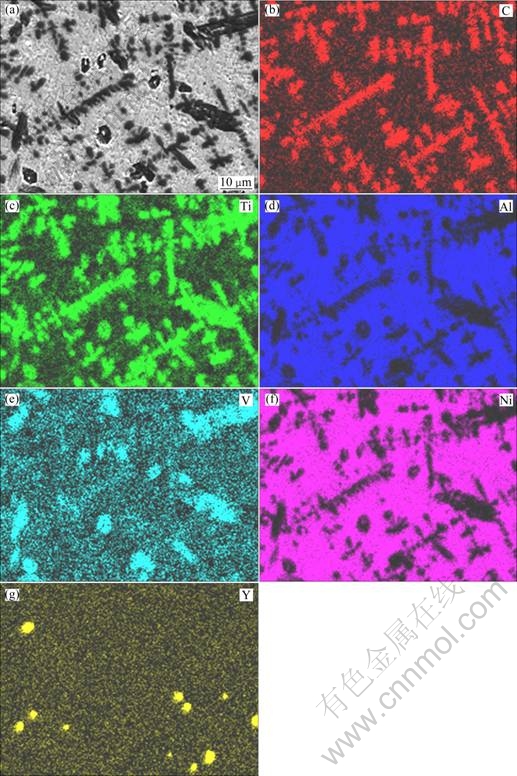
Fig. 4 SEM image taken at top surface of clad layer (a) and corresponding composition distribution maps (b-g) by EDX
Figure 7 shows the friction coefficient versus time for the initial sample and the laser cladded sample, respectively. The friction coefficient of the initial sample is in the range between 0.2 to 0.5 and shows a large fluctuation with sliding time. This indicates the unstable wear behaviour of the initial sample. For the laser cladded sample, the friction coefficient keeps almost constant at 0.5 during the whole measurement time, showing the stable wear behaviour. After 120 min of sliding wear test, the mass loss of the initial sample is 0.0249 g while it is 0.0207 g for the laser cladded sample. The above results confirm that the friction and wear resistance of the TC4 Ti alloy can be significantly improved by laser cladding with Y2O3 particle enhanced Ni/TiC composite coating.
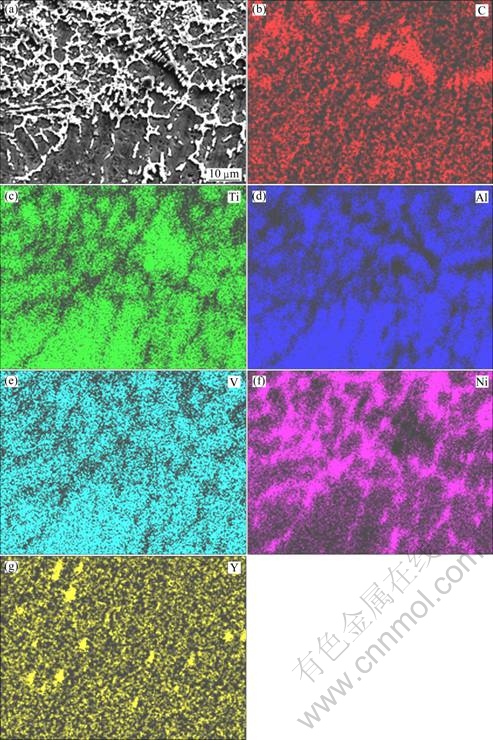
Fig. 5 SEM image taken at bottom of clad layer (a) and corresponding composition distribution maps (b-g) by EDX
4 Discussion
Laser cladding induces many phenomena, such as fast heating, melting, liquid mixing, convection, rapid solidification and cooling in the surface layers of the treated materials [11,14]. The final microstructure and properties of the laser clad layer are determined by the combination of the above phenomena. However, the thermal history at different depth is very different, leading to the formation of a microstructure and composition graded layer after laser treatment.
In the present work, the thick Ni+TiC+Y2O3 powder layer pre-coated on the TC4 alloy substrate is melted during laser cladding. At the early stage of laser cladding, Ni particles at the top surface layer are melted prior to the TiC and Y2O3 particles due to its lower melting point [15]. After absorbing enough energy from the laser beam, TiC particles start to melt and dissolve into Ni melt, so do Y2O3 particles [16]. When the whole powder layer is melted, a part of the matrix will also be included into the laser clad layer through convection effect [17]. A composition gradient is established at this stage, especially at the bottom of the melt close to the matrix. C and Ni will diffuse toward the bottom of the melted layer while Ti, Al and V will move from the melted matrix to the top surface layer. However, the melt dwell time is not enough so that the composition gradient still exists before solidification. During the cooling stage, the solidification initiates from the melt/substrate interface due to the heat conduction toward matrix [15]. At the early stage of the solidification, TiC phase forms first due to its high solidification temperature [17]. It grows larger and larger until the local temperature decreases down to a certain value where the Ni, NiTi or TiV solid phases appear [18]. The melt undergoes such a process until all the liquid is consumed. As the cooling rate at the solidification front decreases when it moves toward the surface [15], the melt duration increases from melt bottom to the top. Consequently, TiC dendrites are larger in the top surface layer than those in the bottom of the melt. On the other hand, since the solubility of Al in TiC is very limited, the Al atoms will be pushed out from TiC dendrites and remain in the rest of liquid. Therefore, the interdendritic areas will be rich in Al, depending on the local cooling rate [19]. Considering the above factors, a laser clad layer having graded microstructure and composition forms in the end.
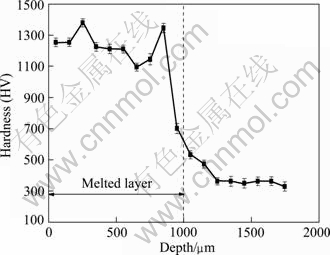
Fig. 6 Cross-sectional microhardness profile of laser cladded TC4 sample
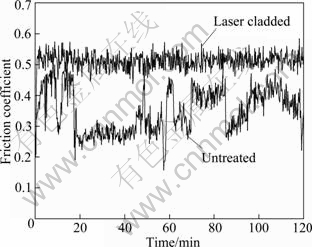
Fig. 7 Friction coefficient vs time for untreated and laser cladded TC4 samples
The high hardness of the laser clad layer is mainly due to the presence of TiC dendrites [13,16]. The fine Y2O3 particles may also increase the hardness of the Ni/TiC composite owing to the dispersion hardening effect. It has been observed that minor addition of Y2O3 particles into laser clad TiC layer leads to the homogenization of the microstructure and thus reduces the cracking tendency of the clad layer. Consequently, the laser clad TiC layer containing Y2O3 particles shows better properties than the TiC layer without Y2O3 particles [8]. However, the experimental results also revealed that excessive addition of rare earth oxide particles would deteriorate the properties of the laser clad layer [8-10]. In the laser clad layer, the volume fraction of TiC dendrites is quite homogeneous throughout the thickness, although the size becomes finer when approaching substrate. As a result, the hardness distribution is quite uniform along depth in the laser clad layer. The higher hardness value of the laser clad layer over substrate accounts for improved friction and wear resistances of the sample after laser cladding.
5 Conclusions
1) A Y2O3 particle enhanced Ni/TiC composite coating was successfully fabricated onto the TC4 substrate by laser cladding. It was observed that a layer having graded microstructures and compositions formed on the matrix due to the melting, liquid state convection followed by rapid solidification and cooling.
2) TiC powders were completely dissolved into the melted layer during melting and segregated as fine dendrites when solidified. The inter-dendritic areas were filled with NiTi rich in Al. Y2O3 was present as dispersed fine particles with diameter in the range of several hundred nanometers to 2 μm.
3) The size of the TiC dendrites decreased with increasing depth, while the distribution of elements became more homogeneous. Some Ti- and V-rich grains were observed at the top surface layer but were absent at the melt/substrate interface.
4) The laser clad layer showed a quite homogeneous hardness profile along depth with a maximum value of HV 1380. Due to the high hardness of the laser clad layer, the friction coefficient of the laser cladded sample became more stable when compared to that of the initial sample. The wear resistance of the TC4 alloy was significantly improved after laser cladding with Y2O3 particle enhanced Ni/TiC composite coating.
References
[1] LIU Dao-xin, TANG Bin, ZHU Xiao-dong, CHEN Hua, HE Jia-wen, CELIS J P. Improvement of the fretting fatigue and fretting wear of Ti6Al4V by duplex surface modification [J]. Surface and Coatings Technology, 1999, 116-119: 234-238.
[2] HAGER C H J, SANDERS J H, SHARMA S. Unlubricated gross slip fretting wear of metallic plasma-sprayed coatings for Ti6Al4V surfaces [J]. Wear, 2008, 265: 439-451.
[3] PANG W, MAN H C, YUE T M. Laser surface coating of Mo–WC metal matrix composite on Ti6Al4V alloy [J]. Materials Science and Engineering A, 2005, 390: 144-153.
[4] VARIOLA F, YI JH, RICHERT L, JWUEST JD, ROSEI F, NANCI A. Tailoring the surface properties of Ti6Al4V by controlled chemical oxidation [J]. Biomaterials, 2008, 29: 1285-1298.
[5] SILVA M M, UEDA M, PICHON L, REUTHER H, LEPIENSKI C M. Surface modification of Ti6Al4V alloy by PIII at high temperatures: Effects of plasma potential [J]. Nuclear Instruments and Methods in Physics Research B, 2007, 257: 722-726.
[6] ALEMOHAMMAD H, ESMAEILI S, TOYSERKANI E. Deposition of Co-Ti alloy on mild steel substrate using laser cladding [J]. Materials Science and Engineering A, 2007, 456: 156-161.
[7] ZHANG Ke-min, ZOU Jian-xin, LI Jun, YU Zhi-shui, WANG Hui-ping. Surface modification of TC4 Ti alloy by laser cladding with Ti+TiC powders [J]. Transactions of Nonferrous Metals Society of China, 2010, 20(11): 2192-2197.
[8] WANG Hui-ping, LI Jun, ZHANG Guang-jun, DAI Jian-qiang, XI Wen-long.Effect of Y2O3 on microstructure and wear resistance of laser cladding TiC coating on the surface of TC4 titanium alloy [J].Applied Laser,2010,30:199-203.
[9] CHENG Xi-yun, HE Ke-shan, HE Jun. Influence of CeO2 on tribological properties and microstructure of laser cladding TiC4 ceramic layer [J]. Tribology, 2010, 30: 250-255.
[10] LI An-min, XU Bo-fan, PAN Ying-jun. Effect of La2O3 on microstructure and property of TiC/Ni-based composite coating [J]. Journal of Iron and Steel Research, 2003, 5: 57-61.
[11] CAI L F, ZHANG Y Z, SHI L K. Microstructure and formation mechanism of titanium matrix composites coating on Ti-6Al-4V by laser cladding [J]. Rare Metals,2007, 26: 342-346.
[12] MAJUMDAR J D, MANNA I, KUMAR A. Direct laser cladding of Co on Ti-6Al-4V with a compositionally graded interface [J]. Journal of Materials Processing Technology, 2009, 209: 2237-2243.
[13] PU Y P, GUO B G, ZHOU J S. Microstructure and tribological properties of in situ synthesized TiC, TiN, and SiC reinforced Ti3Al intermetallic matrix composite coatings on pure Ti by laser cladding [J]. Applied Surface Science, 2008, 255: 2697-2703.
[14] LI Li-qun, LIU De-jian, CHEN Yan-bin, WANG Chun-ming, LI Fu-quan. Electron microscopy study of reaction layers between single-crystal WC particle and Ti–6Al–4V after laser melt injection [J]. Acta Materialia, 2009, 57: 3606-3614.
[15] YANG Y L, ZHANG D, LIU C S, YANG Y R. Laser cladding of TiCN/Ti coatings on Ti6-Al4-V and the calculation of the temperature field [J]. Optical Engineering, 2008, 47: 084301-5.
[16] YANG Yu-ling, ZHANG Duo, YAN Wei, ZHENG Yi-ran. Microstructure and wear properties of TiCN/Ti coatings on titanium alloy by laser cladding [J]. Optics and Lasers in Engineering, 2010, 48: 119-124.
[17] BRIAN C L, SHAHRZAD E. In-situ laser-fabrication and characterization of TiC-containing Ti–Co composite on pure Ti substrate [J]. Journal of Alloys and Compounds, 2009, 482: 246-252.
[18] SUN R L, LEI Y W, NIU W. Laser clad TiC reinforced NiCrBSi composite coatings on Ti–6Al–4V alloy using a CW CO2 laser [J]. Surface and Coatings Technology, 2009, 203: 1395-1399.
[19] BOETTINGER W J, CORIELL S R, GREER A L, KARMA A, KURZ W, RAPPAZ M, TRIVEDI R. Solidification microstructures: Recent developments, future directions [J]. Acta Materialia, 2000, 48: 43-70.
TC4钛合金表面激光熔覆法制备
Y2O3颗粒增强Ni/TiC复合涂层
张可敏1,邹建新2,李 军1,于治水1,王慧萍1
1. 上海工程技术大学 材料工程学院,上海 201620;
2. 上海交通大学 材料科学与工程学院,上海市镁材料及应用工程技术研究中心,上海 200240
摘 要:采用激光熔覆法在TC4钛合金表面原位制备Y2O3颗粒增强Ni/TiC复合涂层,研究涂层的相组成、微结构、成分分布及性能。结果表明,复合涂层内的微结构和成分在深度方向具有分层现象,这主要是由激光熔覆过程的快速熔凝和冷却过程所致。在激光熔覆过程中,TiC粉末完全熔化并在凝固过程中析出为细小枝晶,这些TiC枝晶的尺寸随着深度的增加而减小,而Y2O3颗粒则分布在整个重熔层中。Y2O3颗粒增强Ni/TiC复合涂层具有较均匀的硬度,其最高值约为HV1380,比基体高4倍以上。由于复合涂层具有高的硬度,钛合金经过激光熔覆后其耐磨性得到大幅度提高。
关键词:TC4钛合金;Ni/TiC复合涂层;Y2O3;激光熔覆;硬度;表面改性
(Edited by YUAN Sai-qian)
Foundation item: Projects (51101096, 51002093) supported by the National Natural Science Foundation of China; Project (1052nm05000) supported by Special Foundation of the Shanghai Science and Technology Commission for Nano-Materials Research; Project (J51042) supported by Leading Academic Discipline Project of the Shanghai Education Commission, China
Corresponding author: ZHANG Ke-min; Tel: +86-21-67791203; E-mail: zhangkm@sues.edu.cn; cocozkm@gmail.com
DOI: 10.1016/S1003-6326(11)61392-7