J. Cent. South Univ. (2016) 23: 318-324
DOI: 10.1007/s11171-017-3433-4

Dissolution kinetics of kaolin mineral in acidic media for predicting optimal condition for alum production
Folahan A. ADEKOLA, Alafara A. BABA, Sadisu GIRIGISU
Department of Industrial Chemistry, University of Ilorin, P.M.B. 1515, Ilorin-24003, Nigeria
Central South University Press and Springer-Verlag Berlin Heidelberg 2016
Abstract: The dissolution kinetics and mechanisms of reaction of Batagbon Kaolin in sulphuric and fluosilicic acids were studied. Leaching temperature, acid concentration, particle size, solid-to-liquid ratio, and stirring speed were selected as process parameters. It is observed that the dissolution rate increases with decreasing particle size and solid-to-liquid ratio, and increases with stirring speed, acid concentration, and leaching temperature. The experimental results indicate that the dissolution rate is of mixed control via hydrogen ion [H+] action, with reaction order of 0.813 and the reaction kinetics can be expressed as Kmt=[1-(1-x)1/3+y/6[(1-x)1/3+ 1-2(1-x)2/3]. The activation energy of the process is determined to be 21.6 kJ/mol. The level of the product quality is also evaluated.
Key words: kaolin; alum; sulphuric and fluosilicic acid; leaching; kinetic model; mixed control
1 Introduction
Alum, a colourless to white crystalline substance is a constituent of the mineral alunite KAl(SO4)2·2Al(OH)3 and occurs naturally as potassium aluminium sulphate (Kalunite) KA1(SO4)2·12H2O. It belongs to a class of hydrated double sulphate salts of univalent and trivalent cation and has a general formulae MISO4, MIII2(SO4)3·nH2O. Other common alums are ferric ammonium alum NH4Fe(SO2)2·12H2O, and sodium chrome alum NaCr(SO4)2·12H2O [1]. However, the term ‘alum’ is also applied to a whole series of crystallized double sulphates with the same crystal structure as the common alums, in which sodium and other univalent metals may replace the aluminium. Also, the sulphates radical may be replaced by selenate [2]. Thus, alums occur naturally in various minerals including clay, and are widely purified by crystallization from their solutions. Thus, bauxite (Al2O3) kaolin (Al2O3·2SiO2·2H2O) and alunite KAl(SO4)2·2Al(OH)3 are important minerals for alum production. Of these minerals, bauxite has been found to be richer in alumina content [3].
Due to the increasing demands for water utilization by man and for other uses, it is imperative to monitor the means of water purification from various sources. Consequently, one of the major purification processes for water is coagulation which often requires the use of a coagulant such as alum. But pure bauxite (which is of higher alumina content) is the main conventional raw material for alum production. Apparently this mineral is not available in large quantities for commercial alum production in Nigeria. Therefore, for economic consideration and energy saving, kaolin (with ≥35% Al2O3) is often used as alternative for alum production in this regard. Conventional kaolin processing for alum production requires calcinations at high temperature and pressure, and thus, it is not economically viable owing to the nature of by-product produced [4-5]. In order to avoid alum processing through intensive energy utilization, leaching with sulphuric acid in the presence of fluosilicic acid often helps in energy saving as well as reduction in the by-products produced, which if further processed, can be used for producing alumina-silica composites such as mullite [3].
Leaching is a heterogeneous reaction that takes place at the interface between a solid and liquid phase and sometimes in a gaseous phase. Thus, treatment of kaolin for alum production through leaching is becoming more popular, but apparently there are limited data in this area of study in Nigeria. The closest of this is the work reported by REGINA and ONUKWULI [6] on the dissolution of Udi clay (Anambra State) by nitric acid. Therefore, the objective of this present study is to investigate the use of sulphuric acid in the presence of fluosilicic acid for the leaching of kaolin. The effects of variables such as reaction time, acid concentration, temperatue and particle size for optimization of kinetic
conditions for alum production from the clay mineral were examined. Hence, there is the need to establish an alternative route for alum production from kaolinitic clay, rather than using bauxite which is absent in commercial quantity in Nigeria and diminishing in quantity and quality globally [7]. However, Nigerian kaolin which contains a vast content of alumina is known to have purity as high as 90% and an estimated reserve of three billion metric tons distributed in various parts of the country [8-11].
2 Experimental
2.1 Material
Kaolin sample used for this study was sourced from Batagbon, in Edu local Government Area of Kwara State, Nigeria. The elemental analysis of the kaolin was carried out by X-ray flouresence (XRF, AMPTEK PX2CR model), and the mineralogical purity was examined by X-ray diffraction (XRD, model MD 10). The crushing of the sample was done by mortar and pestle and was sieved into three particle size fractions 75-90 mm, 90-112 mm and 112-300 mm. The sized fraction 90-112 mm, unless otherwise stated was used for all experiments in this work. All reagent used were of analytical grades (BDH product) and de-ionized water was used for the preparation of all aqueous solutions.
2.2 Leaching procedure
Leaching experiments were carried out in a 250 mL glass reactor, equipped with a mechanical stirrer. For each run, the solution mixture was freshly prepared by dissolving 1 g kaolin in (3-9 mol/L) sulphuric acid solution in the presence of 35% fluosilicic acid at 60 °C for different periods of time and at a fixed stirring rate of 400 r/min [7]. Optimization of other parameters such as reaction temperature and particle size were examined by 9 mol/L sulphuric acid solution in the presence of 35% fluosilicic acid. The activation energy and other kinetic data were estimated from the Arrhenius plot [12-15]. The residue at optimal condition was characterized by scanning electron microscope (SEM) and X-ray flouresence (XRF), respectively.
3 Results and discussion
3.1 Mineralogical studies
3.1.1 Elemental analysis by XRF
The result of the elemental analysis of the Kaolin ore by XRF technique is summarized in Table 1.
From Table 1, it is evident that the major elements detected by the XRF are Ti, Fe and Al. High grade kaolin samples were found to contain Al2O3 (alumina) in the range (33%-42%) [16].
Table 1 Main composition of Kaolin sample (Mass fraction, %)
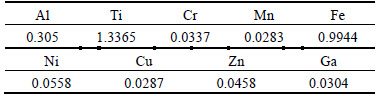
3.1.2 Mineralogical purity by XRD
Figure 1 shows the identified phases correlated by the JCPDS file numbers in the kaolin ore by X-ray diffraction.
The X-ray spectrum in Fig. 1 does complement the results of chemical analysis. It confirms the presence of aluminium silicate hydroxide Al2Si2O5(OH)4 (Kaolinite), silicon dioxide (SiO2) which may be a mixture of cristobalite, geothite (FeO(OH)), tenorite (CuO), bixbyite (Mn2O3) and titanium oxide (TiO2) in the clay mineral and occurs at low to trace levels.
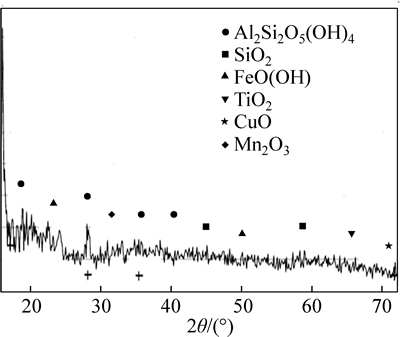
Fig. 1 X-ray spectra of Batagbon (Nigeria) kaolin sample with compounds identified
3.1.3 Morphological examination by scanning electron micrography
The morphology of the kaolin particles before and after leaching was examined by SEM-EDS and the result is presented in Fig. 2.
From Fig. 2, comparing Figs. 2(a) and (b), it could be noted that the pores within the clay after leaching have been increased, and the particle sizes have also been reduced to a very small size fractions, suggesting that the reaction has taken place possibly by diffusion through the porous layer and the surface of the kaolin. Hence, justifying the result of kinetic data suggests a mixed control reaction mechanism [7].
3.2 Leaching studies
3.2.1 Effect of H2SO4 concentration
The effect of H2SO4 concentration (3-9 mol/L) on the dissolution of kaolin sample was investigated at 60 °C using 90-112 mm particle size fraction. The fraction dissolved is plotted against leaching time for different H2SO4 concentrations as depicted in Fig. 3.
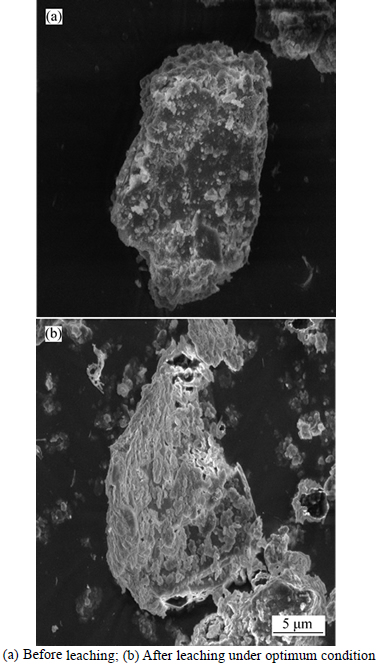
Fig 2 SEM images of kaolin sample from batagbon:
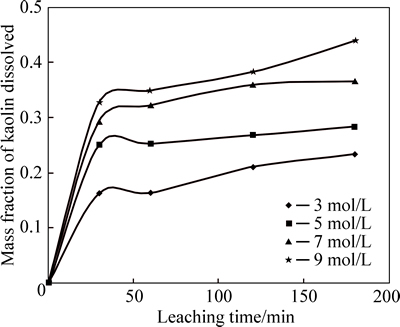
Fig. 3 Mass fraction of kaolin dissolved vs time at different acid concentrations (Experimental conditions:
=3-9 mol/L and 35% flouosilicic acid; solid to liquid ratio: 0.2 g/mL; stirring rate: 400 r/min; particle size: 90-112 mm; temperature: 55-60 °C
Figure 3 shows the effect of H2SO4 acid concentration on the extent of leaching of kaolin in the presence of 35% flousilicic acid. It can be seen that during the initial period of leaching and for all concentrations investigated, the rate of leaching was rather fast. However, the curves become almost flat within a very short period of time, after which the increase in the extent of kaolin dissolution is almost negligible. This may be due to kaolin structure collapsing at this acid concentration [17].
3.2.2 Effect of temperature
The effect of temperature on the rate of kaolin dissolution is studied over the temperature range of 30-60 °C, as shown in Fig. 4.
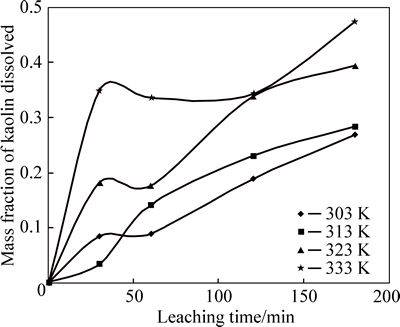
Fig. 4 Mass fraction dissolved vs time at different temperatures (Experimental conditions:
mol/L and 35% fluosilicic acid; solid to liquid ratio: 0.2 g/mL; stirring rate: 400 r/min; particle size: 90-112 mm; temperature: 55-60 °C)
Figrue 4 shows the effect of temperature on the rate of leaching of kaolin in 9 mol/L sulphuric and 35% flouosilicic acids. It can be seen that as the temperature increases, the rate of leaching also increases. This shows that an increase in the temperature of the system is directly proportional to the kaolin dissolution. This is in line with the result obtained by DEGER and MUSTAFA [18], where the dissolution rates for iron and aluminium are largely increased by temperature and the best result were recorded at 378 K. The possible reason for increased dissolution during increase in temperature might be a result of kinetic energy available for the reacting molecules participation during the ore leaching [6].
3.2.3 Effect of particle size
The effect of particle size on the rate of alumina dissolution in kaolin is studied over the range of three particle sizes of 75-90, 90-112 and 112-300 mm, as shown in Fig. 5.
As evident from Fig. 5, it is observed that the dissolution rate increases with decreasing particle size, because an increase in surface area gives rise to a higher reaction rate. Thus, the rate of kaolin dissolution ofsmallest particle size is higher. Studies by REGINA and OKECHUKWU [6] reveal that the dissolution rate is inversely proportional to the particle size. This may be attributed to larger specific surface area provided by the smaller particles for contact with the free hydrogen ions [7].
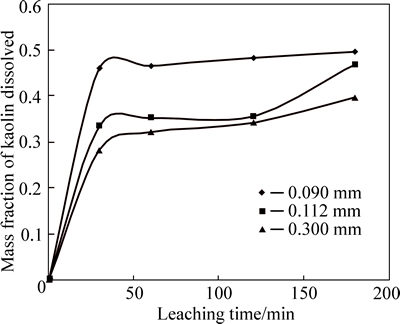
Fig. 5 Mass fraction of kaolin dissolved vs time at different particle sizes (Experimental conditions:
mol/L and 35% flouosilicic acid; solid to liquid ratio: 0.2 g/mL; stirring rate: 400 r/min; temperature: 55-60 °C)
3.3 Discussion
3.3.1 Dissolution kinetic models
The heterogeneous fluid–solid reaction systems have a number of applications in chemical and hydrometallurgical processes. Many studies have been conducted on these types of reactions and a lot of mathematical models have been proposed and developed [19]. The shrinking particle model and the shrinking core model are the most commonly used. The shrinking core model considers that the leaching process is controlled either by the diffusion of reactant through the solution boundary layer, or through a solid product layer while the shrinking particle model considers that the leaching process is controlled by the rate of the surface chemical reaction [20]. Besides, during the leaching process, dissolution rate decreases with time and it is directly dependent on the activation energy. HABASHI [21] stated that if the rate of reaction in the bulk of the solution was fast, the solution would be governed by the rate of diffusion of the ions from the surface of the solid through the boundary layer. On the other hand, if the rate of reaction is slow, it will control the overall process and the process will be chemically controlled; thus, diffusion through the boundary layer does not play any critical role [16]. It is reported that a diffusion-controlled heterogeneous process is characterized by being slightly dependent on temperature, while the chemically controlled process is strongly dependent on temperature which is attributed to linear dependency of diffusion coefficients and exponential dependency of chemical velocity constants on temperature. However, the activation energy of a diffusion-controlled process is characterized as 4-12 kJ/mol, while it is usually greater than 42 kJ/mol for chemically controlled process [13, 20, 21]. Therefore, the form of the rate equation is determined by the control regime, or the rate-limiting step which can be one of the follows:
1) Diffusion through the liquid film surrounding the solid particle;
2) Diffusion through the ash/inert solid layer;
3) Chemical reaction at the surface of the unreacted core. It is assumed that the solid particle is spherical and it reacts with the fluid isothermally. The concentration of the reacting fluid is also assumed to be constant or in excess [20]. Therefore, in order to establish the kinetic parameters and rate-controlling step for the dissolution of kaolin in sulphuric acid solution, the following shrinking core models are employed in this work. Thus, the following mathematical models with the assumption of a shrinking core/constant particle model can be written as follows [21-22]:
Diffusion control:
(1)
Surface control:
(2)
Mixed control: Kmt=[1-(1-x)1/3+y/6[(1-x)1/3+1-2(1-x)2/3] (3)
where α is the fraction reacted, KC is the kinetic constant, MB is the molecular weight of the solid, CA is the concentration of the dissolved lixiviant A in the bulk of the solution, α is the stoichiometric coefficient of the reagent in the leaching reaction, r0 is the initial radius of the solid particle, t is the reaction time, rb is the density of the solid, Kd and Kr are rate constants, and y in Eq. (3) is assumed to be 1 [23-24].
If the reaction rate of the particle is controlled by diffusion of the fluid through a layer, the model can be expressed by Eq. (1). However, if the reaction is controlled by surface reaction, then, Eq. (2) can be employed or mixed model Eq. (3), under the assumption that the dissolution reaction must conform to the first order relation [23]. The three model equations are tested for the Kaolin dissolution in acidic media and it is found that of all the three tested models, the mixed diffusion control model governed by Eq. (3) best fits the dissolution data with average correlation of about 0.958. Thus, the kinetic data of Fig. 2 are linearized with Eq. (3) as depicted in Fig. 6.
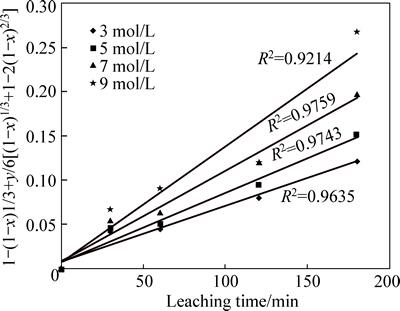
Fig. 6 Plot of [1-(1-x)1/3+y/6[(1-x)1/3+1-2(1-x)2/3] vs time at different H2SO4 concentrations in 35% flouosilicic acid (Experimental conditions:
mol/L and 35% flouosilicic acid; solid to liquid ratio: 0.04 g/mL; stirring rate: 400 r/min; particle size: 90-112m; temperature: 55-60 °C)
The slope of the plot of lnk vs ln
in Fig. 7 derived from Fig. 6 gives 0.813, and could apparently approximate to 1, indicating that the reaction order is assumed to be of first order with respect to [H+] ion concentration. This value apparently conforms to reaction mechanism, because similar results in a separate study by BABA et al [24], on the development of a combined pyro- and hydro-metallurgical route to treat spent zinc–carbon batteries follow mixed control dissolution mechanism for which the calculated reaction order is 0.74.
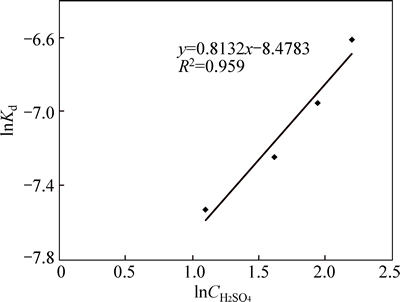
Fig. 7 Plot of Inkd vs ln
(Experimental conditions:
mol/L and 35% flouosilicic acid; solid to liquid ratio: 0.2 g/mL; stirring rate: 400 r/min; particle size: 90-112 mm; temperature 55-60 °C)
Furthermore, the dissolution data with respect to the influence of temperature on the kaolin dissolution (Fig. 4) are also linearized using Eq. (3) as presented in Fig. 8.
The data in the Table 2 are used to evaluate the Arrhenius equation of the form:
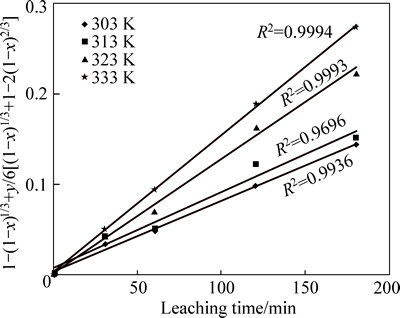
Fig. 8 Plot of 1-(1-x)1/3+y/6[(1-x)1/3+1-2(1-x)2/3] vs time at different temperatures (Experimental conditions:
9 mol/L and 35% flouosilicic acid; solid to liquid ratio: 0.2 g/mL; stirring rate: 400 r/min; particle size: 90-112 mm; temperatue: 55-60 °C)
Table 2 Values of rate constants kd with correlation coefficients, R2 at different temperatures
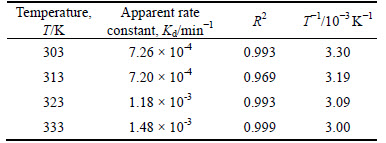
(4)
Equation (4) is further used for the estimation of activation energy for the dissolution process. From Eq. (4), A is the frequency factor, Ea is the activation energy of the reaction, R is the universal gas constant and T is the absolute temperature.
From the Arrhenius relation in Fig. 9, the calculated activation energy is 21.60 kJ/mol. To establish the reaction mechanism for the process, linearization of the kinetic curve for effect of particle size is carried out using Eq. (3). Values of the rate constants, k, are plotted against the reciprocal of the particle radii (1/ro), yielding a linear relationship with correlation of 0.829 (Fig. 10). This confirms that a surface chemical reaction is the rate controlling step during dissolution process [13, 23-24].
Also, the plot of rate constant k vs 1/ro2 is linear until a certain stage where it deviates as shown in Fig. 11. This also adds that diffusion through the product layer is not the rate determining step for the dissolution process [25].
3.3.2 Product analysis by X-ray flourescence
At optimal leaching (9 mol/L H2SO4 in 35% Flousilicic acid, temperature of 60 °C, particle size of0 (erature of 90-112 mm and stirring rate of 400 r/min for 60 min), the chemical composition of the alum produced from Batagbon kaolin sample examined by XRF to determine the level of its purity is summarized in Table 3.
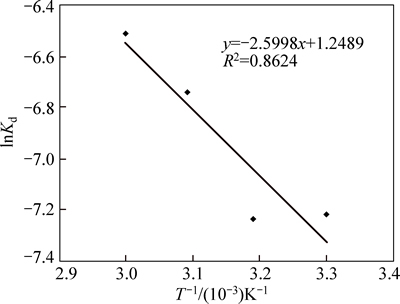
Fig. 9 Plot of In Kd vs T-1
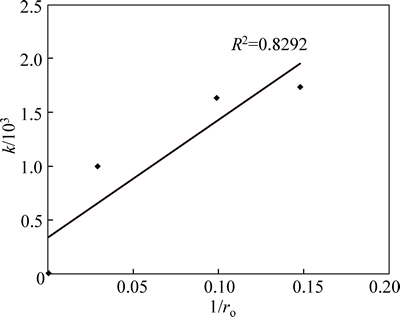
Fig. 10 Plot of k vs 1/ro at different particle sizes (Experimental conditions:
mol/L and 35% fluosilicic acid; solid to liquid ratio: 0.2 g/mL; stirring rate: 400 r/min; particle size: 90-112 mm; temperature: 55-60 °C)
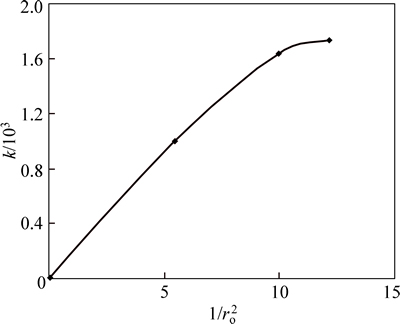
Fig. 11 Plot of k vs 1/ro2
As compared to Table 1, Table 3 reveals that some elements constituting impurities have either been totally removed or reduced to a very low concentration. It is also observed that there have been additions of other elements which are not part of the initial composition of the clay mineral which may be a result of the salt (potassium sulphate) used in crystallizing the alum. Some of the elements are removed, including Zn, Cu and Ga. Looking at these elements, they are major impurities in kaolin samples. These are heavy metals which could be harmful to the body, except Zn and Cu which are not harmful at low concentrations. However, other elements whose concentrations are drastically reduced, including Cr (from 0.0337% to 0.0029%), Ni (from 0.0558% to 0.0030%), Ti (from 1.3665% to 0.0090%) and Fe (from 0.994% to 0.0090%). Elements such as Cr, Ti and Ni are harmful to human body, but their concentrations have been reduced to less than 11, 100 and 18 times, respectively. Also, Fe (from 0.994% to 0.0090%), forming another major impurity, has been reduced to less than 100 times of its initial value. Iron impurities may give rise to brownish colour of most commercial alum; not only that, it also introduces iron into water which can lead to rusting of pipe, thereby introducing more impurities into water system. However, the alum crystal produced in this work has a very sharp white colour indicating that the iron content is very low as evident from the result of the XRF. Thus, it is an indication that the alum produced is of good quality, the content alumina removed before remains practically the same (from 30.8% to 30.35%), indicating that there is a good yield from the leaching process. Finally, the analysis of product quality examined by XRD confirms the presence of aluminium sulphate (alum) of the form: Al2(SO4)3 with JCPDS File number (26-0037) as the major compound formed.
Table 3 Elemental composition of alum produced from batagbon kaolin (mass fraction, %)
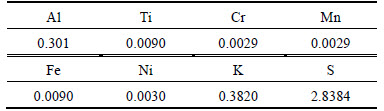
4 Conclusions
1) Kaolin dissolution is carried out by employing sulphuric and fluosilicic acid in the leaching process.
2) The concentration of fluosilicic acid is kept constant while the sulphuric acid concentration, temperature and particle size accordingly vary.
3) The presence of fluosilicic acid which is a strong oxidizing agent might be responsible for the proposed reaction mechanism where apparent activation energy and reaction order of 21.60 kJ/mol and 0.813-1 are obtained, respectively. These values support the proposed mixed control mechanism for the dissolution process.
References
[1] CONSIDINE G D. Van NOSTRAND’S Encyclopedia of Chemistry [M]. London: John Wiley and Sons, Inc., 2005: 251-255.
[2] Industrial Project Report Alum. Aluminium sulphate [EB/OL] [2011-11-28]. http// www.scribd.com/doc.
[3] ISMAIL A K. Energy saving during alum production from kaolin with coproduction of alumina-silica composites from process silica wastes [C]// Proceedings of the XI International Seminar on Mineral Processing Technology. Jamshedpur, India, 2010: 781-787.
[4] FORD K J R. Sulphuric acid and ammonium sulphate leaching of calcined clay [J]. Hydrometallurgy, 1992, 29: 109-130.
[5] PHILIPS C V, WILLS K J. Acid dissolution of alumina from waste aluminium [J]. Hydrometallurgy, 1982, 9: 15-28.
[6] REGINA O A, OKECHUKWU D O. Application of the shrinking core model to the analysis of alumina leaching from ukpor clay using nitric acid [J]. International Journal of Engineering Research & Technology (IJERT), 2012, 3(1): 1-13.
[7] GIRIGISU S. Production of alum from some local kaolin samples: leaching kinetics and process optimization [D]. Department of Chemistry, University of Ilorin, Ilorin, Nigeria. 2013.
[8] FAN A, BROWN R C, WHEELOCK T D, COOKER T A, MOMURA M, ZHUANG Y. Production of a complex coagulant from fly ash [J]. Chemical Engineering Journal, 2005, 106: 269-277.
[9] ADEREMI B O. Kinetics study on aluminium alumination spent acid recovery process [J]. Nigeria Journal of Engineering, 2002, 10(10): 27-34.
[10] BABA A A, MASOBALAJE M A, IBRAHIM A S, GIRIGISU S, ELETTA O A A, ALUKO F I, ADEKOLA F A. Bleaching of a Nigerian kaolin by oxalic acid leaching [J]. Journal of Chemical Technology and Metallurgy, 2015, 50(5): 623-630.
[11] BABA A A, ASALA O A, BALOGUN A F, AYINLA K I, BALE R B, ADEKOLA F A, ALABI A G F. Treatment of a Nigerian kaolin ore for improved industrial applications [J]. Moroccan Journal of Chemistry, 2016, 4(1): 119-127.
[12] BABA A A, ADEKOLA F A. ARODOLA O A, IBRAHIM L, GHOSH M K, SHEIK A R. Simultaneous recovery of total iron and titanium from ilmenite ore by hydrometallurgical processing [J]. Metall Mater Eng, 2012, 18(1): 67-78.
[13] BABA A A, IBRAHIM A S, BALE R B. Purification of a Nigerian talc ore by acid leaching [J]. Applied Clay, 2015, 114: 476-483.
[14] TUMAK S, CIFTILIK A, AKUIL. Factorial experiments for iron removal from kaolin by using single acid two-step leaching with sulphuric acid [J]. Hydrometallurgy, 2013, 134-135: 80-86
[15] SULTANA U K, GULSHAN A S W, KURNY. Kinetics of leaching of iron oxide in clay in oxalic acid and in hydrochloric acid solutions [J]. Materials Science and Metallurgy Engineering, 2014, 2(1): 5-10.
[16] LAWRENCE C, EDOMWONYI O, BENJAMIN O A. Comparative study of alum production from Nigeria kaolin clay [J]. Materials Science and Technology Conference and Exhibition, 2011: 16-20.
[17] AL-ZAHRAN A A, ABDUL-MAJID M H. Extraction of alumina from clays by hydrochloric acid process [J]. JKAU: Eng Sci, 2009, 20(2): 29-41.
[18] DEGER U, MUSTAFA. Dissolution kinetics of iron and aluminium from red mud in sulphuric acid solution [J]. Indian Journal of Chemical Technology, 2007, 14(3): 263-268.
[19] LAPIDUS G, de LOURDES M M. The effect of product solubility on the leaching kinetics of non-porous minerals [J]. Hydrometallurgy, 1988, 20: 49-64.
[20] AYDOGAN S, ARAS A, CANBAZOGLU M. Dissolution kinetics of sphalerite in acidic Ferric chloride leaching [J]. Chemical Engineering, 2005, 114: 67-72.
[21] HABASHI F. Principles of Extractive Metallurgy [M]. New York: Gordon & Breach, 1980: 107-153.
[22] RAY H S. Kinetics of Metallurgical Reactions [M]. New Delhi: Oxford and IBH Publishing, 1993: 23-41.
[23] G
KHAN U. Kinetics of sphalerite dissolution by sodium chlorate in hydrochloric acid [J]. Hydrometallurgy, 2005, 95: 39-43.
[24] BABA A A, ADEKOLA A F, BALE R B. Development of a combined pyro- and hydro-metallurgical route to treat spent zinc-carbon batteries [J]. Journal of Hazardous Materials, 2009, 171: 838-84.
[25] BABA A A, ADEKOLA F A. Hydrometallurgical processing of a Nigerian sphalerite in hydrochloric acid: Characterization and dissolution kinetics [J]. Hydrometallurgy, 2010, 101: 69-75.
(Edited by FANG Jing-hua)
Cite this article as: Folahan A. ADEKOLA, Alafara A. BABA, Sadisu GIRIGISU. Dissolution kinetics of kaolin mineral in acidic media for predicting optimal condition for alum production [J]. Journal of Central South University, 2017, 24(2): 318-324. DOI: 10.1007/s11171-017-3433-4.
Received date: 2015-05-04; Accepted date: 2016-03-28
Corresponding author: Folahan A. ADEKOLA, Professor, PhD; Tel: +23-48067332320; E-mail: faadekola@yahoo.fr