
Chemical kinetic studies on interfacial reaction in SCS-6 SiC/Ti matrix composites
ZHU Yan(朱 艳)1, 2, 3, YANG Yan-qing(杨延清)2, WANG Yao-yu(王尧宇)3
1. College of Environment and Chemical Engineering, Xi’an Polytechnic University, Xi’an 710048, China;
2. School of Materials, Northwestern Polytechnical University, Xi’an 710072, China;
3. Department of Chemistry, Shaanxi Key Laboratory of Physico-Inorganic Chemistry, Northwest University,Xi’an 710069, China
Received 20 June 2007; accepted 7 December 2007
Abstract: Interfacial reaction and its mechanism of SiC/Ti composite were revealed by chemical kinetic studies. A two-step dynamic model of interfacial reaction in SCS-6 SiC/Ti composites was built up, and the rate constant and the activation energy of the interfacial reactions were obtained based on the quantum chemistry calculation. The results show that the first step, in which the atomic Ti, C and Si are decomposed from Ti matrix and SiC fiber, respectively, is a rate-determined step because the activation energy of the step is much larger than that of the second one in which deferent interfacial reaction products form. The theoretically predicted result of the interfacial reaction is coincident with that of experimental observation.
Key words: quantum chemistry; SiC/Ti composites; interfacial reaction; chemical kinetics
1 Introduction
Serious interfacial reaction between SiC fiber and Ti matrix can degenerate the mechanical properties of the SiC/Ti matrix composites that are of great importance to the aero-engines[1-4]. Up to now, the investigation on the interfacial reaction of the SiC/Ti matrix composites is focused mainly on experimental observation[2,4-9]. It is found that the interfacial reaction is much different in different composite systems. For example, only TiC forms by the interfacial reaction in SiC/Ti6Al4V in which the SiC fiber has a pure C-coating on its outmost surface[5]. In SCS-6 SiC/IMI834, the interfacial reaction products are TiC+Ti5Si3, TiC and (Ti,Zr)2Si[6]. But in SCS-6 SiC/Ti-25Al-10Nb-3V-1Mo, TiC+Ti5Si3, TiC, Ti3Si, Ti3AlC and Ti5Si3 were identified in layers from fiber side to matrix side[7]. In addition, other interfacial reaction products, such as Ti3SiC2 and Ti2AlC, were also reported[5,8-9]. It seems true that the layered distribution of the reaction products and the first layer of TiC close to the fiber are the common features. On the other hand, studies on thermodynamics[10] can give us some theoretic comprehension on the interfacial reaction of the composites. In fact, chemical changes favored by thermodynamics sometimes proceed at such a low rate that they cannot come true in practice. In order to reveal the mechanism of interfacial reactions in SiC/Ti matrix composites, it is demanded not only on thermodynamic study but also on kinetic study. Unfortunately, to our knowledge, there is hardly any chemical kinetic study on the interfacial reaction of the SiC/Ti matrix composites. Therefore, in this work, the chemical kinetic study of the interfacial reaction was conducted based on the quantum chemistry calculation.
2 Quantum chemistry calculation with Gaussian’98 software
For a chemical reaction, quantum chemistry calculation[11] can give a lot of thermal dynamic and kinetic data only according to the law of quantum mechanics and small amounts of physical constant. At first, a possible reaction route should be proposed according to the knowledge from literature and experience before quantum chemistry calculating. Then the equilibrium geometric structure of the reactants, the products and the transitions can be optimized with the help of Gaussian’98 program[12]. Equilibrium geometric structure is the molecule structure with the lowest energy in the potential energy plane. During optimization, a TS keyword is needed and a frequency calculation on the optimized structure can confirm that it is a first-order saddle point. At last, running the intrinsic reaction coordinate(IRC) calculation can verify that the transition structure connects the reactants and products. That is to say, when the final energy and structure of both the reactants and products in an IRC calculation are the same as those optimized, the transition states can be verified.
3 Chemical kinetic
3.1 Kinetic model of interfacial reactions in SCS-6 SiC/Ti composites
According to the previous investigations and the structure of the SCS-6 SiC fiber with a C-coating containing very fine SiC grains inside, 16 possible reactions, as listed in Table 1, were supposed and their Gibbs function increments, ?rG, were calculated[4,13]. It can be found that atomic Ti or C or Si as the reactant appears in each interfacial reaction. In general, the reactions in which atoms take part in are the elementary reaction and the activation energies are very low[14]. Fig.1 shows a potential energy scanning on the reaction products TiC and TiSi by quantum chemistry Gaussian’ 98 software, showing that the potential curves have a minimum point when the molecule of TiC or TiSi is stable and each potential energy increases with the increase of the atom distance R. Until the molecule of TiC or TiSi is decomposed into atoms, the curves do not tend to the maximum value which is the sum of the energies of two composing atoms. It is clear that the potential energy of atom is the maximum. Based on quantum chemistry and transition state theory, a two-step kinetic model of interfacial reaction for SCS-6 SiC/Ti matrix composites is supposed. The first step is rate- determined step, in which atomic Ti, Si and C are decomposed from the matrix and the C-coating of the SiC fiber. The second step is the formation of the interfacial reaction products listed in Table 1.
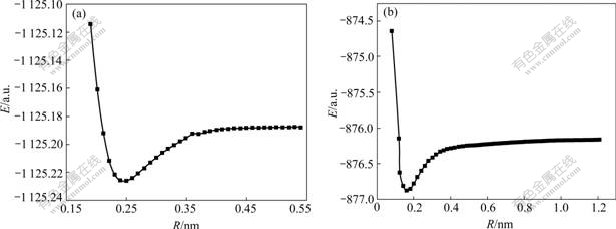
Fig.1 Potential energy scanning of TiSi (a) and Ti (b)
Table 1 Possible interfacial reactions of SCS-6 SiC/Ti matrix composites[4,13] and activation energy
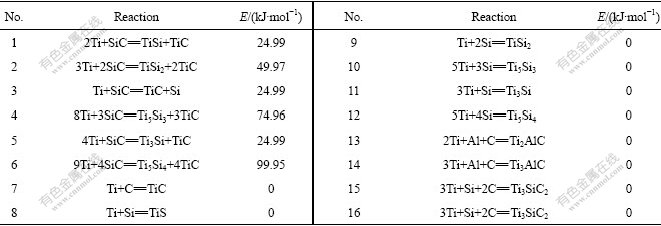
3.2 Rate-determining step of interfacial reaction in SCS-6 SiC/Ti matrix composites
At the first step, atomic Ti, C and Si are decomposed,that is
C(graphite)→C(atom) (1)
Ti(s)→Ti(atom) (2)
SiC(s)→Si(atom)+C(atom) (3)
The dissociation energies of bond are obtained by the quantum chemistry calculation. As listed in Table 2, the calculated values coincide well with the experimental ones in Ref.[15]. That is to say, the activity energies in rate-determined step of interfacial reaction in SCS-6 SiC/Ti composites are needed and the value equals its dissociation energy of bond respectively. Table 2 indicates that the bond strength of Ti—Ti, Si—C and C—C increases in turn. This result is in a close agreement with order of the melting point (the melting points of Ti, SiC and C are 1 668 ℃, 2 700 ℃[12] and 3 527 ℃, respectively). It is clear that under the condition of processing and using the SCS-6 SiC/Ti composites, the reaction Ti(s)→Ti(atom) proceeds at first, next is SiC(s)→Si(atom)+C(atom), then follows the reaction of C(graphite)→C(atom).
Table 2 Dissociation energy of bond (kJ/mol)
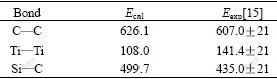
Based on transition theory, the calculation formula of rate-constant is as follows[16]:
k=k′T/h exp[-?GΘ≠/(RT)]=
k′T/hexp[-?SΘ≠/R-?HΘ≠/(RT)] (4)
where k′ is Boltzmann constant; h is Planck constant; ?SΘ≠ and ?HΘ≠ are standard activation entropy and standard activation enthalpy, respectively. ?SΘ≠, ?HΘ≠ and the activation energy ?EΘ≠ can be calculated by quantum chemistry at different temperatures, as listed in Table 3. Therefore, the rate constant of each reaction can be obtained, as listed in Table 4. The activation energy and the rate constant in Tables 3-4 further confirm dissociation order in the rate-controlled step mentioned above.
Table 3 Calculated data of ?EΘ≠, ?HΘ≠ (kJ/mol) and ?SΘ≠ (J/(mol?K))
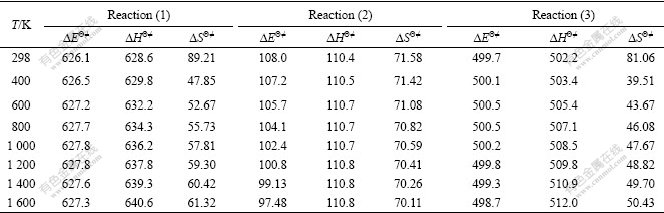
Table 4 Rate constant of each reaction (s-1)
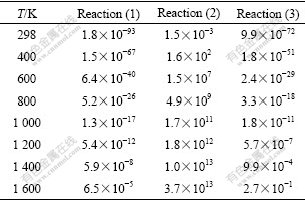
3.3 Kinetic analysis of interfacial reaction in SCS-6 SiC/Ti composites
Normally, the activation energy of a reaction in which atom participates is very small[14]. Therefore, the interfacial reactions in the second step of the SCS-6SiC/ Ti matrix composites belong to the fast ones because the atomic Ti appears in all the reactions, as listed in Table 1. Evidently, the smaller the activation energy is, the more easily the reaction proceeds. The activation energy of the interfacial Reactions 7-16 in Table 1 can be calculated by quantum chemistry calculation. And that of the Reactions 1-6 was estimated empirically.
The potential energy scanning of diatomic molecule, TiC and TiSi, were made by quantum chemistry software, Gaussian’98. As mentioned above, Fig.1 shows that the potential curves have a minimum point when the molecule of TiC or TiSi is stable, and the atomic Ti, Si and C have the highest potential energy. That is to say, the transition states of Reaction 7 and Reaction 8 are, respectively, the state of reactant. So the activation energies of the two reactions are zero. To seek transition state of the other interfacial Reactions 9-16 with multi-atomic molecule as reactive product, an optimization of the transition state structure for the reaction was conducted. Because the energy obtained by seeking transition state method is always less than the sum of reactant energies, it is deduced that the transition states of Reactions 9-16 in Table 1 are the state of reactants. So the activation energies of above eight reactions are equal respectively to zero. Moreover, based on the principle of microscopic reversibility, the reactions between atoms do not need activation energy, namely E=0[14]. The activation energy values calculated by quantum chemistry coincide well with those obtained by principle of microscopic reversibility.
On the other hand, the activation energy of Reactions 1-6 in Table 1 was obtained by empirical estimation due to the difficulty of seeking the transition state by quantum chemistry calculation. It is known that, for an exothermic reaction, the activation energy needed by an elementary reaction A+BC=AB+C, namely a atom reacts with BC molecule, approximately equals 5% bond energy of BC[14]. In other words, E≈0.05E (B—C).The results are listed in Table 1.
4 Discussion
It is shown that the Reaction 3 proceeds preferentially among Reactions 1-6 when Ti is firstly released from matrix according to the Reaction (2) in the rate-determined step. This is because of the minimum activation energy and the smallest number of the atomic Ti needed by the Reaction 3. The Si atoms released by the Reaction 3 may diffuse unceasingly from the coating to the interface of SCS-6 SiC/Ti matrix composites. That is to say, Si atoms that take part in the interfacial reaction are supplied at first by the Reaction 3 and then by the rate-determined Reaction (3). On the other hand, TiC forms at first by the Reaction 3 and soon by the Reaction 7, due to the zero activation energy, especially when the Reaction (1) in the rate-determined step is in action.
As the reactions proceed, Ti diffuses to SiC fiber, and Si and C diffuse to matrix. According to the Ti-C-Si ternary phase diagram[17], when the chemical composition at the interface reaches that of the two- phase region of TiC+Ti5Si3, the Reaction 10 occurs in addition to the Reaction 7. It is more easily to be understand that the former is more favorable than the latter due to the more negative ?rG[4,13], although the activation energy of both reactions is zero. Therefore, a thin layer of Ti5Si3 presents adjacent to TiC layer formed at first. And then a thick TiC layer presents adjacent to the Ti5Si3 because of the abundant C supply from the C-coating.
The theoretical analysis is supported by experimental observations by means of transmission electron microscopy (Philips EM430) in the interfacial reaction of SCS-6 SiC/Ti3Al and SCS-6 SiC/Ti2AlNb composites which were prepared by the technique of so-called matrix-coated-fibers and hot isostatic pressing at 920 ℃ and 192 MPa for 0.5 h. Fig.2 shows that near the C-coating of both SCS-6 SiC/Ti3Al and SCS-6 SiC/Ti2AlNb composites is a fine-grained TiC layer and then the Ti5Si3 layer. The two separately distributed layers of TiC and Ti5Si3 with fine grains were first reported by YANG et al[18]. Next is also a TiC layer but with large grains. Some silicides, such as Ti3Si and Ti5Si3, can be found close to the matrix in the figure. It is believed that the formation of the TiC layer with large grains and the silicides is controlled by diffusion[19-20], but the formation of the fine grains of TiC layer is reaction-controlled[21].
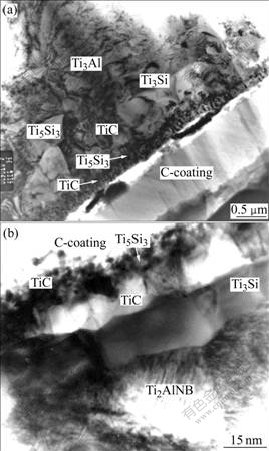
Fig.2 Morphologies of interface of as-processed SCS-6 SiC/Ti3Al (a) and SCS-6 SiC/Ti2AlNb (b)
5 Conclusions
1) A two–step dynamic model of interfacial reaction in SCS-6 SiC/Ti composites is built up. The first step in which the atomic Ti, C and Si are released is the rate-determined step because the activation energy of the step is much larger than that of the second one.
2) In the first step, Ti is decomposed first from the matrix due to the smallest activation energy and the largest rate constant for the reaction. At last, the decomposition of C from the C-coating of the fiber occurs.
3) Following the rate-determined step, the second step starts at once to form the interfacial reaction products, in which the first reaction is controlled by reaction Ti+SiC=TiC+Si, others are diffusion- controlled.
References
[1] ZHOU Y G, YANG Y Q. Progress in the study of titanium matrix composites reinforced by SiC fibers [J]. Acta Met Sinica, 2002, 38(suppl): 461-465.
[2] YANG Y Q, ZHU Y, MA Z J, CHEN Y, DUDEK H J. Study on interfacial reactions of titanium matrix composites reinforced by SCS-6 SiC fibers [J]. Acta Met Sinica, 2002, 38(suppl): 466-469.
[3] HANUSIAK W M, FIELD J L, NANSEN D S. Titanium matrix composites (TMC) status [C]// LUETJENING G, ALBRECHT J. Ti-2003 Science and Technology. Weinheim: Wiley-VCH Verlag, 2004: 2461-2469.
[4] YANG Y Q, ZHU Y, MA Z J, CHEN Y. Interfacial reaction of super α2 and Ti2AlNb composites reinforced by SCS-6 SiC fibers [C]// L?TJENING G, ALBRECHT J. Ti-2003 Science and Technology. Weinheim: Wiley-VCH Verlag, 2004: 2479-2486.
[5] YANG Y Q, MA Z J, L? X H, LI J K, CHEN Y, AI Y L. Studies on interface of SiC/Ti-6Al-4V composites [J]. Rare Met Mater Eng, 2006, 35(10): 1516-1521.
[6] DUDEK H J, BORATH R, LEUCHT R, KAYSSER W A. Transmission electron microscopy of the fibre-matrix interface in SiC-SCS-6-fibre-reinforced IMI834 alloys [J]. J Mater Sci, 1997, 32: 5355-5362.
[7] YANG Y Q, DUDEK H J, KUMPFERT J. Interfacial reaction and stability of SCS-6 SiC/Ti-25Al-10Nb-3V-1Mo composites [J]. Mater Sci Eng A, 1998, 246: 213-220.
[8] L? X H, YANG Y Q, MA Z J, LIU C X, CHEN Y, AI Y L. Kinetics and mechanism of interfacial reaction in SCS-6 SiC continuous fiber-reinforced Ti-Al intermetallic matrix composites [J]. Trans Nonferrous Met Soc China, 2006, 16(1): 77-83.
[9] MOGILEVSKY P, WERNER A, DUDEK H J. Application of diffusion barriers in composite materials [J]. Mater Sci Eng A, 1998, 242: 235-247.
[10] ZHU Y, YANG Y Q, SUN J. Calculation of activity coefficients for components in ternary Ti alloys and intermetallics as matrix of composites [J]. Trans Nonferrous Met Soc China, 2004, 14(5): 875-879.
[11] FORESMA J B. Exploring chemistry with electronic structure methods [M]. USA: Gaussian Inc, 1996: 173-180.
[12] FRISCH A, FRISCH M J. Gaussian 98 User’s Reference [M]. USA: Gaussian Inc, 1999: 17-107.
[13] ZHU Y, YANG Y Q, MA Z J, CHEN Y. Interfacial reaction and mechanism of SiC/Ti2AlNb composite [J]. Rare Met Mater Eng, 2002, 31(6): 410-414.
[14] HAN De-gang, GAO Pan-liang. The base of chemical kinetics [M]. Beijing: Beijing University Press, 1987: 132.
[15] WEAST R C. CRC handbook of chemistry and physics [M]. Florida: CRC Press, 1995: F-219.
[16] WANG Z L, ZHOU Y P, LI S L, LIU J J. Physical chemistry [M]. Beijing: Higher Education Press, 2001: 154.
[17] ZHU Y. Study on interfacial reaction of SiC fiber reinforced Ti-matrix composites [D]. Xi’an: Northwestern Polytechnical University, 2003.
[18] YANG Y Q, DUDEK H J, KUMPFERT J. Microstructure of interfacial reaction zone in SCS-6 SiC reinforced super a2 composites [J]. Mater Sci Techn, 1998,14: 1122-1126.
[19] YANG Y Q, L? X H, LUO X, MA Z J, LI J K, WEN Q, CHEN Y, Kinetics of interfacial reaction in SiC/Ti6Al4V composites [J]. Mater Sci Forum, 2007, 546/549: 1627-1632.
[20] L? X H, YANG Y Q, HUANG B, LUO X, LIU Y C. Reaction diffusion in continuous SiC fiber reinforced Ti matrix composite [J]. Trans Nonferrous Met Soc China, 2007, 17(1): 27-34.
[21] GUO Z X, BERBY B. Interfaces in Ti3Al composites reinforced with sigma SiC fibres [J]. Scripta Met Mater, 1994, 30: 89-94.
Foundation item: Project(50371069) supported by the National Natural Science Foundation of China; Project(2006E121) supported by the Natural Science Foundation of Shaanxi Province, China; Project(05JF21) supported by the Key Laboratory Foundation of Shaanxi Province, China; Project(XGJ07005) support by the Foundation of Xi’an Polytechnic University, China
Corresponding author: ZHU Yan; Tel: +86-29-82330821; E-mail: zhuyan_1963@126.com
(Edited by LI Xiang-qun)