J. Cent. South Univ. Technol. (2008) 15: 474-478
DOI: 10.1007/s11771-008-0089-5
Aerobic oxidation behavior of α-ionone catalyzed by N-hydroxyphthalimide combined with acetylacetone cobalt(Ⅱ)
TANG Rui-ren(唐瑞仁)1, ZHOU Ya-ping(周亚平)1, GONG Nian-hua(龚年华)1, ZHAO Yu(赵 瑜)2
(1.School of Chemistry and Chemical Engineering, Central South University, Changsha 410083, China;
2. Technology Center, China Tobacco Hunan Industrial Corporation,Changsha 410014, China)
Abstract: A practical catalytic method to oxidize α-ionone with molecular oxygen using N-hydroxyphthalimide(NHPI) combined with acetylacetone cobatt(Ⅱ) (Co(acac)2) was developed, and the probable catalytic mechanism was proposed. The influences of the reaction conditions on conversion of α-ionone and the selectivity of the major product (5-keto-α-ionone) were investigated, and the technical parameters for 5-keto-α-ionone were optimized. The results show that the primary product is 5-keto-α-ionone, and by-products include epoxy-α-ionone, as well as rearrangement products 4-keto-β-ionone and epoxy-β-ionone, which are characterized by infrared spectra, proton nuclear magnetic resonance spectra, mass spectra and elemental analysis. The selectivity of 5-keto-α-ionone and the conversion of α-ionone are 55.0% and 97.0%, respectively, when 30%(molar fraction) NHPI, 1.0%(molar fraction) Co(acac)2 and no solvent are employed under O2 pressure of 1.0 MPa and the reaction temperature of 65 ℃ for 11 h. The procedure shows good reproducibility in the parallel experiments.
Key words: catalytic oxidation; α-ionone; 5-keto-α-ionone; N-hydroxyphthalimide
1 Introduction
During the last decade, much attention has been paid to N-hydroxyphthalimide(NHPI) and its analogues, which are known to be effective carbon radical catalysts. These catalysts combined with transition metal ions, such as Co2+, Co3+, Mn2+, V4+, Cu2+, Cu+ or other additives, have been reported to be highly efficient to oxidate organic compounds containing sufficiently reactive C—H bonds, such as alkanes, alkylbenzenes, alcohols, aromatic compounds into oxygen-containing compounds by using O2 as oxidant under mild conditions[1-4]. ISHII and SAKAGUCHI[5] have achieved aerobic oxidation of alkanes to a series of oxygen- containing compounds for the first time, such as alcohols, ketones by using NHPI as the radical catalyst. IWAHAMA et al[6] used NHPI/cobalt acetate/ hexacarbonyl molybdenum system to catalytically epoxidize olefins carbon double bonds effectively, such as oct-1-one and oct-2-ene at p(O2)=0.1 MPa and 60 ℃.
α-ionone is a raw material for preparation of rare flavors and their oxidative products. Epoxy-α-ionone and 5-keto-α-ionone, are the key intermediates for the synthesis of various naturally hydroxylated derivatives of ionone and oxidized carotenoids, such as γ-ionone, isozeaxanthine, diepoxy-β, β-carotene[7-9]. Although many methods on the oxidation of ionone to epoxy-ionone or oxo-ionone have been reported, there are many problems concerning low yield, harsh condition, and serious pollution[10-11].
For the purposes of developing a new method to oxidate α-ionone to epoxy-α-ionone and 5-keto-α-ionone based on low cost, “green” environmental protection, and high conversion, the NHPI/acetylacetone cobalt(Ⅱ) (Co(acac)2) catalyst system was applied to aerobic oxidation of α-ionone in this work. And the oxidative products, reaction mechanism and the technical parameters including reaction time, temperature, pressure of O2, the amounts of NHPI and Co(acac)2 were investigated.
2 Experimental
2.1 Reagents and instruments
All reagents were purchased from commercial source and used without further treatment.
Gas chromatography(GC) analysis was performed using a 0.2 mm×30 m (inner diameter×length) capillary column (HP-Ultra2), equipped with a flame ionization detector. Hydrogen was determined using Vario-EL-Ⅲ elemental analyzer(EA). Infrared spectra (IR) were recorded using KBr press plates on a VATAR-360-FT FTIR spectrometer. Proton nuclear magnetic resonance (1H NMR) spectra were obtained on an INOVA-400MHz NMR instrument using CD3Cl as solvent and tetramethylsilane(TMS) as the internal standard. Gas chromatography mass spectra were recorded on an AGILENT 6890-5973 gas chromatography-mass spectrometer(GC-MS).
2.2 General procedure
Reactions were carried out in 70 mL stainless steel autoclave with a magnetic stirrer. The general procedure was as follows: 5.0 g (0.026 mol) α-ionone, a definite amount of NHPI and Co(acac)2 were put into the autoclave, and air in the autoclave was replaced 3 times by O2. The reaction mixture was stirred at a defined temperature and O2 pressure. After reaction for 10 h, the products were qualitatively analyzed by GC-MS. The yields of products were calculated from the areas of GC peak based on the internal standard technique.
3 Results and discussion
3.1 Analysis and characterization of products
The oxidation of α-ionone (compound 1) with molecular O2 (1.0 MPa) in the presence of catalysts 30% NHPI and 1.0% Co(acac)2 under solvent-free condition at 65 ℃ for 11 h, gives 5-keto-α-ionone (compound 2) in 55.0% selectivity as the major product, epoxy-α-ionone (compound 3) in 15.0% selectivity, together with 4-keto- β-ionone (compound 4) in 20.0% selectivity, and a small amount of epoxy-β-ionone (compound 5) (see Fig.1), and the conversion of α-ionone is 97.0%. No epoxy products with branch chain are observed. The pure products are obtained by column chromatography on a silica gel eluted with 4?1 (volume ratio) petroleum-ethyl acetate. The gas chromatography spectrum of the oxidative products is shown in Fig.2.
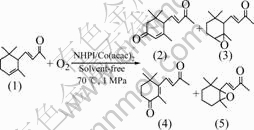
Fig.1 Aerobic oxidation process of α-ionone catalyzed by NHPI/Co(acac)2
The oxidative products were characterized by FTIR, 1H NMR, MS and EA. The data are shown as follows.
5-Keto-α-ionone: m.p. 70-72 ℃; MS m/z: 206[M+], 108[M+—C4H7, —C2H3O]; FTIR: 1 680 cm-1; 1H NMR δ: 1.09-1.11 (s, 6H, 2H3 C—C), 2.15—2.37 (m, 2H, J=16.8 Hz, 2H—C), 5.99 (1H, s, 1H—C), 1.91 (s, 3H, 3H—C), 2.73 (m, 1H, J=9.6 Hz, 1H—C), 6.69 (m, 1H, J=9.6 Hz, 1H—C), 6.20 (m, 1H, J=15.6 Hz, 1H—C),2.29 (s, 3H, 3H—C). Anal. Caled. for C13H18O2: C 75.58%, H 9.62%; Found: C 75.69%, H 9.80%.
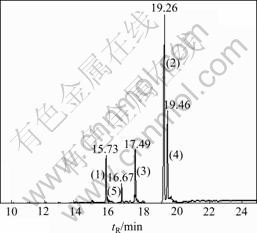
Fig.2 GC spectrum of oxidative products
Epoxy-α-ionone: MS m/z: 208[M+], 109[M+— C3H5CO, —O, —CH3] ; 1H MNR δ: 0.96 (s, 6H, 2H3 C—C), 1.30 (s, 3H, 3H—C), 7.13-7.15 (m, 1H, J=16 Hz, 1H—C), 6.33-6.39 (d, 1H, J=16 Hz, 1H—C), 2.30 (s, 3H, 3H—C).
4-keto-β-ionone[10]: m.p. 50-52 ℃; MS m/z: 206[M+], 163[M+—CH3CO]; FTIR: 1 663 cm-1; 1H- NMR δ: 1.14 (s, 6H, 2H3 C—C), 1.80-1.83 (m, 2H, 2H—C), 2.49 (m, 2H, 2H—C), 1.68 (s, 3H, 3H—C), 7.26-7.30 (m, 1H, J=16 Hz, 1H—C), 6.11-6.15 (m, 1H, J=16 Hz, 1H—C), 2.32 (s, 3H, 3H—C). Anal. Caled. for C13H18O2: C 75.25 %, H 9.66%; Found: C 75.69%, H 9.80%.
Epoxy-β-ionone: MS m/z: 208[M+], 123[M+— C3H5CO, —O] ; 1H MNR δ: 0.94 (s, 6H, 2H3C—C), 1.15 (s, 3H, 3H—C), 7.01-7.05 (m, 1H, J=16 Hz, 1H—C), 6.27-6.31 (d, 1H, J=16 Hz, 1H—C), 2.28 (s, 3H, 3H—C).
The results show that compound (2) is the major product with high selectivity in this NHPI/Co(acac)2 system, which is allylic oxidation product on double bond in the ring, while compound (3) is epoxidation product, and both of them are from direct oxidation of compound (1); compounds (4) and (5) are oxidation products formed from rearrangement of compound (1), and the process can be interpreted by Fig.3.
3.2 Optimization of reaction conditions
In order to improve the conversion of α-ionone(Xα) and the selectivity of the major product 5-keto-α-ionone (S2), the effects of reaction time, temperature, O2 pressure, the amounts of NHPI and Co(acac)2 on Xα and S2 were investigated.
3.2.1 Influence of reaction time
The time course profile is shown in Fig.4, which illustrates that Xα and S2 increase first with the reaction time, and they reach up to highest value (97.0% and 55.0%, respectively) after 11 h. After 12 h, S2 decreases sharply and a few new unknown species are observed in the GC-MS spectrum during the experiment, which indicates the further oxidation products coming from 5-keto-α-ionone. Therefore, the optimum reaction time for 5-keto-α-ionone is 11 h.
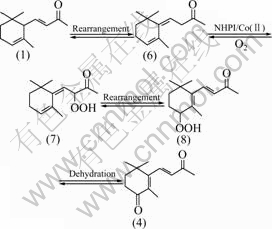
Fig.3 Formation process of 4-keto-β-ionone from α-ionone
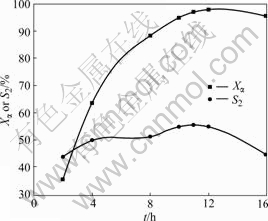
Fig.4 Influence of time on oxidation reaction of α-ionone with O2 (1.0 MPa) in the presence of 25% NHPI and 1.0% Co(Ⅱ) at 65 ℃
3.2.2 Influence of reaction temperature
The influence of reaction temperature on oxidation is shown in Fig.5. After an initial slow increase for Xα and S2, an optimum temperature is obtained at about 65℃, followed by an obvious drop after 80 ℃. Xα almost reaches a maximum at 65 ℃, and there is no conspicuous change from 65 to 80 ℃, which means that the reaction reaches a thermodynamic equilibrium at low temperature. However, Xα and S2 decrease when temperature increases (>80 ℃). The same result was reported by ZHUO et al[12]. The possible reasons are as follows: firstly, the raise of temperature, considered from the aspect of thermodynamics, results in the decrease of equilibrium conversion for its strong exothermic effect. Secondly, partial pressures of gas reactant and product H2O increase, while oxygen partial pressure and oxygen solubility in liquid-state reactant decrease, making the conversion reduce at high temperature. Thirdly, NHPI decomposes to inert phthalimide and phthalic anhydride which have no catalytic activities for the reaction process[13]. Analysis of GC-MS data shows that the decomposition of NHPI is accelerated with the raise of temperature. Finally, advanced oxidation of 5-keto-α- ionone occurs, in which part of NHPI is consumed and the effective utility of NHPI is reduced. In conclusion, the temperature of 65 ℃ is the optimum to oxidate α-ionone to 5-keto- α-ionone.
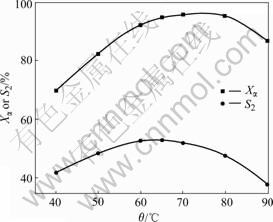
Fig.5 Influence of temperature on oxidation reaction of α-ionone with O2 (1.0 MPa) in the presence of 30% NHPI and 1.0% Co(Ⅱ) for 11 h
3.2.3 Influence of O2 pressure
The influence of O2 pressure is similar to that of temperature. The induction periods of reactions performed under high pressure can be greatly shortened. From Fig.6, it can be seen that Xα increases remarkably and S2 remains constant with the increase of O2 pressure before 1.0 MPa, while Xα and S2 reduce obviously above 1.5 MPa. Therefore, the suitable O2 pressure is selected as 1.0 MPa. Mass-transfer velocity between liquid and gas phase is accelerated, and O2 solubility in liquid phase is improved with O2 pressure enhancement, which makes conversion and selectivity increase when O2 pressure changes from 0.1 to 1.0 MPa. However, they decrease by further oxidation and NHPI decomposing discussed above when O2 pressure is higher than 1.5 MPa. This result does not agree with that of β-ionone in Ref.[14].
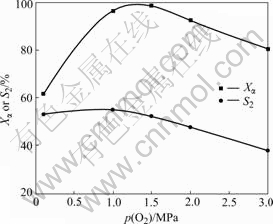
Fig.6 Influence of O2 pressure on oxidation reaction of α-ionone with O2 in the presence of 30% NHPI and 1.0% Co(Ⅱ) at 65 ℃ for 11 h
3.2.4 Influence of amount of NHPI on oxidation
Reactions are difficult to carry out when NHPI or Co(acac)2 is employed independently (Figs.7 and 8). Both of Xα and S2 increase with increasing the amount of NHPI when the molar fraction of NHPI to α-ionone is in the range of 0-30%, while there are inconspicuous changes when it is more than 30% (Fig.7). So the molar fraction of NHPI to α-ionone of 30% is the optimum. Labile dioxygen complex, such as superoxocobalt (Ⅲ) or peroxocobalt(Ⅲ), is formed by the complexation of Co(Ⅱ), with O2, and such cobalt-oxygen species accelerates the generation of phthalimide-N-oxyl (PINO) radical (Fig.9) which is the key intermediate during the oxidation[15]. Not enough PINO from NHPI can be offered when small amount of NPHI is added, thus making little influence on oxidation for excessive NHPI.
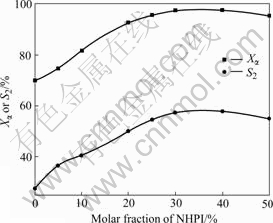
Fig.7 Influence of amount of NHPI on oxidation reaction of α-ionone with O2 (1.0 MPa) in the presence of 1.0% Co(Ⅱ) at 65 ℃ for 11 h
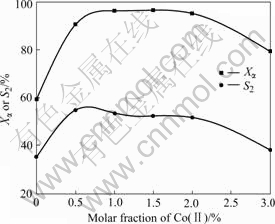
Fig.8 Influence of amount of Co(Ⅱ) on oxidation reaction of α-ionone with O2 (1.0 MPa) in the presence of 30% NHPI at 65 ℃ for 11 h
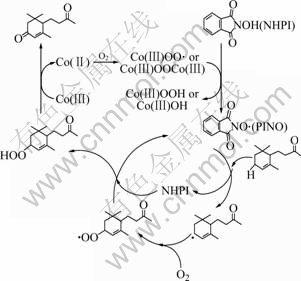
Fig.9 Mechanism for aerobic oxidation of α-ionone catalyzed by NHPI combined with Co(acac)2
3.2.5 Influence of amount of Co(acac)2 on oxidation
Experiments for various amount of Co(acac)2 were made under the conditions determined by experiments above. The results are displayed in Fig.8. Dramatic changes take place when a little Co(Ⅱ) is added. Both of Xα and S2 grow sharply first, and reach the maximum value when the molar fraction of Co(acac)2 to α-ionone are 1.0% and 0.5%, respectively, and almost keep invariable when the molar fraction of Co(acac)2 to α-ionone is not higher than 2.0%, then followed by an obvious decrease when it is higher than 2.0%. Meanwhile, deep oxidative products were observed on GC-MS. Considered from all aspects, 1.0% Co(acac)2 should be employed.
3.2.6 Repeated experiments
To conclude, both conversion of α-ionone and selectivity of 5-keto-α-ionone reach the maximum values of 97.0% and 55.0%, respectively, when 30% NHPI, 1.0% Co(acac)2 and no solvent are employed, under 1.0 MPa O2 pressure at 65 ℃ for 11 h. Parallel experiments under these conditions were repeated 5 times to examine the reproducibility in the parallel experiments, and the results indicate a good reproducibility.
4 Mechanism
Since oxygen molecule is in triplet state and α-ionone is in singlet state, oxidation reaction of α-ionone using O2 as oxidant and NHPI combined with Co(acac)2 as catalysts proceed according to radical mechanism[16]. A plausible reaction pathway for aerobic oxidation of α-ionone catalyzed by NHPI/Co(acac)2 system is displayed in Fig.9, which indicates that a labile dioxygen complex is formed by the complexation of Co(Ⅱ) with O2. The in-situ generation of PINO from NHPI by the action of the Co(Ⅲ)-oxygen complex formation is a key step in the present oxidation. The next step involves the abstraction of hydrogen atom from α-ionone by PINO to form α-ionone radicals. Synchronously, PINO becomes NHPI again during this step. Trapping the resulting α-ionone radical by dioxygen provides peroxy radical, which is eventually converted into oxygenated product through ionone hydroperoxide, synchronously, Co(Ⅲ) is reduced into Co(Ⅱ)[17].
5 Conclusions
1) The catalytic oxidation of α-ionone with molecular oxygen in the presence of NHPI/Co(acac)2 system under mild conditions gives 5-keto-α-ionone, epoxy-α-ionone, as well as rearrangement products of 4-keto-β-ionone and epoxy-β-ionone; 5-keto-α-ionone is the major product.
2) The technical parameters, including reaction time, temperature, O2 pressure and the amounts of NHPI and Co(acac)2 are optimized to improve the selectivity of 5-keto-α-ionone, and α-ionone can be converted into 5-keto-α-ionone with the selectivity of 55.0%, conversion of 97.0%, when 30% NHPI, 1.0% Co(acac)2 and no solvent are employed under 1.0 MPa O2 pressure at 65 ℃ for 11 h.
References
[1] PUNNIYAMURTHY T, VELUSAMY S, IQBAL J. Recent advances in transition metal catalyzed oxidation of organic substrates with molecular oxygen [J]. Chemical Reviews, 2005, 105(6): 2329-2363.
[2] RAJABI F, KARIMI B. Efficient aerobic oxidation of alcohols using a novel combination N-hydroxyphthalimide(NHPI) and a recyclable heterogeneous cobalt complex [J]. Journal of Molecular Catalysis A: Chemical, 2005, 232(1/2): 95-99.
[3] KOGUCHI S, KITAZUME T. Synthetic utilities of ionic liquid- supported NHPI complex [J]. Tetrahedron Lett, 2006, 47(16): 2797-2801.
[4] COSERI S, MENDENHALL G D, INGOLD K U. Mechanisms of reaction of aminoxyl (nitroxide), iminoxyl, and imdoxyl radicals with alkenes and evidence that in the presence of lead tetraacetate, N-Hydroxyphthalimide reacts with alkenes by both radical and nonradical mechanisms [J]. Journal of Organic Chemistry, 2005, 70(12): 4629-4636.
[5] ISHII Y, SAKAGUCHI S. A new strategy for alkane oxidation with O2 using N-hydroxyphthalimide(NHPI) as a radical catalyst [J]. Catalysis Surveys from Japan, 1999, 3(1): 27-35.
[6] IWAHAMA T, HATTA G, SAKAGUCHI S, ISHII Y. Epoxidation of alkenes using alkyl hydroperoxides generated in situ by catalytic autoxidation of hydrocarbons with dioxygen [J]. Chemical Communications, 2000(2): 163-164.
[7] JIANG Si-cui, WU Yang, LI Jun, LI? Zhi-ping, LIU Hui. Synthesis of rare fragrance precursor used in tobacco [J]. Fragrance Flavor Cosmetic, 2003, 5(5): 5-6. (in Chinese)
[8] ALEU J, BRENNA E, FUGANTI C, SERRA S. Lipase-mediated synthesis of the enantiomeric forms of 4,5-epoxy-4,5-dihydro-α- ionone and 5, 6-epoxy-5, 6-dihydro-β-ionone: A new direct access to enantiopure (R)-and (S)-α-ionone[J]. Journal of the Chemical Society, Perkin Transactions. 1: Organic and Bio-Organic Chemistry, 1999, 999(3): 271-278.
[9] LIU J, COLMENARES L U, LIU R S M. Fluorinated astaxanthins [J]. Tetrahedron Lett, 1997, 38(49): 8495-8498.
[10] LUO Yi-ming, LIU Chang-hui, TANG Rui-ren, YANG Hua-wu. Synthesis of 4-oxo-β-ionone by oxidation of sodium chlorate [J]. Journal of Central South University: Science and Technology, 2006, 37(3): 521-526. (in Chinese)
[11] PENG Qian-rong, YANG Min, XIE Ru-gang, SONG Guang-fu, LIU Zhong-xiang, WANG Dong-shan, CAI Yuan-qing. A synthesis method of oxo-α-ionone, oxo-β-ionone and their ether and ester derivatives by one step. China: 1817842A [P]. 2006-08-16. (in Chinese)
[12] ZHUO Guang-lan, ZHAO Wei-juan, JIANG Xuan-zhen. A novel catalyst system for the oxidation of toluene to benzoic acid [J]. Chinese Journal of Organic Chemistry, 2004, 24(8): 962-965. (in Chinese)
[13] HIRAI N, SAWATARI N, NAKAMURA N, SAKAGUCHI S, ISHII Y. Oxidation of substituted toluenes with molecular oxygen in the presence of N, N′, N″-trihydroxyisocyanuric acid as a key catalyst [J]. Journal of Organic Chemistry, 2003, 68(17): 6587-6590.
[14] TANG Rui-ren, LIU Chang-hui, LUO Yi-ming, GUO Can-cheng. Studies on the catalytic oxidation of β-ionone to 4-oxo-β-ionone [J]. Journal of Applied Chemistry, 2006, 23(7): 718-723. (in Chinese)
[15] SHELDON R A, ARENDS I W C E. Organocatalytic oxidations mediated by nitroxyl radicals [J]. Advanced Synthesis and Catalysis, 2004, 346(9/10): 1051-1071.
[16] FAN Qian, LI Yao-zhong, CHENG Pu-ming, HU Jia-yuan, LI Xian-jun. Studies on allylic oxidation in cyclohexene [J]. Chemical Research and Application, 2001, 13(5): 557-559. (in Chinese)
[17] ISHII Y, SAKAGUCHI S. Recent progress in aerobic oxidation of hydrocarbons by N-hydroxyimides [J]. Catalysis Today, 2006, 117(1/3): 105-113.
Foundation item: Project(50573019) supported by the National Natural Science Foundation of China
Received date: 2007-11-22; Accepted date: 2008-03-07
Corresponding author: TANG Rui-ren, Professor; Tel: +86-731-8836961; E-mail: trr@mail.csu.edu.cn
(Edited by CHEN Wei-ping)