J. Cent. South Univ. Technol. (2008) 15: 176-182
DOI: 10.1007/s11771-008-0034 -7

Fast preparation of photopolymerized monolithic columns for capillary electrochromatography
GONG Wen-jun(龚文君)1, XU Guang-ri(许光日)1, ZHANG Yi-jun(张毅军)1,
ZHANG Yu-ping(张裕平)1, CHOI Seong-ho2, LEE Kwang-pill3
(1. Henan Institute of Science and Technology, Xinxiang 453003, China;
2. Department of Chemistry, Hannam University, Daejon, South Korea;
3. Department of Chemistry, Graduate School, Kyungpook National University, Daegu702-701, South Korea)
Abstract: Photopolymerized sol-gel(PSG) columns were prepared using methacryloxypropyltrimethoxysilane as the monomer, toluene as the porogen and hydrochloric acid as the catalyst. Four different photoinitiators such as benzoin methyl ether, Irgacure 819, Irgacure 1700 and Irgacure 1800 were comparatively used in the reaction solution in the presence and absence of sodium dodecyl sulfate. The above eight solutions were respectively irradiated at 365 nm for 5-10 min in each capillary (75 mm inside diameter) to prepare the porous monolithic sol-gel column by a one-step, in situ, process. The chromatographic behavior of the eight PSG columns were comparatively studied, all of which exhibit reversed-phase character. Using these columns, several neutral compounds, namely thiourea, benzene, toluene, ethyl benzene, biphenyl and naphthalene can be separated from mixtures with a largest column efficiency of 74 470 plate/column for thiourea. Addition of sodium dodecyl sulfate in the polymerization process has a significant influence on the morphology and migration time.
Key words: electrochromatography; monolithic columns; photopolymerized sol-gel; aromatic hydrocarbons
1 Introduction
Interest in capillary electrochromatography(CEC) in recent years has been motivated by the promising capacity of CEC combining the selectivity of HPLC with the miniaturization and high efficiency power of CZE[1-3]. Monolithic columns containing a wall-supported continuous porous bed have shown a great potential for CEC due to simple preparation procedures[4-5]. Sol-gel technology can provide a versatile approach to the synthesis of inorganic polymers and organic-inorganic hybrid materials applied in HPLC, micro-HPLC and CEC[6-7]. It can take place under extraordinarily mild thermal conditions (often room temperature) and is especially important in the context of CEC column technology where reactions need to be carried out inside small diameter capillaries. Since sol-gel columns possess significant advantages over traditional packed columns and open-tubular columns, it has led to the growing interest in the field of chromatography and related technologies[8].
Methacryloxypropyltrimethoxysilane (MPTMS), which contains both methacrylate and alkoxysilane groups, was ever used to prepare a photopolymerized or thermal-polymerized sol-gel in a single-step reaction. A porous CEC sol-gel frits and columns were fabricated by using Irgacure 1800 as photoinitator[9-12]. A porous photopolymerized sol-gel (PSG) monolith was also synthesized in the separation channel of a borosilicate glass chip via UV irradiation of a mixture of MPTMS, an acid catalyst, a porogen, and a photoinitiator[13]. In Refs.[14-15] capillary pretreatment with MPTMS was carried out to form an anchor onto the silicate matrix and prevent the gel from being leached out of the capillary. The polymerization process may be initiated in principle by heat. A porous monolithic silica column was prepared by using MPTMS as the monomer and azobisisobutyronitrile(AIBN) as the thermal initiator, but this thermal polymerization process took 24 h[16]. In this work, the monoliths were quickly prepared for 5-10 min, and the effectiveness of four photoinitiators and sodium dodecyl sulfate(SDS) was comparatively investigated in the polymerization process.
2 Experimental
2.1 Apparatus
All CEC experiments were performed on a Agilent 3DCE system (Walbronn, Germany) equipped with a diode array detector. A spectronics XL-1500 UV cross-linker (NY, USA) equipped with six 15 W blacklight tubes of predominantly 365 nm wavelength was used to irradiate the reaction solutions. An AMRAY 1000B scanning electron microscope (MA, USA) was used to study the morphology of PSG. A PSG capillary was sectioned into 10 mm segments. These segments were sputtered with gold prior to SEM analysis.
2.2 Materials and chemicals
Fused-silica capillaries (75 μm inside diameter, 375 μm outside diameter) were purchased from Yongnian Ruipu Chromatogram Equipment Co. Ltd. (Hebei, China). Irgacure 1800, Irgacure 1700 and Irgacure 819 were donated by Ciba-Geigy (Tianjin, China).
Methacryloxypropyltrimethoxysilane, benzoin methyl ether, acetonitrile(ACN), ammonium acetate, thiourea, benzene, toluene, ethyl benzene, biphenyl and naphthalene were purchased from Beijing Chemical Reagent Company and Tianjin Chemical Reagent Company (Beijing, China). Distilled water was obtained from a super-purification system (Jiangsu, China). The buffers used in the experiments were prepared with various volume ratios of 50 mmol/L ammonium acetate, water, and acetonitrile (1:3:6, 1:4:5, 1:5:4). All solutions were degassed with ultra-sonication and filtered through a membrane (0.45 mm) before use. In a typical electrophoretic experiment, aromatic hydrocarbons compounds were dissolved with methanol and injected for peak identification.
2.3 Preparation of photopolymerized sol-gel(PSG) column
The purpose of capillary pretreatment is to increase the concentration of surface silanol groups. Since silanol groups on the capillary surface represent the principal binding sites for in situ created sol-gel stationary phases, higher concentration of these binding sites on the capillary surface would facilitate the formation of highly secured sol-gel stationary phases through chemical binding with the capillary inner walls. A PSG column was prepared using a previously described procedure[9-13] with some modifications such as the use of a photoreactor with different lamp intensities, the addition of SDS for obtaining homogeneous solution and the change of different photoinitiators used in the published procedure. Briefly, a monomer stock solution containing 1 500 μL MPTMS, 45 μL 1.0 mol/L hydrochloric acid and 450 μL water, was stirred at room temperature for 30 min in dark. Toluene (1 700 μL) was added to 300 μL of above monomer stock solution and stirred at room temperature for 30 min. In the comparative experiment, 100 mg SDS was added to the monomer stock solution with toluene for obtaining the homogenous phase as soon as possible. Each photoinitiator (89 mg) such as benzoin methyl ether, Irgacure 819, Irgacure 1700 or Irgacure 1800 was respectively added to the toluene mixture and stirred at room temperature for about 1 h. In the sol-gel reaction, HCl plays an important role in the hydrolysis and condensation reactions. Photopolymerization having morphologies with different permeabilities and surface areas were prepared by varying the ratio of the monomer stock solution to toluene (porogen). The porogen acted as a through-pore template and solubilizer of the silane reagent during the reaction. Here, the amounts of four different photoinitiators and HCl were kept constant at the above values. It is noted that alkoxysilane-based sol-gel precursors usually show poor solubility in water. Therefore, SDS was selected as one of the sol solution components to achieve a homogeneous system with all the sol-gel ingredients effectively dissolved together.
Eight solutions containing four photoinitiators in the presence and absence of SDS were respectively flushed through each 40 cm long capillary with a 24.5 cm stripe of the polyimide coating removed in the middle of the capillary. After the pretreated capillary was completely filled with the mixture, the capillary was sealed at both ends with rubber stoppers. The filled capillaries were irradiated in a photochemical reactor using 365 nm light with a total dose of 0.9 J/cm2 (power 3000 μW/cm2, 5 min) to form the PSG.
In general, the sol-gel reaction involves the following steps: 1) the hydrolysis of MPTMS; 2) the condensation of hydrated silica to form siloxane bonding; 3) the polymerization of linkage of additional silanol group to form the cyclic oligmers and eventually cast a silicate work. In addition, the silanol groups on surface of the silica capillary can be incorporated into the polymerization reactions and anchor the material to the capillary walls. In the former process, the rate of hydrolysis reaction is significantly faster than that of the condensation reaction, providing favorable conditions for the linear polymers. In the latter one, the condensation rate is faster than that of the hydrolysis rate, thus it is easy to form highly branched polymeric structures and uniform particles formation.
After irradiation, the capillaries were washed with methanol using a HPLC pump to remove unreacted reagents. A window was created immediately after the PSG monolith using a razor blade to remove the polyimide coating. Once fabricated, the capillary was installed in an Agilent CE cartridge without damaging the capillary. The PSG capillary was conditioned with the separation solution for approximately 5 min using a syringe and a hand-held vise. The column was further conditioned electrokinetically in the CE instrument by driving the mobile phase through the capillary at an applied voltage of 5 or 10 kV until a stable baseline was achieved.
3 Results and discussion
After eight PSG columns were prepared using four different photoinitiators in the presence and absence of SDS, a reversed-phase mechanism was observed for these PSG columns studied. Solution partitioning between the mobile and stationary phases is the only mechanism responsible for retention of the neutral analytes. The elution order of the column is similar to that of reversed-phase chromatography with a larger relative molecular mass or more hydrophobic analytes eluting later than a smaller relative molecular mass or more hydrophilic analytes. Elution of the analytes was obtained for the mixture in less than 6 min using eight PSG columns. All experiments were carried out using the same buffer with various volume ratios of 50 mmol/L ammonium acetate, water and acetonitrile (1?3?6, 1?4?5, 1?5?4). Some typical chromatograms are shown in Fig.1. Bubble formation was not a problem during the CEC experiments, for which the typical operating currents were in the range of 10-30 mA. The retention property of these monolithic columns was calculated, as shown in Table 1. For the typical monolithic column 4, the largest efficiency up to 74 470 plate/column was achieved for thiourea, a less-retained compound. Apparent variations in the migration times were observed between the monolithic columns in the presence and absence SDS. These PSG monolithic columns added SDS had a longer migration time than that in absence of SDS (Fig.1). These phenomena probably result from the form of the homogenous phase in the polymerization process, which creates a larger surface area and denser monolithic beds. The small variation of migration time for eight columns was respectively obtained with the thiourea (0.19%- 1.48% RSD), benzene (0.16%-2.06% RSD), toluene (0.27%-3.95% RSD), ethyl benzene (0.55%-3.68% RSD), biphenyl (0.05%-4.23% RSD) and naphthalene (0.09%-3.90% RSD) over a period of 5 d (n=6).
The versatility of the PSG columns 4 and 6 was further investigated for a pressure driven separation of the typical mixture, which is shown in Fig.2. Each component was separated within 10 min at an applied pressure of 1.2 MPa (the maximum limit of the instrument).
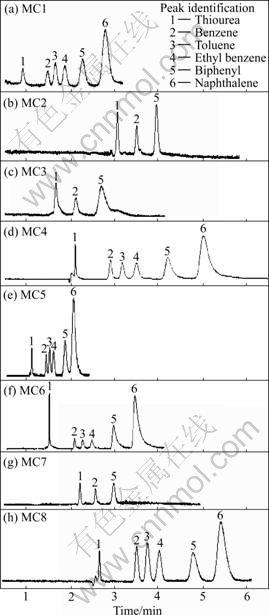
Fig.1 Electrochromatograms of separation of three to six analytes on different PSG monolith columns (Experimental conditions: Capillary length 33 cm (24.5 cm)×75 mm, 20 ℃; 50 mmol/L ammonium acetate (A), water (B), and acetonitrile (C); DAD detector): (a) MC1, injection 5 kV, 3 s, 10 kV, buffer V(A)/V(B)/V(C)=1/5/4; (b) MC2, injection 5 kV, 10 s, 20 kV, buffer V(A)/V(B)/V(C)=1/3/6; (c) MC3, injection 5 kV, 1 s; 20 kV, buffer V(A)/V(B)/V(C)=1/3/6; (d) MC4, injection 3 kV, 5 s, 20 kV, buffer V(A)/V(B)/V(C)=1/4/5; (e) MC5, injection 2 kV, 2 s, 20 kV, buffer V(A)/V(B)/V(C)=1/4/5; (f) MC6, injection 3 kV, 5 s, 30 kV, buffer V(A)/V(B)/V(C)=1/4/5; (g) MC7, injection 5kV, 3 s, 10 kV, buffer V(A)/V(B)/V(C)=1/5/4; (h) MC8, injection 5kV, 1 s, 10 kV, buffer V(A)/V(B)/V(C)= 1/4/5
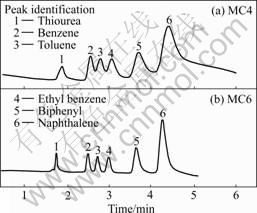
Fig.2 Chromatograms of pressure-driven separation of six analytes using PSG monolith columns 4 and 6 (Experimental conditions: Capillary length 33 cm (24.5 cm)×75 mm, 20 ℃; 50 mmol/L ammonium acetate (A), water (B), and acetonitrile (C); peak identification is the same as Fig.1): (a) MC4, injection 3 kV, 5 s, separation pressure 1.2 MPa, buffer V(A)/V(B)/V(C)=1/4/5; (b) MC6, injection 3 kV, 3 s, separation pressure 1.2 MPa, buffer V(A)/V(B)/V(C)=1/4/5
The sol-gel process is in essence the formation of a silica glass at low temperatures and atmospheric pressures. The network is achieved via the hydrolysis of an alkoxy silicate followed by condensation then polymerization. The result is a 3D and porous silica network. Characterization of PSG columns using different photoinitiators by SEM reveals an inter- connecting network of about 2 mm spherical structures through which micrometer-sized macropores (as large as 5 mm) are interspersed (Fig.3). These macropores serve as “through pores” that allow for mass transfer of the analytes from the mobile phase to the chromatographic active sites for separation. SEM analyses also reveal that covalent bonds are formed between the silica and the capillary wall during the polymerization process. Denser monolithic beds are less permeable and higher pressures
are needed to drive liquid flow through. Superficially, column permeability may be irrelevant in CEC separa- tion since EOF as the driving force in a CEC to propel the mobile phase through the column without requiring mechanical pressure[14]. However, columns with high permeability provide some significant advantages, especially in pressure-assisted CEC operation and sample injection or quick flushing of the capillary during column regeneration or equilibration[15-16]. The macroporous monolithic structures facilitate the mobile phase flow through the pores, and thereby, promote effective solute/stationary phase interaction by bringing them together. Effective solute transport mechanism operating within this monolithic structure due to mobile phase flow through the macropores together with the flat flow profile of EOF leads to high speed and separation efficiency in CEC[11].
Photopolymerization is the process of converting a liquid monomer to a solid polymer by using ultraviolet radiation. This photochemical process starts by the absorption of ultraviolet light by a photosensitive chemical compound (photoinitiator). We learn that different photo-initiators determine the speed of photopolymerization. Irgacure 1700 consists of 25% bis(2, 6-dimethoxybenzoyl)-2, 4, 4-trimethyl pentyl- phosphineoxide and 75% 2-hydroxy-2-methyl-1- phenyl-propan-1, but Irgacure 1800 consists of 25% bis(2,6-dimethoxybenzoyl)-2, 4, 4-trimethyl-pentyl phosphineoxide and 75% 1-hydroxy-cyclohexyl-phenyl- ketone. The principles of four photoinitiators in the reaction are shown in Fig.4.
The photoinitiators start the polymerization process by producing free radicals. Free radicals are highly reactive species that break up carbon-carbon double bonds (C=C bonds) in molecules in the monomer that undergoes polymerization. By destroying carbon-carbon bonds, the molecule itself becomes highly reactive and
Table 1 Experimental results on retention factor and theoretic plates for eight monolithic columns
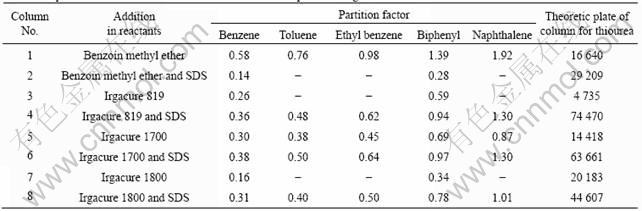
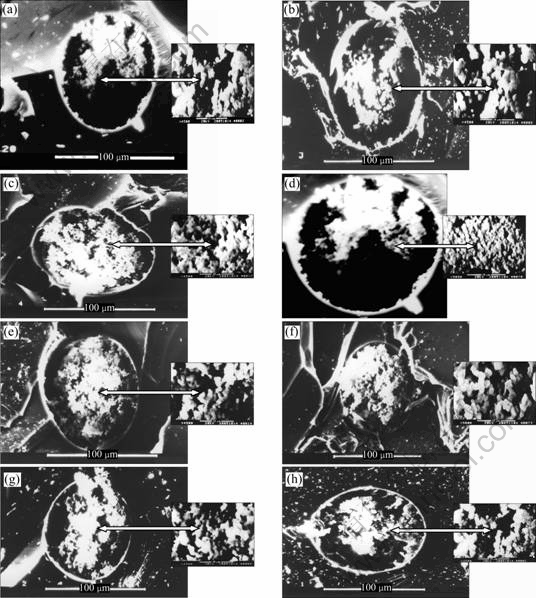
Fig.3 SEM images of monolithic columns 1-8: (a) MC1; (b) MC2; (c) MC3; (d) MC4; (e) MC5; (f) MC6; (g) MC7; (h) MC8
links itself to another highly reactive molecule. By means of forming of long macromolecules the liquid monomer changes to a solid polymer that can have totally different properties from the liquid monomer. The speed of the polymerization process is highly dependent on the number of free radicals. In this work, each mixture containing different photoinitiators with the same amount was exposed to ultraviolet radiation of 365 nm for about 5-10 min. The final polymerization did not create apparent difference. The faster the polymerization occurs and the higher the degree of conversion of the liquid monomer to a solid polymer is, here, the polymerization rate with different photoinitiators dissolved in the monomer MPTMS was not investigated specifically.
4 Conclusions
1) An easy and fast method for the preparation of monolithic columns using photopolymerized sol-gel techniques is developed in less than 5 min. It avoids the use of expensive capillaries with UV-transparent outer coatings.
2) Rapid separation of all model compounds can be obtained by the obtained monoliths prepared using different photoinitiators in less than 6 min.
3) The performance evaluations by both voltage- driven and pressure-driven separation modes show that solution partitioning between the mobile phase and stationary phases is the main mechanism responsible for retention of the hydrophobic analytes.
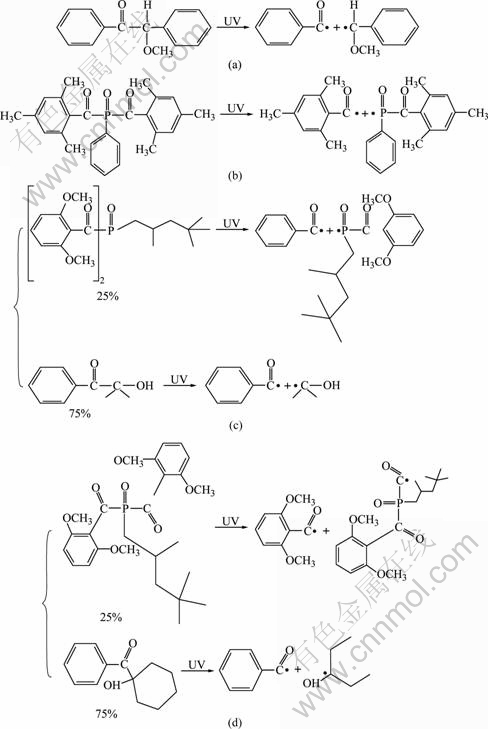
Fig.4 Schematic representation of principle of four photoinitiators in polymerization process: (a) Benzoin methyl ether; (b) Irgacure 819; (c) Irgacure 1700; (d) Irgacure 1800
References
[1] ZHANG Yu-ping, GONG Wen-jun, FAN Li-qun, LEE K P. Novel method of preparation of monolithic capillary columns for capillary electrochromatography by g-ray irradiation[J]. Chin Chem Lett, 2006, 17: 1485-1488.
[2] GONG Wen-jun, ZHANG Yi-jun, ZHANG Yu-ping. Rapid preparation of monolithic columns for capillary electro- chromatography separation[J]. Chin Chem Lett, 2006, 17: 813-815.
[3] ZHONG Fu-jin, CHEN Xiao-qing, ZHANG Shu-chao, LI Yue-ping. Organic acids and inorgranic anions in Bayer liquors by ion chromatography after solid-phase extraction[J]. Journal of Central South University of Technology, 2007, 14(1): 191-195.
[4] PREINERSTORFER B, LINDNER W, LAMMERHOFER M. Polymethacrylate-type monoliths functionalized with chiral amino phosphonic acid-derived strong cation exchange moieties for enantioselective nonaqueous capillary electrochromatography and investigation of the chemical composition of the monolithic polymer[J]. Electrophoresis, 2005, 26: 2005-2018.
[5] MOTOKAWA M, OHIRA M, MINAKUCHI H. Performance of octadecylsilylated monolithic silica capillary columns of 530 μm inner diameter in HPLC[J]. J Sep Sci, 2006, 29: 2471-2477.
[6] HENCH L L, WEST J K. The sol-gel process[J]. Chem Rev, 1990, 90: 33-72.
[7] JANCO M, XIE S, PETERSON D S, DULAY M T, QUIRINO J P, ZARE R N. Effect of porosity and surface chemistry on the characterization of synthetic polymers by HPLC using porous polymer monolithic columns[J]. J Sep Sci, 2002, 25: 909-916.
[8] GATSCHELHOFER C, MAGNESA C, PIEBER T R, BUCHMEISERB M R, SINNERA F M. Evaluation of ring-opening metathesis polymerization (ROMP)-derived supports[J]. J Chromatogr A, 2005, 1090: 81-89.
[9] DULAY M T, QUIRINO J P, ZARE R N. Bonded-phase photopolymerized sol-gel monoliths for reversed phase capillary electrochromatography[J]. J Sep Sci, 2002, 25: 3-9.
[10] KATO M, SAKAI-KATO K, TOYO’OKA T, DULAY M T, QUIRINO J P, BENNETT B D, ZARE R N. Effect of prepartatory conditions on the performance of photopolymerized sol-gel monoliths for capillary electrochromatography [J]. J Chromatogr A, 2002, 961: 45-51.
[11] DULAY M T, QUIRINO J P, BENNETT B D, ZARE R N, KATO M, ZARE R N. Photopolymerized sol-gel monoliths for capillary electrochromatography[J]. Anal Chem, 2001, 73(16): 3921-3926.
[12] KATO M, DULAY M T, BENNETT B D, ZARE R N. Photopolymerized sol-gel frits for packed column in capillary electrochromatography[J]. J Chromatogr A, 2001, 924: 187-195.
[13] MORISHIMA K, BENNETT B D, DULAY M T, ZARE R N. Toward sol-gel electrochromatographic separations on a chip[J]. J Sep Sci, 2002, 25: 1226-1230.
[14] SAKAI-KATO K, KATO M, TOYO’OKA T. On-line trypsin-encapsulated enzyme reactor by the sol-gel method integrated into capillary electrophoresis[J]. Anal Chem, 2002, 74(13): 2943- 2949.
[15] SAKAI-KATO K, KATO M, TOYO’OKA T. On-line drug-metabolism system using microsomes encapsulated in a capillary by the sol-gel method and integrated into capillary electrophoresis[J]. Anal Biochem, 2002, 38: 278-284.
[16] SHAO Hua, DENG Qi-liang, LUN Zhi-hong, YAN Cao, GAO Ru-yu. Preparation and evaluation of monolithic silica columns for capillary electrochromatography[J]. Chin J Chromatogra, 2005, 23: 243-246. (in Chinese)
(Edited by YANG Hua)
Foundation item: Project(20611140646) supported by the NSFC-KOSEF Scientific Cooperation Program; project(2006HANCET-01) supported by Program for New Century Talents of University of Henan Province; project(2005-461-140) supported by Program for Backbone Teacher in Henan Province
Received date: 2007-10-15; Accepted date: 2007-11-25
Corresponding author: ZHANG Yu-ping, Professor, PhD; Tel: +86-373-3040861; E-mail: yupzhang@hotmail.com