
ARTICLE
J. Cent. South Univ. (2019) 26: 393-403
DOI: https://doi.org/10.1007/s11771-019-4011-0

Effect of substrate amendment on alkaline minerals and aggregate stability in bauxite residue
TIAN Tao(田桃), KE Wen-shun(可文舜), ZHU Feng(朱锋), WANG Qiong-li(王琼丽),YE Yu-zhen(叶羽真), GUO Ying(郭颖), XUE Sheng-guo(薛生国)
School of Metallurgy and Environment, Central South University, Changsha 410083, China
Central South University Press and Springer-Verlag GmbH Germany, part of Springer Nature 2019
Abstract: Bauxite residue is an alkaline waste material in the process of alumina production due to its characteristics of higher salinity and alkalinity, which results in environmental issues and extremely restricts the sustainable development of alumina industries. In this work, we conduct a column experiment to study the effects of two amendments on aggregate stability and variations in alkaline minerals of bauxite residue. The two amendments are phosphogypsum (PG) and phosphogypsum and vermicompost (PVC). The dominant fraction in aggregate is 1–0.25 mm in diameter on the surface, which takes up 39.34%, 39.38%, and 44.51 % for CK, PG, and PVC, respectively. Additions of PG and PVC decreased pH, EC, ESP, exchangeable Na+ concentration and the percentage of alkaline minerals, and then increased exchangeable Ca2+ concentration in bauxite residue. There was significant positive correlation between pH and exchangeable Na+ concentration, the percentage of cancrinite, tricalcium aluminate and calcite; while negative correlation was found in pH value versus exchangeable Ca2+ concentration. Theses findings confirmed that additions of phosphogypsum and vermicompost have a stimulative effect on aggregate stability in bauxite residue. In particular, amendment neutralization (phosphogypsum + vermicompost) in column represents an advantage for large-scale simulation of vegetation rehabilitate in bauxite residue disposal areas.
Key words: bauxite residue; substrate amendment; alkaline minerals; aggregate stability; soil formation in bauxite residue
Cite this article as: TIAN Tao, KE Wen-shun, ZHU Feng, WANG Qiong-li, YE Yu-zhen, GUO Ying, XUE Sheng-guo. Effect of substrate amendment on alkaline minerals and aggregate stability in bauxite residue [J]. Journal of Central South University, 2019, 26(2): 393–403. DOI: https://doi.org/10.1007/s11771-019-4011-0.
1 Introduction
China is the largest producer of the alumina in the world, and the output of bauxite residues reached 100 million tons per year, as well as the accumulative storage of bauxite residue was over 600 million tons in 2018. In the world, the current best practice in the industry is disposal in engineered bauxite residue disposal areas, increasingly using dry staking methods [1–4]. The dry bauxite residues are concerning due to the potential influence of numerous fugitive dust, which can emit a amounts of alkaline particle into the surrounding environment, and affect the deep regions of the lung of animals [5–8]. Meanwhile, bauxite residue is found to own the characteristics of high pH, high salinity, low permeability and, in particular, poor aggregate structure [9], which restricts plant growth in bauxite residue disposal areas. Soil formation of bauxite residue is mainly the transformation process by which bauxite residue can be transformed a soil-like media, meeting the environmental needs of vegetation restoration [10, 11]. Thus, it is curial issue that revegetation is deemed to the extremely important effective measure way to bauxite residue disposal areas, and physico-chemical evaluation can be used for the major indicator of rehabilitation success.
Recently, some approaches have been demonstrated to improve physical and chemical properties of bauxite residue such as soil capping, halophytes, microbial and amendments [12–14]. However, among them, amendments exhibited a promising method owing to its advantage of low-cost, extensive source, high efficiency, and rendered the concentration of the hydrogen ion. A variety of amendments, including seawater, phosphogypsum, sulfuric acid, gypsum, hydrochloric acid, organic compounds and carbon dioxide [15–17], have been reported, which brought advancement in helping neutralization of alkaline substance. Phosphogypsum, an acidic waste produced in the phosphate fertilizer industry, can effectively deplete alkaline substance. Moreover, phosphogypsum is one of the most extensive amendments to reduce the pH of bauxite residues. pH reduction neutralization by adding phosphogypsum was originated from acid neutralization, which was due to Ca2+ removed alkaline anions from solution by precipitation reaction [18]. It should be noted that phosphogypsum could also improve quality and productivity of high-magnesium soils [19]. Moreover, gypsum transformation of bauxite residue could reduce the pH by precipitating OH–, Al(OH)4– and CO32–, which caused formation of Ca(OH)2, tri-calcium aluminate (TCA, Ca3Al2(OH)12), hydrocalumite (Ca4Al2(OH)12·CO3) and calcite (CaCO3) [20]. Vermicompost, an organic manure, belongs to a waste material in agriculture field. Recent decades, organic manure has been reported to ameliorate soil properties, i.e. soil structure, aggregate stability and chemical and biological properties [13]. Vermicompost increased wet aggregate stability and air permeability, while decreased bulk density and penetration resistance [14]. It provided an avenue for vermicompost application to improve soil physical characteristics. GOSWAMI et al [21] also conducted field experiments by cultivating tomato and cabbage, and demonstrated the improvement of vermicompost on the soil quality and crop growth. The amendments play an important role in nutrient availability, physical stability, and microbial diversity of bauxite residue. Indeed, the combination of gypsum and organic manure has generally been observed to be extremely effective measure to reclaim bauxite residue disposal areas [10, 22]. ZHU et al [22] evaluated the characteristics of the aggregate formation in bauxite residue by adding vermicompost and gypsum, which indicated that the aggregate size distribution and structure are positively correlated to the combination of vermicompost and gypsum. However, the combination of vermicompost and gypsum has been conducted by pot experiments, which limited its large-scale industry applications and technologically improvement. Meanwhile, mineral transformations have been ignored although they played an important role in aggregate formation in bauxite residue [5].
In this work, we carried out the column experiment to investigate the change of alkaline substance in bauxite residues treated by the combination of phosphogypsum and vermicompost. The characteristics of the mineral transformation were also investigated by X-ray diffraction (XRD). In addition, effect of phosphogypsum and vermicompost on aggregate stability of bauxite residue was systematically studied.
2 Materials and methods
2.1 Sample preparation
Bauxite residue samples, beneath of ground surface 0–20 cm, were collected and located in a bauxite residue disposal area of the aluminum corporation, Guangxi Province, China (23°54'627"N, 108°18'200"E). The bauxite residue disposal areas have a typical subtropical monsoonal climate, where the mean annual temperature is 11–17 °C and mean annual rainfall is 1300 mm. Bauxite residue samples were air-dried and screened using a 2-mm nylon sieve. So, it could be homogenized. Finally, the physical and chemical properties were analyzed. Phosphogypsum was provided by a commercial company in Hunan Province, China. Vermicompost was obtained from a farm in Jiangsu Province, China. The compost originated from the decomposition of cow dung through earthworm activity, then it was air-dried and sieved below 2 mm. The main properties of the phosphogypsum and vermicompost are shown in Table 1.
Table 1 Physico-chemical properties and element concentration of bauxite residue and amendments
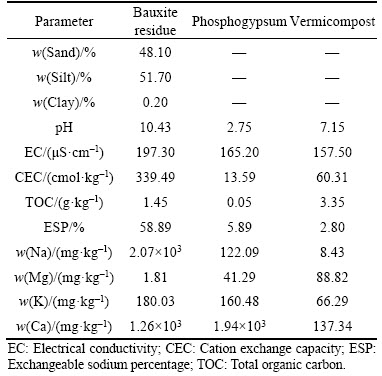
2.2 Experimental design
The experiment was replicated three times and included three treatments, namely, 1) controlling, abbreviated as CK, 2) addition of phosphogypsum (2% v/v), abbreviated as PG, 3) addition of phosphogypsum and vermicompost (2% and 4% v/v), abbreviated as PVC. Nine columns were established from the plexiglass cylinders (12 cm in inner diameter, 14 cm in external diameter, and 100 cm in height). A 15 cm filter layer of quartz sand was placed above a piece of 150 μm nylon mesh at the bottom of each column. A layer of 60 cm of untreated bauxite reside was then filled with column (middle, 25–45 cm and bottom, 45–60 cm). Amendments were thoroughly mixed with bauxite residue and then added in a layer 25 cm deep above the untreated layer (surface, 0–25 cm). All columns were saturated with demonized water and then stabilized for 48 h. The columns were incubated for a further one month and leached with 175 mm every two days (the average monthly rainfall in the locality of the industry). The mean temperature and relative humidity were 26.5–27.6 °C and 65.4%– 72.1% in laboratory, respectively.
2.3 Physical and chemical properties analysis
At the end of the incubation, bauxite residue samples were collected in each column at the depth of 0–25 cm, 25–45 cm, and 45–65 cm, respectively, in total 27 samples. The samples were air-dried and preserved for physical and chemical analysis. The size distribution of bauxite residue aggregate was measured by dry sieve analysis method. The five analysis data represent different particle size distributions of bauxite residue, in the order of >2 mm, 2–1 mm, 1–0.25 mm, 0.25–0.05 mm,<0.05 mm. Different fractions collected from diverse sieves were weighed and reserved. The pH and electrical conductivity (EC) of samples were determined in a 1:5 ratio (mass/volume) extracting solution after 30 min of stirring and stored. Exchangeable cations concentrations (Na+, Ca2+, Mg2+ and K+) were extracted with 1 mol/L ammonium acetate (pH=7), and were measured using inductively coupled plasma atomic emission spectrophotometry (ICP-AES). Organic carbon was determined by the low-temperature external-heat potassium dichromate oxidation colorimetric method [29]. The samples were oven-dried at 65°C and sieved to retain the <0.38 μm fraction prior to X-ray powder diffraction (XRD) analysis conducted on a Bruker D8 discover 2500 with Cu Kα1 tube using a Sol-X detector (LynxEye array). XRD analysis used the PANalytical analysis package to identify and quantify phases. The relative intensity ratio method was used to quantitatively analyze mineral phases [4].
2.4 Data analysis
The mean weight diameter (MWD) and geometry weight diameter (GWD) of each sample were calculated as follows:
(1)
(2)
where
is mean diameter of each size fraction and Wi is the proportion of the total sample weight corresponding to size fraction. Exchangeable sodium percentage (ESP) was calculated using:

(3)
The statistical significance of experimental treatments was conducted by using the Microsoft Excel 2003 and Origin 8.0. Differences were analyzed by using Dunnett’s test in SPSS at the 5% significance level. The relationships among pH, EC, and exchangeable cation for alkaline minerals in bauxite residue were fitted to linear regression functions.
3 Results and discussion
3.1 Distribution and stability of aggregate
The aggregation process of bauxite residue added with amendments was analyzed as shown in Figure 1, and their aggregates distributions as well as stability are shown in Figure 2. It can be seen from Figure 1, the aggregate fractions of 1–0.25 mm in diameter accounted for the largest proportion of bauxite residue aggregates, and the fractions <0.05 mm in diameter take up the smallest proportion in the surface, middle, and bottom position. The dominant fraction in aggregate is 1–0.25 mm in diameter in the surface of 0–25 cm, which takes up 39.34%, 39.38%, and 44.51% for CK, PG, and PVC, respectively. Moreover, in comparison with CK, addition of PG and PVC increased the aggregate fraction at the size of 1–0.25 mm. The similar results were also found at the 25–45 cm and 45–65 cm depth of bauxite residue. Therefore, these experiments revealed that macro-aggregates (1–0.25 mm) were coagulated from micro-aggregates (<0.05 mm) with adding to amendments. Meanwhile, the changes of bauxite residue aggregates distribution could improve the bauxite residue structure. The above results were accordant with the previous study, which demonstrated that addition of amendments could accelerate formation of aggregate in bauxite residues [21]. Based on these findings, it can be concluded that addition of amendments is one of the major factors contributing to improving physical properties of bauxite residue, which facilitated vegetation reconstruction in bauxite residue disposal areas [23].
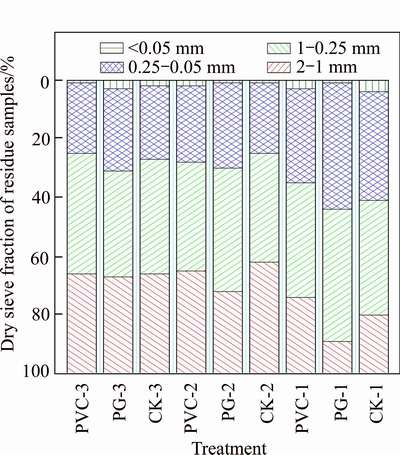
Figure 1 Dry sieve fraction of bauxite residue with different amendment treatments
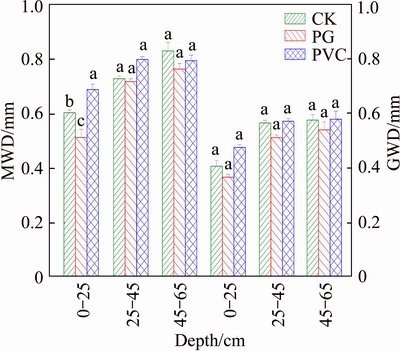
Figure 2 Changes in mean weight diameter (MWD) and Geometric mean weight diameter (GWD) of bauxite residue with different amendment treatments
Our results indicated that the macro-aggregate proportion in PVC on the surface of 0–25 cm profile, accounting for 99.85% in the total aggregate proportion for 2–1 mm, 1–0.25 mm, and 0.25–0.05 mm ranges, was higher than PG (97.35%). Also, the aggregate stability of MWD and GWD in PVC was higher than PG. The values of MWD and GWD in PVC were higher than PG for the same depth under three different depth levels. These experiments demonstrated that vermicompost has a positive effect on aggregate formation [14], and it could also increase the aggregate cohesion [15]. Studies demonstrated that vermicompost has the peat-like characteristics, such as high- concentration humid substances, abundant organic acids, sufficient porosity, and large specific surface area as well as superior microbial activity, which facilitated the aggregation formation and enhanced the aggregate stability. In addition, phosphogypsum can optimize particulate agglomeration and permeability [12]. Therefore, the combinations of phosphogypsum and vermicompost (PVC) can accelerate and facilitate the aggregate stability in bauxite residue disposal areas.
3.2 pH and EC
The pH values of CK, PG, and PVC, ranging in 0–25 cm on the surface of bauxite residue, were lower than those at the depth of 25–65 cm, as shown in Figure 3(a). The 25–45 cm depths represent a transition zone between the amendments and bauxite residue, where PG and PVC can be washed into the solution of bauxite residue. No significant changes could be found for the pH value in bauxite residue after addition of amendments at the 45–65 cm depth, which mainly occurred at the fractions of 0.25–0.05 mm and <0.05 mm. The reasonable speculation is that the introduction of phosphogypsum can result in lower pH due to the dissolution and migration of Ca2+, then Ca2+ transfers to solution as well as reacts with OH–, Al(OH)4– and CO32–[4].
Figure 3(b) implies the EC value changes for different amendment treatments at different depths. The EC values of adding PG and PVC treatments were lower than CK on the surface of 0–25 cm depth, where the EC was lower than that at the depth ranges of 25–45 cm and 45–65 cm. Moreover, the EC was the highest at the 45–65 cm depth, as shown in Figure 3(b). The EC value of CK treatment was 510 μS/cm at the 0–25 cm depth, while the EC value of PG and PVC only reached 188 and 171 μS/cm, respectively. The highest EC occurs at the 45–65 cm depth, and the EC value at different amendment treatments exhibits the order of CK>PG>PVC. The EC value for CK, PG, and PVC is 1119, 976, and 170 μS/cm, respectively. The EC value rises with increasing the depth, which demonstrates that cation and anion transfer from upper to lower sites at the dissolution process.
3.3 Exchangeable cation (Na+, Ca2+, K+, Mg2+) concentrations
The exchangeable Na+ concentration was the lowest in the 0–25 cm surface of profiles, and its value increased with increasing depth at the 25–65 cm, as shown in Figure 4(a). In comparison with CK treatment, exchangeable Na+ concentration was significantly reduced under PG and PVC treatments (P<0.05), which could be ascribed to hat displacement reaction of Na+ displaced by released Ca2+ occurring in the exchange system and the addition of phosphogypsum improved Ca2+ availability [24]. Further, compared with CK, the PG and PVC treatments reduced exchangeable Na+ concentration by about 57.32% and 60.78%, respectively.
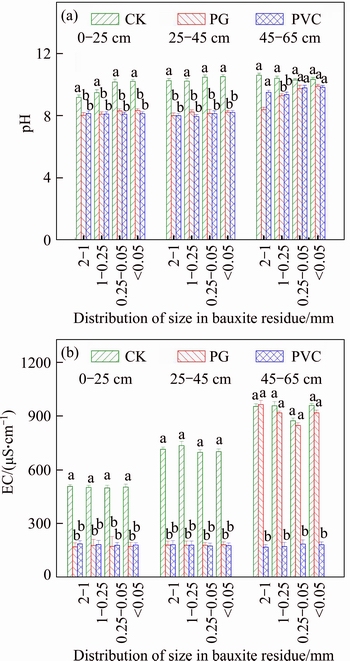
Figure 3 Comparison of pH (a) and EC (b) in bauxite residue with different amendment treatments
In comparison with CK the exchangeable Ca2+ concentration in bauxite residue was higher after amendment treatments (PG and PVC), as shown in Figure 4(b).The amendment effect on the exchangeable Ca2+ concentrations of bauxite residue is in the order of PVC>PG>CK. Also, the exchangeable Ca2+ concentration in bauxite residue treated by PG and PVC were 1.47×103 and 1.48×103 mg/kg, respectively. These results indicate that vermicompost can effectively increase the exchangeable Ca2+ concentrations in bauxite residue, which facilitates the Na+ displacement from exchange positions and enhances rehabilitation in bauxite residue disposal areas. JONES et al [25] reported similar cases that the exchangeable Ca2+ concentration in bauxite residue increased when the leaching bauxite residue was treated by amendment of poultry manure and PG combination. In addition, JALALI et al [26] found that the soil extractable Ca2+ concentrations in saline sodic soils significantly increased due to the combination treatments of gypsum, sheep and poultry manure.
The exchangeable K+ concentration in bauxite residue by PVC treatment was lower than that by the PG and CK treatments at the surface layer of 0–25 cm depth, as shown in Figure 4(c). Moreover, the exchangeable K+ concentration in bauxite residue declined at the surface layer (0–25 cm), where the PG was applied into the surface. At the 25–45 cm and 45–65 cm depth, no PG applied in subsurface of soils and the exchangeable K+ concentration in bauxite residue increased.Figure 4(d) indicates that exchangeable Mg2+ concentrations in bauxite residue was extremely low, and its value in bauxite residue under PVC treatment was significantly greater than that by CK and PG treatments at the surface layer. This result can be ascribed to the fact that the increase of Ca2+ concentrations is in favor of adsorption on the exchange positions of the sodic soils in comparison with exchangeable Mg2+ concentration [26].
3.4 ESP in bauxite residue
The variations of bauxite residue exchangeable sodium percentages (ESP) under different treatments are shown in Figure 5. In comparison with CK treatment the reduction degrees of ESP in bauxite residue under PG and PVC amendments were significant at the surface depth (0–25 cm). This result can be ascribed to the fact that large concentration of Ca2+ is presented in the PG treatment.The addition of PVC is in favor of the dissolution of native calcite, which causes Ca2+ contribution and enhances the Na+_Ca2+ exchange proportion between the solution and exchange phase. Reductions in bauxite residue ESP were also reported by previous studies through gypsum and other organic amendment application [22].
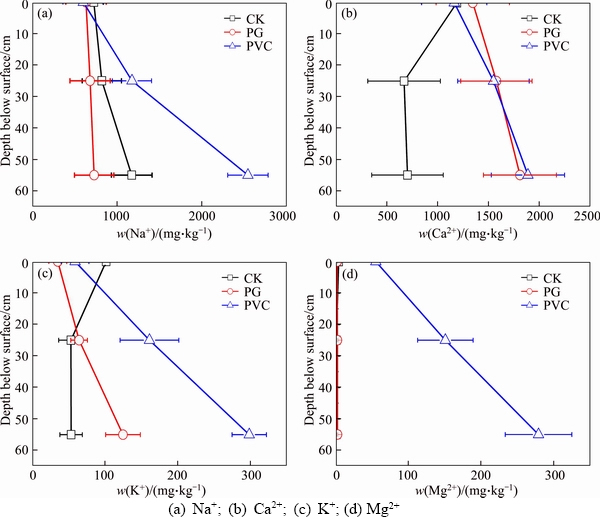
Figure 4 Exchangeable cation concentrations in depth below surface of bauxite residue:(Mean ± standard error values are shown (n=3))
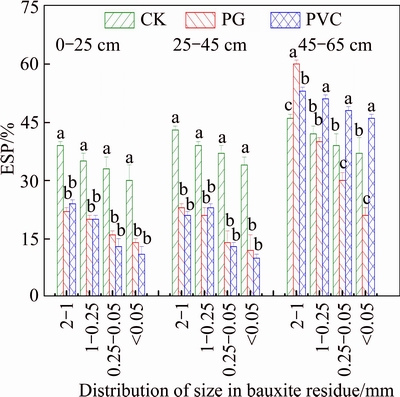
Figure 5 ESP comparison in bauxite residue with different amendment treatments (Mean ± standard error values are shown (n=3))
3.5 Mineral composition
Bauxite residue contains many mineral phases, including hematite, katoite, calcite, diaspore, cancrinite, gibbsite, and tricalcuium aluminate, which were confirmed from XRD results (as shown in Figure 6) and was composed of crystalline structure phase about 70% and 30% amorphous materials in bauxite residue [26]. The characterization of CK, PG and PVC and their quantities using PANalytical analysis from XRD results are presented in Table 2. It can be seen from XRD results (Figure 6 and Table 2) that abundant hematite amounts of 21.00%–29.94% are examined in all treatments and the amounts have no significant variation according to the quantitative identification. The occurrence with hematite transformation is to meet the requirements both enough H+ and appropriate amounts of soluble and solid-phase alkalinities presented in the bauxite residues. Hematite could effectively affect acid neutralizing capacity (ANC) occurring in bauxite residue and caused pH modulation in the range of 4–5 [27, 28]. No mineral phase transformation was observed in gibbsite and diaspore, which illustrated that it was difficult to cause phase transformation by phosphogypsum and vermicompost in minerals of gibbsite and diaspore due to its existing form of stable structure. The fastest amount change occurred in cancrinite in all treatments, which was attributed to the fact that the cancrinite was a typical alkalinity material and the chemical reaction occurred in the process of leaching. Also, the high alkalinity ions such as OH–, CO32–, and Al(OH)– could be washed out and then pH value increased in the process of leaching:
Na6Ca2Al6Si6O24CO3(H2O)2+16H2O+6H+→6Na++2Ca2++6Al(OH)3+6H4SiO4+2CO32– (4)
In comparison with the cancrinite concentration in CK (19.75%), this value decreased in PG (10.61%) and PVC (10.32%) due to more cancrinite transformed into solution in acid environment after adding amendments. The tricalcium aluminate (TCA) amounts, by the treatment of CK, PG, and PVC, are 38.33%, 25.98%, and 25.21% on the surface of 0–25 cm, respectively, which indicated that the TCA amounts by PG and PVC treatments are significantly lower than those by CK treatment. The above results demonstrated that the addition of amendments plays a role in mineral phase transformation in bauxite residue, which is in accordance with similar reports [4]. Katoite (Ca3Al2(SiO4)(OH)8) is also an alkaline mineral, where the reaction (5) is easily to occur in the process of leaching. According to reactions(4–6), alkaline minerals dissolve lots of alkaline ions such as OH–, CO32– and Al(OH)–, which increases alkalinity in bauxite residue. However, addition of PG and PVC could decrease alkalinity in bauxite residue. The reasons is that, on one hand, phophogypsum is acid material and vermicompost contains a lot of humic acid, which can create H+ reacting with alkaline ions. On the other hand, phophogypsum continues to supply Ca2+, and Ca2+ can react with alkaline ions to create precipitate reaction as well as displacement reaction with cations.
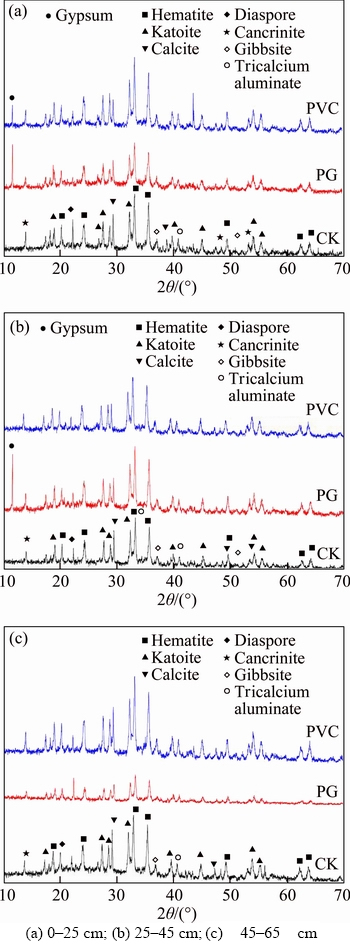
Figure 6 X-ray diffraction patterns of bauxite residue samples with different depth:(CK: Without amendment; PG: Phosphogypsum; PVC: Phosphogypsum+vermicompost)
Table 2 Mineral composition of bauxite residue and transformed bauxite residue using different treatments
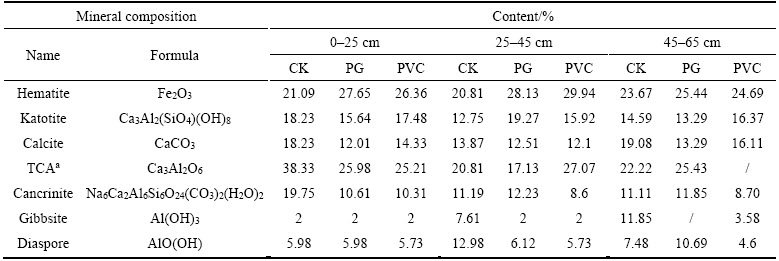
Ca3Al2(SiO4)(OH)8+4H2O→3Ca2++2Al(OH)3+6OH–+H4SiO4 (5)
Ca3Al2O6+6H2O→3Ca2++2Al(OH)3+6OH– (6)
OH–+H+→H2O (7)
Al(OH)4–+H+→H2O+Al(OH)3 (8)
CO32–+H+→HCO3– (9)
Ca2++CO32–→CaCO3 (10)
Ca2++2OH–→Ca(OH)2 (11)
3Ca2++2Al(OH)4–+4OH–→Ca3Al2(OH)12 (12)
Na2CO3+CaSO4→CaCO3+Na2SO4 (13)
3.6 Correlation among pH, EC and Cation, alkaline minerals in bauxite residue
The correlation analysis from Figure 7(a) revealed that positive correlation was performed in pH value versus exchangeable Na+ concentration (R=0.667, P<0.05), while negative correlation was found in pH value versus exchangeable Ca2+ concentration (R=0.886, P<0.01). The pH value was positively correlated to the percentage of cancrinite, TCA and calcite (R=0.773, 0.773 and 0.768, respectively) in Figure 7(b). We could conclude that pH was variable in the bauxite reside because the solution pH of the bauxite residues was buffered by alkaline minerals [36]. In addition, the alkaline anions in bauxite residue solution are mainly OH–, CO32–/HCO3–, Al(OH)4–/Al(OH)3 and H2SiO42–/H3SiO4–, which increases pH by dissolution of alkaline minerals in bauxite residue. The EC value was positively correlated to exchangeable Na+ concentration (R=0.672, P<0.05), and negatively correlation was found in EC versus exchangeable Ca2+ concentration (R=–0.993, P<0.01), as shown in Figure 8(a). There was no significant correlation in EC versus exchangeable Mg2+ or K +concentration, which was consistent with existing study [20]. GR
FE et al [20] reported that exchangeable K+ and Mg2+ as well as other cations are difficult to affect EC variation, which is attributed to the fact that the concentration of cations can be negligible in solution. The concentration of Na+ exceeding Mg2+ about 2–4 orders of magnitude was reported [20]. The EC value was positively correlated to the percentage of cancrinite, TCA and calcite (R=0.904, 0.873 and 0.818, P<0.01, respectively), as shown in Figure 8(b).
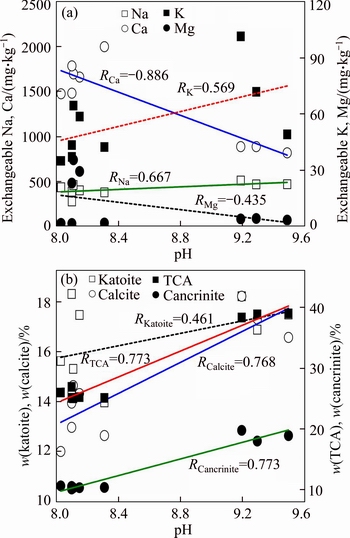
Figure 7 Correlations of pH versus exchangeable cation ((Na+, Ca2+, K+, Mg2+) concentrations (a) and pH versus alkaline minerals (katoite, calcite, TCA, cancrinite) in bauxite residue below 0–25 cm (b)
4 Conclusions
The dominant fraction in aggregate is 1–0.25 mm in diameter on the surface of 0–25 cm, which takes up 39.34%, 39.38%, and 44.51% for CK, PG, and PVC, respectively. Additions of PG and PVC decreased pH, EC, ESP, exchangeable Na+ concentration and the percentage of alkaline minerals, and then increased exchangeable Ca2+ concentration in bauxite residue. Further study found that there were significant positive linear relations between pH value and exchangeable Na+ concentration, the percentage of cancrinite, TCA and calcite in bauxite residue, but there were significant negative linear relations between pH value and exchangeable Ca2+ concentration. It can be concluded that additions of phosphogypsum and vermicompost not only effectively improve fundamentally physical and chemical properties but also have a stimulative effect on soil formation in bauxite residue, which provides a favorable and technological way for vegetation reconstruction in bauxite residue disposal areas.
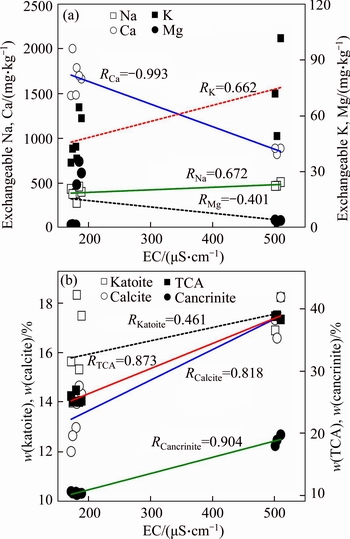
Figure 8 Correlations of EC versus exchangeable cation (Na+, Ca2+, K+, Mg2+) concentrations (a) and EC versus alkaline minerals (katoite, calcite, TCA, cancrinite) in bauxite residue below 0–25 cm (b)
References
[1] XUE Sheng-guo, KONG Xiang-feng, ZHU Feng, HARTLEY W, LI Xiao-fei, LI Yi-Wei. Proposal for management and alkalinity transformation of bauxite residue in China [J]. Environmental Science and Pollution Research, 2016, 23(13): 12822–12834. DOI: 10.1007/s11356-016- 6478-7.
[2] ZHU Feng, CHENG Qing-yu, XUE Sheng-guo, LI Chu-xuan, HARTLEY W, WU Chuan. TIAN Tao. Influence of natural regeneration on fractal features of residue microaggregates in bauxite residue disposal areas [J]. Land Degradation and Development, 2018, 29(1): 138–149. DOI: 10.1002/ldr.2848.
[3] ZHU Feng, LIAO Jia-xin, XUE Sheng-guo, HARTLEY W, ZOU Qi, WU Hao. Evaluation of aggregate microstructures following natural regeneration in bauxite residue as characterized by synchrotron-based X-ray micro-computed tomography [J]. Science of the Total Environment, 2016, 573: 155–163. DOI: 10.1016/j.scitotenv.2016.08.108.
[4] KONG Xiang-feng, LI Meng, XUE Sheng-guo, HARTLEY W, CHEN Cheng-rong, WU Chuan, LI Xiao-fei, LI Yi-wei. Acid transformation of bauxite residue: Conversion of its alkaline characteristics [J]. Journal of Hazardous Materials, 2017, 324: 382–390. DOI: 10.1016/j.jhazmat.2016.10.073.
[5] KONG Xiang-feng, TIAN Tao, XUE Sheng-guo, HARTLEY W, HUANG Long-bing, WU Chuan, LI Chuan-xuan. Development of alkaline electro-chemical characteristics demonstrates soil formation in bauxite residue undergoing natural rehabilitation [J]. Land Degradation and Development, 2018, 29: 58–67. DOI: 10.1002/ldr.2836.
[6] WU Chuan, HUANG Liu, XUE Sheng-guo, HUANG Yu-ying, HARTLEY W, CUI Meng-qian, WONG Ming-hung. Arsenic sorption by red mud-modified biochar produced from rice straw [J]. Environmental Science and Pollution Research, 2017, 24: 18168–18178. DOI: 10.1007/ s11356-016-9466-7.
[7] XUE Sheng-guo, WU Yu-jun, LI Yi-wei, KONG Xiang-feng, ZHU Feng, WILLIAM H, LI Xiao-fei, YE Yu-zhen. Industrial wastes applications for alkalinity regulation in bauxite residue: A comprehensive review [J]. Journal of Central South University, 2019, 26(2): 268–288.
[8] XUE Sheng-guo, ZHU Feng, KONG Xiang-feng, WU Chuan, HUANG Ling, HUANG Nan, HARTLEY W. A review of the characterization and revegetation of bauxite residues (red mud) [J]. Environmental Science and Pollution Research, 2016, 23: 1120–1132. DOI: 10.1007/s11356-015- 4558-8.
[9] WANG Jun, CHENG Qing-yu, XUE Sheng-guo, RAJENDRAN M, WU Chuan, LIAO Jia-xin. Pollution characteristics of surface runoff under different restoration types in manganese tailing wasteland [J]. Environmental Science and Pollution Research, 2018, 10: 9998–10005. DOI: 10.1007/s11356-018-1338-2.
[10] ZHU Feng, ZHOU Jia-yi, XUE Sheng-guo, HARTLEY W, WU Chuan, GUO Ying. Aging of bauxite residue in association of regeneration: A comparison of methods to determine aggregate stability and erosion resistance [J]. Ecological Engineering, 2016, 92: 47–54. DOI: 10.1016/ j.ecoleng.2016.03.025.
[11] XUE Sheng-guo, YE Yu-zhen, ZHU Feng, WANG Qiong-li, JIANG Jun, HARTLEY W. Changes in distribution and microstructurre of bauxite residue aggregates following amendments addition [J]. Journal of Environment Sciences, 2019, 78: 276–286. DOI: 10.1016/j.jes.2018.05.016.
[12] XUE Sheng-guo, LI Meng, JIANG Jun, MILLAR G J, LI Chu-xuan, KONG Xiang-feng. Phosphogypsum stabilization of bauxite residue: Conversion of its alkaline characteristics [J]. Journal of Environmental Sciences, 2019, 77: 1–10. DOI: 10.1016/j.jes.2018.05.016.
[13] COURTNEY R, HARRINGTON T. Growth and nutrition of Holcus lanatus, in bauxite residue amended with combinations of spent mushroom compost and gypsum [J]. Land Degradation and Development, 2012, 23(2): 144–149. DOI: 10.1002/ldr.1062.
[14] AKSAKAL E L, SARI S, ANGIN I. Effects of vermicompost application on soil aggregation and certain physical properties [J]. Land Degradation and Development, 2016, 27(4): 983–995. DOI: 10.1002/ldr.2350.
[15] CLARK M W, JOHNSTON M, REICHELT-BRUSHETT A J. Comparison of several different neutralisations to a bauxite refinery residue: Potential effectiveness environmental ameliorants [J]. Applied Geochemistry, 2015, 56: 1–10. DOI: 10.1016/j.apgeochem.2015.01.015.
[16] JOHNSTON M, CLARK M W, MCMAHON P, WARD N. Alkalinity conversion of bauxite refinery residues by neutralization [J]. Journal of Hazardous Materials, 2010, 182(1): 710–715. DOI: 10.1016/j.jhazmat.2010.06.091.
[17] BURKE I T, PEACOCK C L, LOCKWOOD C L, STEWART D I, MORTIMER R J, WARD M B, RENFORTH P, GRUIZ K, MAYES W M. Behavior of aluminum, arsenic, and vanadium during the neutralization of red mud leachate by HCl, gypsum, or seawater [J]. Environmental Science and Technology, 2013, 47(12): 6527–6535. DOI: 10.1021/ es4010834.
[18] MAHMOUD E, ABD EI-KADER N. Heavy metal immobilization in contaminated soils using phosphogypsum and rice straw compost [J]. Land Degradation and Development, 2015, 26(8): 819–824. DOI: 10.1002/ldr.2288.
[19] QADIR M, NOBLEA D, SCHUBER S, THOMAS R J, ARSLAN A. Sodicity-induced land degradation and its sustainable management: problems and prospects [J]. Land Degradation and Development, 2010, 17(6): 661–676. DOI: 10.1002/ldr.751.
[20] GR
FE M, KLAUBER C. Bauxite residue issues IV.: Old obstacles and new pathways for in situ residue bioremediation [J]. Hydrometallurgy, 2011, 108: 46–59. DOI: 10.1016/j.hydromet.2011.02.005.
[21] GOSWAMI L, NATH A, SUTRADHAR S, BHATTACHARYA S S, KALAMDHAD A, VELLINGIRI K, KIM K H. Application of drum compost and vermicompost to improve soil health, growth, and yield parameters for tomato and cabbage plants [J]. Journal of Environmental Management, 2017, 200(15): 243–252. DOI: 10.1016/ j.jenvman.2017.05.073.
[22] ZHU Feng, HOU Jinag-tao, XUE Sheng-guo, WU Chuan, WANG Qiong-li, HARTLEY W. Vermicompost and Gypsum amendments improve aggregate formation in bauxite residue [J]. Land Degradation and Development, 2017, 28(7): 2109–2120. DOI: 10.1002/ldr.2737.
[23] YILMAZ E. Assessment of the role of agricultural wastes in aggregate formation and their stability [J]. Journal of Environmental Management, 2014, 144(21): 93–100. DOI: 10.1016/j.jenvman.2014.05.023.
[24] COURTNEY R G, JORDAN S N, HARRINGTON T. Physico-chemical changes in bauxite residue following application of spent mushroom compost and gypsum [J]. Land Degradation and Development, 2010, 20(5): 572–581. DOI: 10.1002/ldr.926.
[25] JONES B E, HAYNES R J. Cation and anion leaching and growth of Acacia saligna in bauxite residue sand amended with residue mud, poultry manure and phosphogypsum [J]. Environmental Science and Pollution Research, 2012, 19(3): 835–846. DOI: 10.1007/s11356-011-0630-1.
[26] JALALI M, RANJBAR F. Effects of sodic water on soil sodicity and nutrient leaching in poultry and sheep manure amended soils [J]. Geoderma, 2009, 153(1): 194–204. DOI: 10.1016/j.geoderma.2009.08.004.
[27] POWER G, GRAFE M, KLAUBER C. Bauxite residue issues: I. current management, disposal and storage practices [J]. Hydrometallurgy, 2011, 108: 33–45. DOI: 10.1016/ j.hydromet.2011.02.006.
[28] SNARS K, GILKES R J. Evaluation of bauxite residues (red muds) of different origins for environmental applications [J]. Applied Clay Science, 2009, 46(1): 13–20. DOI: 10.1016/ j.clay.2009.06.014.
(Edited by YANG Hua)
中文导读
基质改良对赤泥碱性矿物转变和团聚体稳定性的影响
摘要:赤泥规模化处置关系到氧化铝工业的可持续发展,迄今尚无经济可行的处置方法,而赤泥土壤化则是一种极具发展前景的赤泥生态化处置方法。通过土柱实验,研究改良材料(CK: 对照;PG:磷石膏;PVC:磷石膏+蚯蚓菌肥)对赤泥团聚体稳定性及碱性矿物相变化的影响,结果表明:在CK,PG和PVC改良条件下1~0.25 mm团聚体颗粒比例最大,分别为39.34%、39.38%和44.51%;改良材料PG和PVC可显著降低赤泥中pH、EC、ESP、交换态Na含量和碱性矿物含量,增加赤泥交换态Ca2+含量;赤泥pH值与交换态Na+含量和钙霞石、铝酸三钙、碳酸钙的含量呈正相关关系,而与交换态Ca2+含量呈负相关;磷石膏和蚯蚓菌肥联用可明显降低赤泥碱性矿物含量、增强团聚体结构的稳定性。研究结果为赤泥碱性调控及土壤化过程团聚体稳定性调控提供科学依据。
关键词:赤泥;基质改良;碱性矿物;团聚体稳定性;赤泥土壤化
Foundation item: Projects(41701587, 41877511) supported by the National Natural Science Foundation of China
Received date: 2018-10-31; Accepted date: 2018-11-27
Corresponding author: XUE Sheng-guo, PhD, Professor; Tel: +86-13787148441; E-mail: sgxue70@hotmail.com, sgxue@csu.edu.cn; ORCID: 0000-0002-4163-9383