
J. Cent. South Univ. (2019) 26: 796-805
DOI: https://doi.org/10.1007/s11771-019-4049-z

Effect of iron transformation on Acidithiobacillus ferrooxidans bio-leaching of clay vanadium residue
FENG Ya-li(冯雅丽)1, WANG Hong-jun(王洪君)1, LI Hao-ran(李浩然)2,
CHEN Xi-pei(陈熹沛)1, DU Zhu-wei(杜竹玮)2, KANG Jin-xing(康金星)1
1. School of Civil and Resource Engineering, University of Science and Technology Beijing,Beijing 100083, China;
2. Key State Laboratory of Biochemical Engineering, Institute of Process Engineering,Chinese Academy of Sciences, Beijing 100190, China
Central South University Press and Springer-Verlag GmbH Germany, part of Springer Nature 2019
Abstract: The acid bio-leaching process of vanadium extraction from clay vanadium water-leached residue was studied and the effect of the performance of iron transformation was investigated. Acidithiobacillus ferrooxidans affects the dissolution of vanadium through the catalytic effect on Fe3+/Fe2+ couple and material exchange. The passivation of iron settling correlates with ferrous ion content in bio-leaching solution. In medium containing A. ferrooxidans and Fe(III), the increment in Fe(II) concentration leads to the formation of jarosite, generating a decline in vanadium extraction efficiency. Analysis of cyclic voltammetry shows that Fe(II) ion is apt to be oxidized and translated into precipitate by A. ferrooxidans, which strongly adsorbed to the surface of the residue. Fe(III) ion promotes the vanadium extraction due to its oxidizing activity. Admixing A. ferrooxidans to Fe(III) medium elevates the reduction of low valence state vanadium and facilitates the exchange of substance between minerals and solution. This motivates 3.8% and 21.8% increments in recovery ratio and leaching rate of vanadium compared to the Fe(III) exclusive use, respectively. Moreover, Fe(II) ion impacts vanadium extraction slightly in sterile medium but negatively influences vanadium leaching in the presence of bacteria.
Key words: iron ion cycle; Acidithiobacillus ferrooxidans; passivation; cyclic valtammetry; clay vanadium
Cite this article as: FENG Ya-li, WANG Hong-jun, LI Hao-ran, CHEN Xi-pei, DU Zhu-wei, KANG Jin-xing. Effect of iron transformation on Acidithiobacillus ferrooxidans bio-leaching of clay vanadium residue [J]. Journal of Central South University, 2019, 26(4): 796–805. DOI: https://doi.org/10.1007/s11771-019-4049-z.
1 Introduction
Vanadium is one of the most common alloy elements used in ferrous and non-ferrous alloy because it possesses unique properties of high tensile strength, hardness, and fatigue resistance [1]. Clay vanadium mineral, like vanadium-titanium magnetite and vanadium-bearing stone coal, is an important resource for vanadium [2]. The extraction of vanadium from clay ore usually adopts roasting-water leaching-precipitation separation [3, 4]. However, multiple factors, involving the partial oxidation of vanadium minerals and the insufficient water-leaching efficiency, contribute to low recovery ratio of vanadium and massive loss of metal. Hence, a practical process needs to be developed for the utilization of clay vanadium residues.
In the development of low-grade ores, several reasons emerge for the biohydrometallurgy process preference including environmental and economic benefits [5–7]. Acidithiobacillus ferrooxidans (A. ferrooxidans) is a kind of microorganisms most widely used in bio-leaching on account of its catalysis oxidation for the dissolution of valuable metals [8, 9]. Studies have been conducted in the applications of A. ferrooxidans in vanadium ore. HUANG et al [10] and LIAO et al [11] reported the biological process of vanadium-bearing stone coal using A. ferrooxidans. According to the literature, A. ferrooxidans could lower the toxicity of waste catalyst and extract 94.8% vanadium [12]. However, since the oxidation state of vanadium in the raw material was often unknown and the effect of the transformation of iron ion was not determined in most reports, the behavior of vanadium material A. ferrooxidans leaching was not studied sophisticatedly.
This study investigates the bio-extraction of vanadium from the vanadium residue of sodium roasting-water leaching clay ore using A. ferrooxidans. The effects of A. ferrooxidans, Fe(III), and Fe(II) on vanadium leaching in sulfuric acid solution were studied using A. ferrooxidans, Fe(III), or Fe(II) alone and their combined solutions. The influence of iron ion transformation on vanadium biological course was estimated. The purpose of this study was to provide a theoretical reference for clean and efficient bioprocess of vanadium material.
2 Experimental procedure
2.1 Materials and bacterial culture
The raw clay vanadium residues were obtained from Hubei Province, China, which had been processed by sodium roasting and water immersion in smelting plant. The sample was air-dried, crushed and ground to 74 μm in the laboratory before use. The chemical compositions of the vanadium residue and raw clay ore were listed in Table 1, and the oxidation states of vanadium of them were shown in Table 2. When the original clay ore has handled with Na-activation-and-water logging, most of the vanadium recycled. The raw residual vanadium emerged three kinds of valence which showed that vanadium existed in insoluble compounds, unoxidized navajoite, and silicate minerals.Figure 1 shows the X-ray diffraction (XRD) pattern of water-leaching residue. The main minerals in the residue include quartz, anorthite, and albite [13]. The scanning electron microscope (SEM, JSM- 7001F, JEOL) image and energy dispersive spectrometer (EDS, Inca X-Max, Oxford Instruments) analysis (Figure 2) illustrate that vanadium is mainly presented in the aluminosilicate.
Table 1 Chemical composition of raw clay vanadium ore and its water-leaching residue (mass fraction, %)
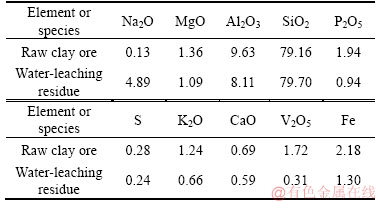
Table 2 Distribution ratio of valence state of vanadium in raw clay ore and its water-leaching residue (mass fraction, %)
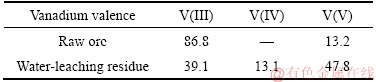
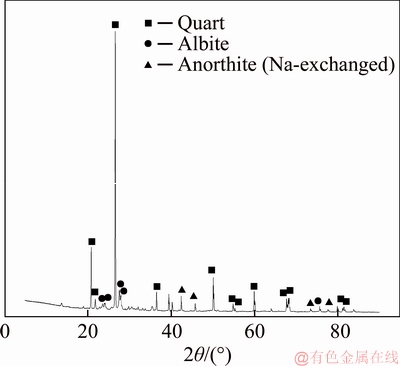
Figure 1 XRD pattern of water-leaching vanadium residue
A. ferrooxidans was obtained from the Environmental Biological Science and Technology Research Center, Institute of Geochemistry, Chinese Academy of Science. The abundance of A. ferrooxidans species was determined by sampling microorganism cultivated in iron-free 9K medium ((g/L)): (NH4)2SO4 0.15, KCl 0.05, K2HPO4 0.05, MgSO4·7H2O 0.05, Ca(NO3)2 0.01. The multiplication culture medium added 9 g/L FeSO4·7H2O to the 9K basic medium. The bath solutions involved a blank iron-free 9K medium, an admixture of Fe(III), Fe(II) or A. ferrooxidans and 9K basic medium, and a mixed solution of 9K basic medium, bacteria, and iron salts. The blank group culture was a germfree and ironless 9K basic medium. A selected mass of Fe2(SO4)3 and FeSO4·7H2O dissolved in the blank culture constituted to the Fe(III) and Fe(II) medium, respectively. The A. ferrooxidans medium was a culture which suspended bacteria in 9K basic medium. The complex solutions of A. ferrooxidans and iron ion were obtained by inoculating bacteria to the iron-containing 9K basic culture. The cells used in this work were isolated from the late logarithmic growth phase by centrifuging at 12000 r/min for 20 min. A solution of 20% H2SO4 was used to adjust the pH of the liquid media.
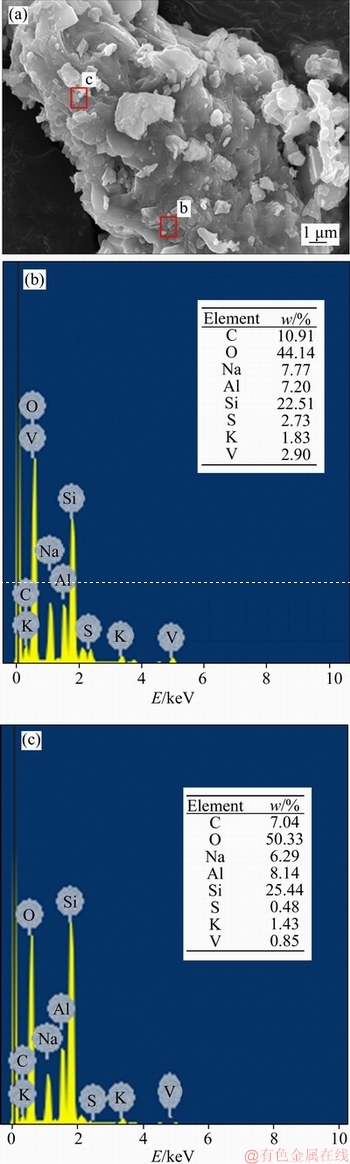
Figure 2 SEM and EDS diagram of water-leaching vanadium residue
2.2 Procedures
2.2.1 Leaching experiments
A selected water-leaching vanadium residue (5 g) and bath medium (100 mL) were mixed in a shake flask (250 mL) for leaching tests. Vibration leaching experiments were conducted in a temperature shaking incubator at the temperature of 30 °C and vibration speed of 140 r/min. Three groups were tested in parallel. After an established time, a quantity of infusion solution was taken out for analysis of the elemental content and potentiometric. End of the leaching, the slurry was filtered and the filter residue was washed and dried.
The leaching efficiency of vanadium was calculated based on the amount of leached metal in the infusion solution with respect to the original input quantity. Vanadium and iron in the lixivium were estimated by titrimetry of ammonium ferrous sulfate and phenanthroline spectrophotometric method, respectively. The difference between inorganic and biological leaching residues was characterized by Fourier transform infrared spectroscopy (FIRT, ALPHA, BRUKER). The pH value was measured by a pH meter (pHS-25).
2.2.2 Thermodynamic analysis
According to the analysis of the chemical composition of the raw vanadium residue, V, Fe and S elements were studied using calculations based on 1 and 0.01 unit activities at 25 °C and supposing that all of the species were at the ideal state. The Eh–pH diagrams of V-S-H2O and Fe-K2SO4-H2O were calculated and plotted by the software HSC Chemistry 6.0.
2.2.3 Electrochemical experiments
The electrochemical tests were performed on the electrochemical workstation (CH1660D, CH Instruments) which adopted a three electrodes system with Pt plate (1.0 cm2) as a counter electrode, saturated calomel electrode (SCE) as a reference electrode, and the glassy carbon electrode(GEC) which was plated residues as working electrodes (0.1 cm2). The plated working electrodes were set up by depositing a thin-layer of the original vanadium residue ink over a GEC. The residue ink was prepared by blending the vanadium residue (90 wt%) and polytetrofluoroethylene (10 wt%) in isopropyl alcohol. The electrodes were dried at 60 °C for 12 h. After that three electrodes were immersed in the inorganic or organic sulfuric acid leaching medium for 20 min, and the scan was cativatied. Electrochemical measurements were implemented at 30 °C by connecting a circulating thermostatically controlled water loop (refer to SCE for all potential shown in this work).
3 Results and discussion
3.1 Dependence of vanadium extraction on ferric ion concentration, ferrous ion concentration, and inoculation of A. ferrooxidans
Figure 3 shows dependence of vanadium extraction degree on the Fe(III) concentration, Fe(II) concentration and bacteria number density. Fe(III) ion has a remarkable effect on the leaching efficiency and leaching rate of vanadium (Figure 3(a)). About 27% vanadium was leached in vitriol solution after 30 h. The addition of Fe(III), even 0.2 g/L, led to increasing in vanadium extraction ratio by 11%. ZHU [14] reported that diluted acid could merely expend vanadium from water-insoluble vanadate. It is likely that Fe(III) ion participates in the redox of vanadium-containing minerals and oxidizes insoluble vanadium material to soluble substance. Higher Fe(III) concentration strengthens the extraction rate of vanadium. With the presence of 1.0 g/L Fe(III), average dissolution rate of vanadium reaches a maximum within 12 h. However, the leaching rate of vanadium did not increase with the concentration of Fe(III) increased from 1.0 to 1.5 g/L, and the vanadium recovery decreased 0.8%. So, it can be claimed that admixing exorbitant Fe(III) to the solution is harmful to vanadium leach. Addition of Fe(II) to the acid solution slightly affects vanadium leaching, suggesting that a small quantity of vanadium mineral can be corroded by Fe(II) ion (Figure 3(b)). The initial amount of A. ferrooxidans has a minor effect on vanadium leaching as shown in Figure 3(c). Clearly, without iron ion, the vanadium leach is not responding to A. ferrooxidans directly.
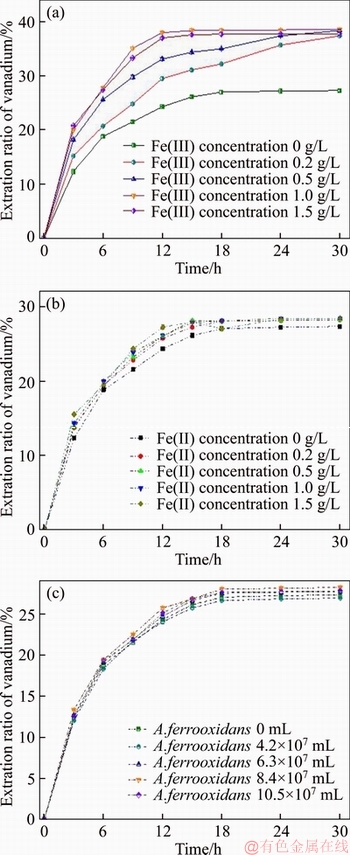
Figure 3 Effects of Fe(III) concentration (a), Fe(II) concentration (b), and inoculation amount of A. ferrooxidans (c) on vanadium acid leaching
3.2 Effect of Fe(III) concentration on vanadium A. ferrooxidans leaching
The combined effects of Fe(III) and A. ferroxidans on the leaching process were shown in Figure 4. Fe(III) ion and A. ferrooxidans impact vanadium bio-leaching remarkably. When adding A. ferrooxidans to the 1.0 g/L Fe(III)-containing medium, leaching rate increases with increasing the number of bacteria. This consequence, combined with the results obtained from Figure 3, leads to the vanadium A. ferrooxidans bio-leaching supports the indirect effect mechanism of A. ferrooxidans.Studies have reported that A. ferrooxidans increases the rate of electron transmission resulting in promotion of oxidation-reduction reaction [15, 16]. However, admixing Fe(III) ion and A. ferrooxidans to the bath solution, the extraction fraction of vanadium increased first and then decreased. This decrement may be explained by sedimentation of the dissolved vanadium. When the concentration of Fe(III) is higher than 0.5 g/L in the microbial process, the vanadium extraction efficiency reduced within 18 h. This elucidates that ferric concentration, beyond a certain level, is against vanadium bio-leaching in the later stage.
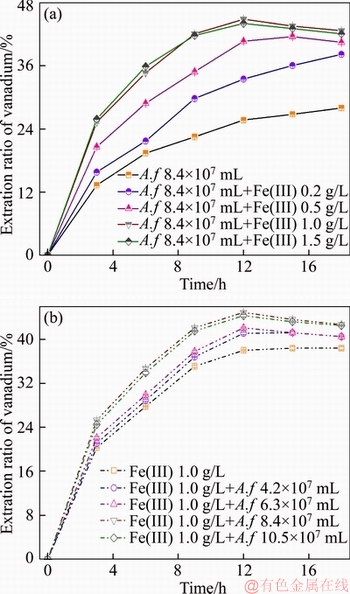
Figure 4 Effect of Fe(III) on vanadium A. ferrooxidans (A.f) bio-leaching
Figure 5 presents the change of ferric ion and pH during the leaching. With the decrement in Fe(III), vanadium leached into solution. The drop of total iron should originate in the absorption of the ore. The Fe(II) content of solution increased with the reaction degree in the sterile solution, indicating that Fe(III) has been restored to Fe(II) by low valence vanadium. However, with the usage of A. ferrooxidans, the content of iron ion in the solution lowered 30 mg/L, the Fe(II) concentration increased to 13.6 mg/L then decreased, and the decline rate of Fe(III) concentration increased after 9 h leaching, suggesting that in A. ferrooxidans-containing solution the later decrement in vanadium extraction is caused by the sedimentation of iron compounds. pH of the solution increased over time, indicating that hydrogen ions enter into the dissolution of the water-leaching vanadium residue. A. ferrooxidans promotes the formation of this dreg rather than operation with Fe(III) solely in the experimental pH range (1.8 to 2.06) in line with the results in Figure 3. Thereby, it is possible that an organic film, of which the amount of iron species has been estimated to be approximately 53 g/L, formed in the bacterium–substratum interaction [17]. Moreover, the ratio of Fe(III)/Fe(II) in the solution determines that of in the layer [18, 19]. The increase in the proportion of Fe(II) causes settlement phenomenon in bio-leaching process assigned to that A. ferrooxidans oxidizes Fe(II) to Fe(III) on the membrane surface inducing the generation of precipitate [20]. So, it is reasonable to postulate, based on those results, that it can regulate the formation of sediment by controlling ratio of Fe(III)/Fe(II) and pH of the bio-leaching solution.
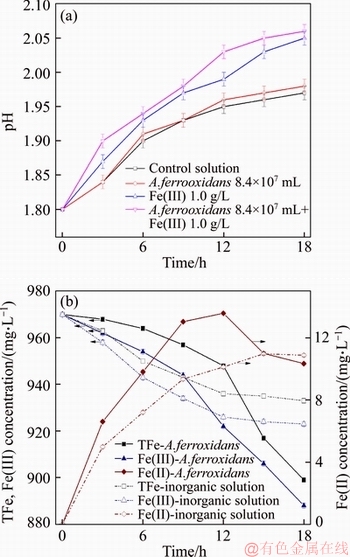
Figure 5 Variations of pH (a), total Fe, Fe(III), and Fe(II) (b) concentration versus time
3.3 Effects of Fe(III) to Fe(II) ratio and pH on vanadium A. ferrooxidans leaching
The raw residue leached at constant pH and ferric to ferrous ratio (Figure 6). Figure 6(a) shows that controlling sulfuric acid concentration influences the vanadium leaching significantly. To maintaining pH at 1.8, the extraction ratio obtained 48.4% in organic solution. However, high or low pH is unfavourable to vanadium leaching due to the performance of biological activity. The results in Figure 6(b) show employing Fe(II) in the Fe(III) and A. ferrooxidans mixture solution lowered vanadium leaching rate and extraction ratio. Introducing only 10 mg/L Fe(II) to the Fe(III) and A. ferrooxidans containing solution, the vanadium recovery reduced over 11%. This means that as long as there is ferrous high-enough concentration in the vanadium bio-leaching can be precipitated iron compounds which evoke a decrease in leaching efficiency. It is likely that in vanadium bio-leaching the reaction of iron deposition depends much on the ratio of Fe(III)/Fe(II) at pH 1.8.
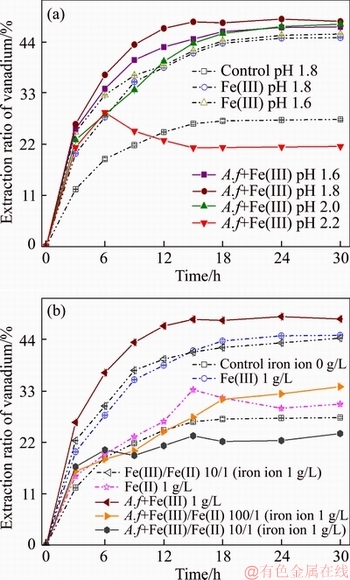
Figure 6 Effects of (a) pH and (b) ferric to ferrous ratio on vanadium A. ferrooxidans (A.f) bio-leaching
The results summarized in Figure 7 demonstrate that with 1.0 g/L Fe(III) germs facilitate the conversion of V(III), V(IV), and V(V) minerals, improving vanadium leaching recovery and speed by 3.8% and 21.8%, respectively. Part of V(III) and V(V) minerals dissolved in dilute sulfuric acid. Fe(III) oxidized the V(III) minerals, and it appears to directly affect the reduce of V(V)- containing substances, increasing the V(IV) ores.
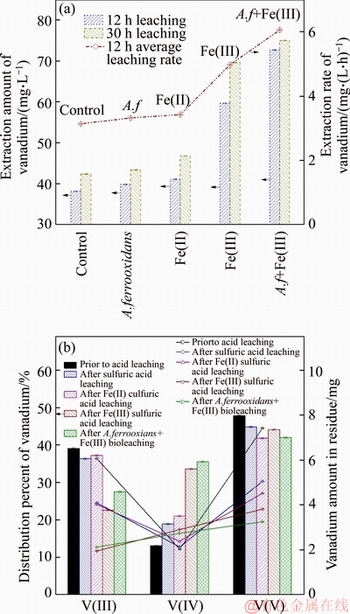
Figure 7 Effect of leaching solution on vanadium leaching efficiency and rate (a), content of vanadium valence states (b)
On the other hand, using Fe(II) gives a rise to the V(IV) matters. Thereby, it can be concluded that iron ion enhances the dissolution of vanadium materials. With the presence of A. ferrooxidans, there is a decline in the remaining quantity of tetravalent vanadium compound. This should be because the promotion of A. ferrooxidans on electron transfer reduces the production of the V(IV) settings.
3.4 A. ferrooxidans vanadium leaching process
3.4.1 Infrared analysis
Figure 8 represents the FTIR spectra of Fe(III)-containing acid leaching and bio-leaching residues. Infrared ray spectrum recovered that the absorption peak of ihleite formed in bio-leaching route. An infrared absorption between 3390 and 3410 cm–1 of bio-leaching residue is observed, which is identified as —O—H. Characteristic peak of FeO6 that is a positive octahedral structure appeared at 474 cm–1. The stretching vibration peak of 629 cm–1 is considered to SO42–. Therefore, it can be claimed that A. ferrooxidans promotes the formation of ihleite precipitate that influences vanadium leaching. In addition, microorganism impacts the disintegration of roscoelite according to the weakening of Si—O—Si symmetrical stretching vibration peak of 690 cm–1.
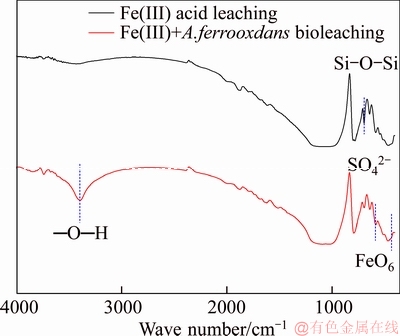
Figure 8 Infrared spectroscopy of Fe(III) acid leaching and bio-leaching
3.4.2 Thermodynamic analysis
In order to predict the thermodynamic feasibility and decomposition behavior of both navajoite and utahite, the systems both V-S-H2O and Fe-K2SO4-H2O were considered. The Eh–pH diagrams at different ion activity for V-S-H2O and Fe-K2SO4-H2O systems were drawn, as shown in Figure 9. Higher potential of the solution is conducive to vanadium dissolution. The oxidation potential of Fe3+/Fe2+ is higher than that of V2O3 and V2O4 minerals, so that those vanadium ores can be oxidized to leachable vanadium by Fe3+. At this range of pH, V2O5 is deliquescent and its extraction is workable when the dissolved V(V) ions do not reduce to VOSO4. The reduction of stability region of VOSO4 could concur with a decrease of vanadium ion activity. It could be seen that most of the stability zone of anions containing pentavalent vanadium ion locates in the area of iron precipitation. Meanwhile, the equilibrium potential of Fe3+/Fe2+ couple is determined by the ratio of ferric to ferrous ion. With the increment of Fe(II) concentration, the anode potential of Fe(III) moves to the negative direction. The probable reactions representing vanadium A. ferrooxidans leaching may be listed as [21, 22]:
(1)
(2)
(3)
(4)
(5)

(6)
Various factors contributed to the produce of jarosite but mainly to ion balance of Fe(III). Iron ion is through a series of hydrolysis to ferric hydroxide in aqueous medium with pH increasing. Under the required cation conditions, the hydroxide of iron gathers and grows with the bacteria for nucleation site and eventually forms jarosite in vitriol solution [23, 24]. A. ferrooxidans reinforces the formation of this precipitation due to the equilibrium between HSO4- and Fe3+ written as Eq.(12) [25].
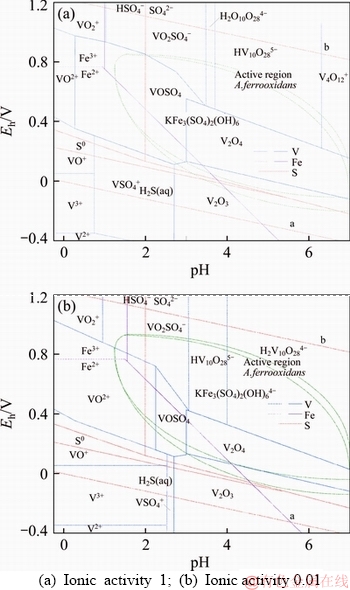
Figure 9 Eh-pH diagram for V-S-H2O and Fe-K2SO4- H2O system (at 25 °C):
(7)
(8)
(9)
(10)

(11)

(12)
where M+ is a monovalent cation, such as K+, Na+, NH4+, H3O+.
3.4.3 Cyclic voltammetry analysis
Figure 10 presents cyclic voltammograms of water-leaching vanadium residue in the different medium ranging from –1.2 V to 1.2 V (vs SCE). Two anodic peaks appeared at nearly –0.03 V and 0.26 V in blank solution. According to Eh-pH diagram for the V–S–H2O (Figure 9), the equilibrium potential of V2O4/V2O3 couple and HV2O5–/V2O3 couple were –0.06 V and 0.14 V at the pH of 1.8, respectively. A cathodic peak appeared at approximately –0.08 V could be attributed to V2O3/VO couple. However, the reversibility of those redox couples is not good. Hence, it is clarified that without oxidizing agent the dissolved vanadium is mainly from V(IV) minerals. Admixing A. ferrooxidans to the sulfuric acid solution, an obvious increasing of voltammetrical current could be observed,particularly, the anodic peak at 0.16 V suggests that A. ferrooxidans promotes the exchange of ore and solution.
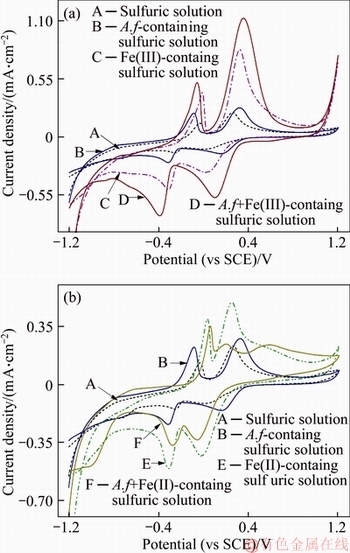
Figure 10 Cyclic voltammograms of residues in various solutions (A.f prepresents A.ferrooxidans)
Fe(III) expedites the redox of V(IV)/V(III), and V(V)/V(III) mineral couples (Figure 10(a)). With the presence of Fe(III), the reduction activation of ore electrode enhanced obviously. Two anodic peaks were detected approximately at 0.14 V and –0.42 V and the peak current are 3.94×10–4 A·cm–2 and 5.06×10–5 A·cm–2. This means that Fe(III) could oxidize low valence state of vanadium to high valence quickly. In addition, Fe(III) causes the reduction potential, observed at –0.25 V in control group, negatively shifted by 0.17 V, indicating that V(III) mineral is apt to turn into V(IV) and V(V) substances. Introducing A. ferrooxidans into Fe(III)-containing bath solution, vanadium could be dissolved faster due to the auxo-action of A. ferroxidans in electron exchange and material movement. The peak currents in both cathode and anode increased and the ratios of the peak current are more close to the value of 1 with the presence of A. ferrooxidans.
The results shown in Figure 10(b) indicate the effect of Fe(II) on clay vanadium flood residual slag bio-leaching. Fe(II) has a marked impact on the redox of vanadium minerals in bio-leaching process. Like Fe(III), Fe(II) facilitates the oxidation of low valence state vanadium assigned to a higher redox potential of Fe(III)/Fe(II) than that of vanadium matter. However, when there is A. ferrooxidans, the catalytic effect of Fe(II) is broken. It can be seen that an absorption peak appeared at 0.58 V attributed to a reductive product. The cathodic peak current shrunken as well as the anodic. The cycle efficiency of vanadium-containing couples decreased significantly. Moreover, the decrement of cathodic peak current of V(V) mineral at 0.3 V may be explained by the inhibition of mass transfer. Thus, it can be concluded that with the presence of Fe(II) A. ferrooxidans causes a precipitation and an absorption of iron compounds to happen to impede vanadium bio-leaching.
3.4.4 Polarization curve analysis
The results in Figure 11, show an increase in exchange current density and corrosion potential of water leaching vanadium residues with the employment of iron ion and A. ferrooxidans to leaching solutions. With the presence of Fe(III) ion, the corrosion potential increased 0.18 V to 0.59 V and the exchange current increased by 4 folds to 5.94×10–4 A·cm–2. The reduction current in Fe(III) solution is much higher than in sulfuric solution as a result that Fe(III)/Fe(II) oxidizes vanadium minerals. Fe(II) causes a drop in current density of oxidation because that Fe(II) is easier to oxidize than low valence of vanadium ore.
A. ferrooxidans evokes a negative movement of corrosion potential of vanadium ore electrode in Fe(III)-containing solution but opposite Fe(II)- containing solution. Admixing A.ferrooxidans to the Fe(III) medium, the corrosion potential decreases by 0.18 V and the corrosion current increases by a factor of 0.95. However, when introducing A. ferrooxidans into the Fe(II) solution, the corrosion potential increases 0.09 V with a lower corrosion current. It is found that A. ferrooxidans with Fe(III) promotes the vanadium leaching, but with Fe(II), it may obstruct the process.
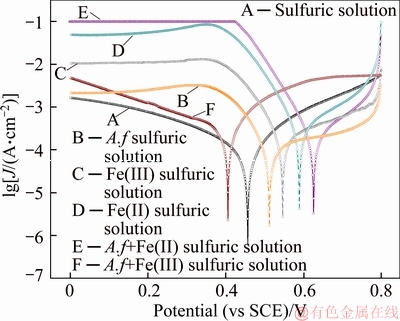
Figure 11 Polarization curves of residues in various solutions
4 Conclusions
1) A. ferrooxidans affects the vanadium extraction from clay vanadium via the influence on the cycle of Fe(II)/Fe(III) and the exchange of iron ion and deposits.
2) The passivation of iron compounds in vanadium A. ferrooxidans bio-leaching is closely related to the ferrous ion content in lixivium.
3) Fe(III) accelerates the redox of V(IV)/V(III), and V(V)/V(III) mineral couples. In Fe(III)- containing solution, A. ferrooxidans promotes the exchange of electron and substance. However, when the concentration of Fe(II) crosses a particular threshold in leaching solution, A. ferrooxidans is apt to oxidize Fe(II) into iron sedimentation which hinders the dissolution of vanadium.
4) A. ferrooxidans with Fe(III) increases vanadium leaching recovery and rate by 3.8% and 21.8% refer to using Fe(III) alone, respectively. The vanadium recovery of bio-leaching is 48.9%, with an overall increase of 18.5% over that of the control.
References
[1] YANG Q W, XIE Z M, PENG H, LIU Z H, TAO C Y. Leaching of vanadium and chromium from converter vanadium slag intensified with surface wettability [J]. Journal of Central South University, 2018, 25 : 1217–1325.
[2] LIU C, ZHANG Y M, BAO S X. Vanadium recovery from stone coal through roasting and flotation [J]. Transactions of Nonferrous Metals Society of China, 2017, 27: 197–203.
[3] JU Z J, WANG C Y, YIN F, YANG Y Q, LI D F. Process of vanadium extraction from stone coal vanadium ore by activated sulfuric acid leaching at atmospheric pressure [J]. Chinese Journal of Nonferrous Metals, 2012. 22: 2061–2068. (in Chinese)
[4] ZHANG X Y, QIN W Q, TIAN X D, CHEN Y B, GU Y, XI X G. Microwave roasting-acidic leaching technique for extraction of vanadium from stone coal [J]. Chinese Journal of Nonferrous Metals, 2011. 21: 908–912. (in Chinese)
[5] WU A X, HU K J, WANG H J, ZHANG A Q, YANG Y. Effect of ultraviolet mutagenesis on heterotrophic strain mutation and bioleaching of low grade copper ore [J]. Journal of Central South University, 2017, 24: 2245–2252.
[6] GOVENDER E, BRYAN C G, HARRISON S T L. Effect of physico-chemical and operating conditions on the growth and activity of Acidithiobacillus ferrooxidans in a simulated heap bio-leaching environment [J]. Minerals Engineering, 2015, 75 : 14–25.
[7] HAO X D, LIU X D, YANG Q, LIU H W, YIN H Q, QIU G Z, LIANG Y L. Comparative study on bioleaching of two different types of low-grade copper tailings by mixed moderate thermophiles [J]. Transactions of Nonferrous Metals Society of China, 2018, 28: 1847–1853.
[8] CHNE B W, WU B, LIU X Y, WEN J K. Effect of CO2, and N2, on microbial community changes during column bioleaching of low-grade high pyrite-bearing chalcocite ore [J]. Journal of Central South University, 2015, 22: 4528–4535.
[9] YU R L, SHI L J, ZHOU D, QIU G Z, ZENG W M. Research development of microorganism synergy mechanisms during bio-leaching [J]. Chinese Journal of Nonferrous Metals, 2013, 23: 3006–3014. (in Chinese)
[10] HUANG J, ZHANG Y M, HUANG J, LIU T, CAI Z L, XUE N N. Selective leaching of vanadium from roasted stone coal by dilute sulfuric acid dephosphorization-two-stage pressure acid leaching [J]. Minerals, 2016, 6: 75–87.
[11] LIAO M X, ZHANG X Y, ZHANG M X, DENG R, ZENG Z N. Study on the bio-leaching conditions of vanadium from vanadium-bearing stone coal by Acidthiobacillus ferrooxidans [J]. Fine Chemicals, 2015, 32: 144–148. (in Chinese)
[12] MISHRA D, KIM D J, RALPH D E, AHN J G, RHEE Y H. Bio-leaching of vanadium rich spent refinery catalysts using sulfur oxidizing lithotrophs [J]. Hydrometallurgy, 2007. 88: 202–209.
[13] LUO X B, FENG Y L, LI H R, DU Z W, WANG H J, LIU W. Investigation on extraction of V2O5 from clay containing vanadium by acid process [J]. Mining and Metallugical Engineering, 2007, 27: 48–53. (in Chinese)
[14] ZHU X B. Process and transfer behavior of extracting vanadium existed in muscovite from stone coal by activating roasting [D]. Wuhan: WuHan University of Technology, 2013. (in Chinese)
[15] LIU X W, FENG Y L, LI H R, YANG Z C, CAI Z L. Effect of Acidithiobacillus ferrooxidans on pyrite oxidation by ferric iron under aerobic and anaerobic conditions [J]. Chinese Journal of Nonferrous Metals, 2013, 23: 274–281. (in Chinese)
[16] SCHIPPERS A, JOZSA P, SAND W. Sulfur chemistry in bacterial leaching of pyrite [J]. Applied & Environmental Microbiology, 1996, 62: 3424–3431.
[17] SAND W, GEHRKE T, JOZSA P G, SCHIPPERS A. (Bio) chemistry of bacterial leaching-direct vs. indirect bio-leaching [J]. Hydrometallurgy, 2001, 59: 159–175.
[18] BROMBACHER C, BACHOFEN R, BRANDL H, Biohydrometallurgical processing of solids: A patent review [J]. Applied Microbiology & Biotechnology, 1997, 48: 577–587.
[19] CHEN J, GAO G Z, WANG Y, Microbial Hydrometallurgy [J]. Guangdong Chemical Industry, 2013, 40: 98–99. (in Chinese)
[20] DAOUD J, KARAMANEV D. Formation of jarosite during Fe2+ oxidation by Acidithiobacillus ferrooxidans [J]. Minerals Engineering, 2006, 19: 960–967.
[21] PARAMGURU R K, MISHRA K G, KANUNGO S B. Electrochemical phenomena in MnO-FeSLeaching in dilute HCl. Part 2: Studies on polarization measurements [J]. Canadian Metallurgical Quarterly, 1998, 37: 395–403.
[22] TRIBUTSCH H. Direct versus indirect bio-leaching [J]. Hydrometallurgy, 1999, 9: 51–60.
[23] DEMPERS C J N, BREED A W, HANSFORD G S. The kinetics of ferrous-iron oxidation by Acidithiobacillus ferrooxidans and Leptospirillum ferrooxidans: Effect of cell maintenance [J]. Biochemical Engineering Journal, 2003, 16: 337–346.
[24] NAZARI B, JORJANI E, HANI H, MANAFI Z, RIAHI A. Formation of jarosite and its effect on important ions for Acidithiobacillus ferrooxidans bacteria [J]. Transactions of Nonferrous Metals Society of China, 2014, 24: 1152–1160.
[25] BASCIANO L C. Crystal chemistry of the jarosite group of minerals-solid solution and atomic structures [D]. Kingston, Ontario: Queen’s Unveristy, 2008.
(Edited by HE Yun-bin)
中文导读
铁转化对氧化亚铁硫杆菌生物浸出黏土钒渣的影响
摘要:本文研究了黏土钒矿水浸渣的生物提钒工艺,考察了浸出过程中铁转化对钒生物浸出的影响。氧化亚铁硫杆菌通过催化Fe3+/ Fe2+电对循环及物质交换影响钒溶解;过程中铁的沉积与体系亚铁离子含量有关。在含有氧化亚铁硫杆菌和Fe(III)介质中,Fe(II)浓度的增加导致黄钾铁矾的形成,从而致使钒浸出效率下降。循环伏安分析表明,氧化亚铁硫杆菌易氧化Fe(II)离子并转化为沉淀物,强烈吸附在浸渣表面。Fe(III)离子因其高氧化活性而促进钒浸出。含氧化亚铁硫杆菌和Fe(III)溶浸体系可提高低价态钒物种还原,加速矿物与溶液间的物质交换,较仅含Fe(III)体系钒的回收率和浸出率分别提高3.8%和21.8%。此外,Fe(II)离子在无菌介质中对钒提取影响较小,而有菌存在时负向影响钒浸出。
关键词:铁离子循环;氧化亚铁硫杆菌;钝化;循环伏安法;黏土钒矿
Foundation item: Project(DY135-B2-15) supported by the China Ocean Mineral Resource R&D Association; Project(2015ZX07205-003) supported by Major Science and Technology Program for Water Pollution Control and Treatment, China; Projects(21176242, 21176026) supported by the National Natural Science Foundation of China
Received date: 2018-04-01; Accepted date: 2018-11-03
Corresponding author: LI Hao-ran, PhD, Professor; Tel: +86-13701399765; E-mail: hrli@ipe.ac.cn; ORCID: 0000-0002-5165-4819