
Binding tendency with oligonucleotides and cell toxicity of cetyltrimethyl ammonium bromide-coated single-walled carbon nanotubes
YAN Xue-bin1, 2, GU Yong-hong2, HUANG Dong2, GAN Li2, WU Li-xiang3,
HUANG Li-hua2, CHEN Zhe-dong1, HUANG Su-ping1, ZHOU Ke-chao1
1. State Key Laboratory for Powder Metallurgy, Central South University, Changsha 410083, China;
2. The Third Xiangya Hospital, Central South University, Changsha 410013, China;
3. Xiangya Medical School, Central South University, Changsha 410013, China
Received 29 July 2010; accepted 19 April 2011
Abstract: Functionalized carbon nanotubes (CNTs) were made for the delivery of genes and drugs and CNT-based biosensors. The basis of CNTs is for binding with biomolecules in biomedical applications. The binding tendency with small interfering RNA oligonucleotides and cytotoxicity of cetyltrimethyl ammonium bromide (CTAB)-coated single-walled carbon nanotubes (SWNTs) were studied. The field emission scanning electron microscopy and transmission electron microscopy results show that a SWNT suspension in CTAB solution was well-dispersed and stable. CTAB is the cross-linker between SWNTs and oligonucleotides. The CTAB-coated SWNTs have less cytotoxicity to human umbilical vein endothelial cells than single SWNTs and the cytotoxicity of CTAB-coated SWNTs depended on the concentration of CTAB-coated SWNTs.
Key words: single-walled carbon nanotubes; cetyltrimethyl ammonium bromide; oligonucleotides; cytotoxicity
1 Introduction
Single-walled carbon nanotubes (SWNTs) are composed of a single graphene sheet rolled into a tubular shape with unique thermal, physical, optical, and electrical properties. Since the discovery of SWNTs, they have been extensively studied for biomedical applications[1] and functionalized to be the transporters of proteins[2-3], drugs[4-5], and DNA[6-8][17] N.W.S. Kam, Z. Liu and H.J. Dai, Angew. Chem. 45 (2006), pp. 577–581. Full Text via CrossRef | View Record in Scopus | Cited By in Scopus (178). Small molecules like drugs can be covalently conjugated to the side walls of SWNTs with disulfide bonds and targeting modules. Various proteins such as bovine serum albumin, protein A, cytochrome C, and human immunoglobin G have also been adsorbed spontaneously on the sidewalls of acid-oxidized SWNTs due to hydrophobic interactions[3]. Single stranded DNAs consisting of tens of bases can wrap around SWNTs to form tight helices[9]. Chemically functionalized SWNTs with hexamethylenediamine (HMDA) and poly (diallyldimethylammonium) chloride (PDDA) can bind with negatively charged small interfering RNAs by electrostatic interactions[10].
Small interfering RNA (siRNA) is a class of oligonucleotides with double stranded RNA, 20-25 nucleotides in length, which interferes with the expression of a specific gene. Many functioned SWNTs have been developed to deliver siRNA into target cells. It is essential to understand the way of siRNA oligonucleotides binding to the surface of SWNTs to set up a CNTs delivery system. Of course, many potential biological applications of SWNTs require their dispersion in aqueous conditions. Recent developments have facilitated their applicability through strategies for linking chemical moieties on nanotubes. Surfactants, polymers, and biopolymers are mainly used in the noncovalent dispersion process of CNTs. Surfactants can enhance the stability of CNTs in water through their absorption on the surface of CNTs. Sodium dodecyl sulfate (SDS)[11], sodium dodecylbenzene sulfonate (SDBS)[12], cetyltrimethyl ammonium bromide (CTAB)[13], and Triton-X series[14] have been shown to disperse CNTs effectively. Phospholipids with PEG chains (PL-PEG) terminated with an amine group or with a methyl group have been used by KAM et al[15] to make stable aqueous suspensions of SWNTs for siRNA delivery. However, a sulfosuccinimidyl 6-(3’-[2- pyridyldithio] propionamido) hexanoate (sulfo-LC-SPDP) cross-linker and thiol-containing siRNA were necessary to afford binding between SWNTs and siRNA. Here, we present a novel complex composed of CTAB-coated SWNTs to test the binding efficacy with siRNA oligonucleotides and cytotoxicity to cultured human umbilical vein endothelial cells.
2 Experimental
The SWNTs used in this study had an outer diameter of 1-2 nm, a length of 1-3 ?m, a surface area of more than 380 m2/g, which were purchased from Chengdu Organic Chemical Co. Ltd. of the Chinese Academy of Sciences. CTAB was donated by the State Key Laboratory for Powder Metallurgy of Central South University, China. Poly(ethylene glycol) (relative molecule mass of PEG of 2000) terminated with an amine group (PL-PEG-NH2, Avanti Polar Lipids, U.S.A.) was used as another surfactant to enable dispersion of SWNTs for control experiment to test the binding tendency of PL-PEG with siRNA. For the binding experiments of SWNTs with oligonucleotides, synthetic siRNA from Shanghai Genepharma Co. Ltd. were used. The siRNA sequences used are as follows:
Sense strand
5’ AGCUCGUUCCCAAAAGAGCUU 3’
Antisense strand
3’ UUUCGAGCAAGGGUUUUCUCG 5’
CTAB powder was dissolved in deionized water at a concentration of 0.3 mg/mL. Then, SWNTs were sonicated for 30 min at a concentration of 0.1 mg/mL in the above CTAB solution. The dispersion and morphous properties of SWNTs in CTAB solutions were investigated by field emission scanning electron microscopy and transmission electron microscopy. In addition, the surface chemistry of SWNTs was investigated by measuring the zeta potential using a Zetasizer Nano ZS90 (Malvern Instruments, Worcestershire, UK).
The siRNA oligonucleotides were stored at -20 °C and were diluted in nuclease-free water (0.3 ?g/?L) for the binding experiments. The SWNT suspensions were blended with diluted siRNA. The mixture was vortexed gently, incubated at room temperature for 30 min, and subsequently centrifuged at 16 000g for 10 min. In order to evaluate the efficacy of SWNT binding with siRNA, the clear supernatants of each sample and the sediments of some samples were collected to test for the existence of siRNA by gel electrophoresis.
The gel electrophoresis patterns of siRNA in 1.5% agarose gels under 110 V for 10 min were manifested as sharply defined bands stained by GoldView (Shanghai Suolaibao Bio-technology Co. Ltd.). The nucleic acid samples tested were: undiluted siRNA, siRNA in nuclease-free water, siRNA in SWNTs suspended in aqueous solution, siRNA in CTAB solution, siRNA in PL-PEG solution, siRNA in CTAB coated SWNTs at different concentrations, and siRNA in PL-PEG coated SWNTs at different concentrations. All siRNA mixtures, except for undiluted siRNA, were centrifuged at 16 000g for 10 min before being loaded onto the gel.
Human umbilical vein endothelial cells were cultured for cytotoxicity assay with MTT method. The cells treated with various dilutions of CTAB solution, SWNTs suspensions in deionized water and SWNTs suspensions in CTAB solution were seeded into 96-well microplate. Cell without treatment served as a control group. The number of viable cells after 24 h incubation at 37 °C in a humidified atmosphere of 5% CO2 and 95% air was determined by the MTT assay. At the end of the incubation period, the medium with MTT was removed, and 100 μL dimethylsulphoxide was added to each well. The plate was shaken on the microplate shaker to dissolve the blue MTT-formazan. The relative viability of cells was expressed as color intensity of the number in the experimental wells relative to that of control. Absorbances were read at 570 nm on a microplate reader with background subtraction at 620 nm. Duplicates of each concentration were performed for each test. All assays were repeated three times to ensure reproducibility.
3 Results and discussion
3.1 Characterization and dispersion of SWNTs functionalized by CTAB
Field emission scanning electron microscopy (FE-SEM) showed that the SWNTs formed as bunches in powders due to their strong aggregation tendency (Fig.1). The strong van der Waals interactions endue the SWNTs with more hydrophobic characteristics.
In order to apply SWNTs as potential siRNA carriers, surface functionalization needs to be developed for good dispersion and separation of SWNTs. CTAB has been shown to provide a stable homogeneous dispersion of carbon nanotubes. XIAO et al[16] discussed the two-step mechanism of CTAB absorption on carbon nanotubes and suggested that a concentration of 9.6 ×10-4 mmol/L is the optimum CTAB concentration to disperse CNTs. Therefore, we prepared CTAB in deionized water at the concentration of 0.3 mg/mL, which was very close to 9.6 ×10-4 mmol/L, to make sure of the maximum absorption of CTAB on the surface of SWNTs and the good bind potential with siRNA.
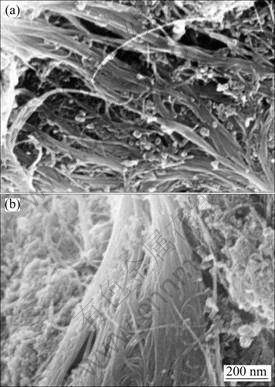
Fig.1 FE-SEM images of SWNTs powders: (a) SWNTs as intricate boundless; (b) Single nanotubes overlaid on surface of SWNTs bundles
As shown in Fig.2, the suspensions of SWNTs in CTAB and PL-PEG-NH2 solutions were well-dispersed and stable without deposition of SWNT powders on the walls and bases of the tubes, even after several days. The stability of the suspensions increased after being centrifuged.
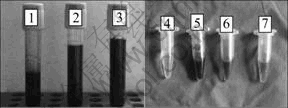
Fig.2 SWNT suspensions after being prepared in deionized water (1), CTAB solution (2), and PL-PEG-NH2 solution (3) for 2 h, and empty eppendorf tube (4), SWNT suspensions in CTAB solution (5) and centrifuged SWNT suspension in CTAB solution (6) after being prepared for 1 week, and deionized water(7)
The SWNTs dispersed well in CTAB solution (Fig.3) and the single nanotubes were coated by CTAB molecules (Fig.4). The dispersion of SWNTs in various solvents and aqueous surfactant emulsions was investigated with the Hansen solubility parameters (δt2=δd2+δp2+δh2) defined by HAM et al[17]. They found that the nanotubes were dispersed or suspended very well in solvents with certain dispersive component (δd) values. The surfactants with a lipophilic group equal to and longer than decyl, containing nine methylene groups and one methyl group, contributed to nanotube dispersion in water. CTAB ((C16H33)N(CH3)3Br) is a cationic surfactant according to the charge types of hydrophilic “heads” and have nonpolar and lipophilic hydrocarbon “tails”. It was hypothesized that CTAB could bind with SWNTs through the lipophilic “tails” absorbed on the nanotube surface and the charged hydrophilic “head” of CTAB separates the nanotubes from each other. A possible mechanism of CTAB absorption on SWNTs is shown in Fig.5.
The zeta potential of SWNTs in CTAB solution (0.3 mg/mL) was 32.38 mV in our experiment. According to XIAO et al[16], CNTs dispersed in CTAB solution have a negative zeta potential below 2.0×10-4 mol/L CTAB and a positive zeta potential above 2.0×10-4 mol/L CTAB. The positive zeta potential increases along with an increase of CTAB concentration and stabilizes at 9.6×10-4 mol/L CTAB. SWNT aggregation was prevented by electrostatic repulsion and steric effects of CTAB on the SWNT surfaces.
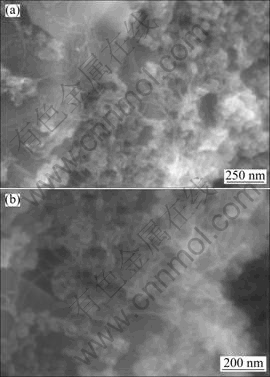
Fig.3 FE-SEM images of SWNTs dispersed in CTAB solution (a) and tangled nanotubes dispersed in matrix (b)
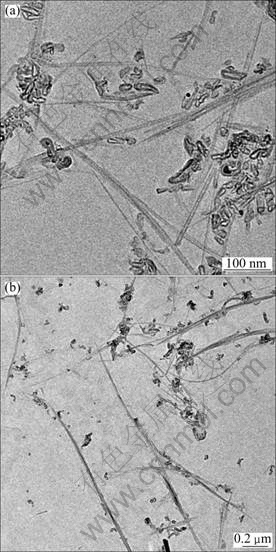
Fig.4 TEM images of SWNTs in CTAB solution (a) and CTAB adhere to side wall of SWNTs (b)
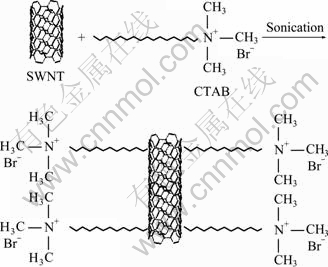
Fig.5 Schematic for CTAB absorption on SWNT surfaces
3.2 Gel electrophoresis
Figure 6 shows that the siRNA bands were present in these samples like undiluted siRNA sample, and siRNA in nuclease-free water, and siRNA in SWNT suspended in aqueous solution, and siRNA in CTAB- coated SWNTs at 1:2 and 1:4 volume ratio of SWNT:siRNA, and siRNA in PL-PEG coated SWNTs at different volume ratios. There were no siRNA bands in those samples like siRNA in the CTAB solution, siRNA in CTAB-coated SWNTs at 5:1, 2:1, 1:1, 0.7:1, 1:1.5, and 1:2.5 volume ratio of SWNT:siRNA. The siRNA bands demonstrated that free siRNA or incompletely neutralized siRNA migrated in the electric field towards the anode. In contrast, no siRNA band indicated that the stable complex of CTAB and siRNA plus or not plus SWNTs retarded in the sample wells due to large size and charge neutralization. WANG et al[18] proved that the cationic surfactant CTAB can combine with negative yeast RNA through electronic attraction and form ion association. The single nanotubes were coated by CTAB molecules and formed the structure of lipophilic hydrocarbon “tails” of CTAB binding the wall of SWNTs. The charged hydrophilic “head” of CTAB tended to bind the negative siRNA and the charge ratio(z+/-) of CTAB verse siRNA may determine the capability of SWNTs binding with siRNA. When the negative charge of siRNA exceeded the positive charge of CTAB, the excess siRNA would appear as bands in gel electrophoresis. So, we found that siRNA can be neutralized by CTAB-coated SWNTs when the volume ratio of SWNT:siRNA was below 1:1.5. But strangely, there was an exception at 1:2.5 of the volume ratio of SWNT:siRNA which manifested as no siRNA band in the electric field. We conjecture that there may be another mechanism involved in the interaction of siRNA and CTAB-coated SWNTs. We did not consider the impact of SWNTs to the electronic attraction between CTAB and siRNA.
According to the gel electrophoresis patterns of siRNA in Fig.6, the following deductions could be made. 1) If siRNA was uncombined or residual, it existed in the supernatant and RNA bands could be present. 2) The combination of CTAB-SWNT-siRNA may reach saturation between a volume ratio of 1:1.5 and 1:2. When the volume of siRNA was two times more than that of the SWNTs, there was an excess of siRNA and extra bands appeared. But strangely, there were no RNA bands at a 1:2.5 volume ratio of SWNT to siRNA, which did not fit with the other results. Further experiments are required to determine why this happened. 3) CTAB is the cross-linker between SWNTs and siRNA. SWNTs could not combine with siRNA if CTAB was absent. Single CTAB can also bind siRNA which results in the absence of RNA bands. (4) SWNTs, coated by PL-PEG, could not combine with siRNA, therefore, sharp and distinct RNA bands were present.
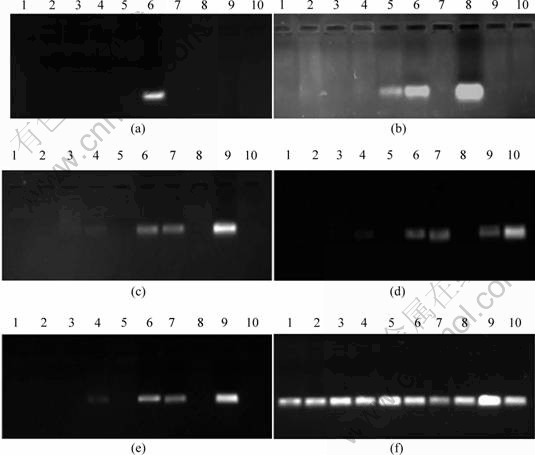
Fig.6 Agarose (1.5%) gel electrophoresis of single siRNA and composited siRNA in CTAB coated SWNT suspensions (a, b, c, d, e) and PL-PEG coated SWNT suspensions (f): Undiluted siRNA presented in lane 6(a), lane 8(b), lane 9(c), lane 10(d); siRNA in deionized water presented in lane 6(b), lane 7(c, d, e); the supernatant of 2:1 volume ratio of CTAB:siRNA sample presented in lane 7(b), lane 6(c), lane 8(d, e); siRNA in SWNT suspended in aqueous solution presented in lane 6(c), lane 9(d, e); the supernatant of 0.7:1 volume ratio of SWNT:siRNA presented in lane 2(b); the sediment of 0.7:1 volume ratio of SWNT:siRNA presented in lane 9(b); the supernatant of 1:1 volume ratio of SWNT:siRNA presented in lane 4(b), lane 2(c, d, e); the sediment of 1:1 volume ratio of SWNT:siRNA presented in lane 10(e); the supernatant of 1.5:1 volume ratio of SWNT:siRNA presented in lane 3(b), lane 1(c, d, e); the sediment of 1.5:1 volume ratio of SWNT:siRNA presented in lane 1(b), lane 10(c); the supernatant of 1:1.5 volume ratio of SWNT:siRNA presented in lane 3(c, d, e); the supernatant of 1:2 volume ratio of SWNT:siRNA presented in lane 5(b), lane 4(c, d, e); the sediment of 1:2 volume ratio of SWNT:siRNA presented in lane 10(b); the supernatant of 1:2.5 volume ratio of SWNT:siRNA presented in lane 5(c, d); the sediment of 1:2.5 volume ratio of SWNT:siRNA presented in lane 5(e); the supernatant of 1:4 volume ratio of SWNT:siRNA presented in lane 6(d, e); the supernatant of 2:1 volume ratio of SWNT:siRNA presented in lane 3, 4(a); the sediment of 2:1 volume ratio of SWNT:siRNA presented in lane 5(a); the supernatant of 5:1 volume ratio of SWNT:siRNA presented in lane 1, 2(a); various volume ratios like 1.5:1, 1:1, 1:1.5, 1:2, 1:2.5, 1:4, 5:1, 2:1, 0.7:1 of PL-PEG-SWNT to siRNA presented in lane 1 to lane 9 and siRNA in PL-PEG solution in lane 10(f)
3.3 Cytotoxicity of CTAB-coated SWNTs
Table 1 shows the absorbance values (OD570 nm) of the MTT test carried out for 24 h after human umbilical vein endothelial cells were incubated with various dilutions of CTAB solution, SWNTs suspensions in deionized water and SWNTs suspensions in CTAB solution. The cells viability were calculated through the formula which is cell viability =ODe/ODc×100%, and the ODe represented the absorbance value of experimental group while ODc represented the absorbance value of control group (see Fig.7).
From Fig.7, it was found that the SWNTs in deionized water have more cytotoxicity than the SWNTs in CTAB solution, and the cell viability would became lower and lower following the concentrations of SWNTs increased. The cytotoxicity of CNTs have been reported controversially in several types of cells in vitro. CUI et al[19] addressed a variety of factors affecting CNTs cytotoxicity like mental impurities, length and size distribution, surface area, dispersion and aggregation status, coating and functionalization, cell type. The surfactant treatment causes the dispersion of CNTs in the culture medium, thus contributing to less cytotoxicity[20]. In our experiments, CTAB made SWNTs dispersed well thus induced less cytotoxicity. But CTAB was also toxicity to human umbilical vein endothelial cells compared with control group. QIU et al[21] proved that CTAB molecules played a key role for Au NRs cytotoxicity. According to the results of QIU et al[21], CTAB is a cationic surfactant and cations are capable of causing mitochondrial dysfunction in subcellular level which manifested as inflammatory responses of mitochondrial, like the increasing in number, swelling in volume and disappearing of cristae. There is no evidence to indicate that CTAB enhanced the cytotoxicity of SWNTs in our experiments. The dispersion of SWNTs plays the key role in SWNTs cytotoxicity.
Table 1 Absorbance values at 570 nm obtained by MTT assay

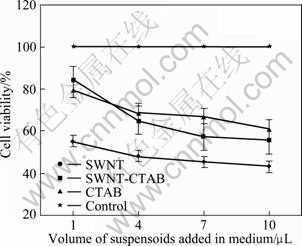
Fig.7 Cell viability of human umbilical vein endothelial cells incubated with CTAB, single SWNTs and CTAB-coated SWNTs at different concentration for 24 h
4 Conclusions
1) CTAB is a good surfactant for dispersing SWNTs. The lipophilic “tails” of CTAB makes it absorb onto the surface of SWNTs and the hydrophilic “heads” separate SWNTs into single nanotubes. In addition, the positively charged CTAB changes the surface zeta potential of SWNTs, which also contributes to the dispersion of SWNTs in CTAB solutions.
2) CTAB has an RNA binding tendency and is a good cross-linker between SWNTs and siRNA. SWNTs cannot be combined with oligonucleotides if CTAB is absent. The combination of CTAB-SWNT-oligonu- cleotides may reach saturation between volume ratios of 1:1.5 and 1:2.
3) Well dispersed SWNTs by CTAB behave less toxicity to human umbilical vein endothelial cells than single SWNTs and the cytotoxicity of CTAB-coated SWNTs depends on the concentration of CTAB-coated SWNTs.
References
[1] LI Xiao-ming, FAN Yu-bo, WATARI F. Current investigations into carbon nanotubes for biomedical application [J]. Biomed Mater, 2010, 5(2): 22001.
[2] KAM N W S, JESSOP T C, WENDER P A, DAI Hong-jie. Nanotube molecular transporters: Internalization of carbon nanotube–protein conjugates into mammalian cells [J]. J Am Chem Soc, 2004, 126: 6850-6851.
[3] KAM N W S, DAI Hong-jie. Carbon nanotubes as intracellular protein transporters: generality and biological functionality [J]. J Am Chem Soc, 2005, 127: 6021-6026.
[4] WU Wei, WIECKOWSKI S, PASTORIN G, BENINCASA M, KLUMPP C, BRIAND J P, GENNARO R, PRATO M, BIANCO A. Targeted delivery of amphotericin B to cells by using functionalized carbon nanotubes [J]. Angew Chem: Int Ed, 2005, 44: 6358-6362.
[5] YINGHUAI Z, PENG A T, CARPENTER K, MAGUIRE J A, HOSMANE N S, TAKAGAKI M. Substituted carborane-appended water-soluble single-wall carbon nanotubes: new approach to boron neutron capture therapy drug delivery [J]. J Am Chem Soc, 2005, 127: 9875-9880.
[6] PAN Bi-feng, CUI Da-xiang, XU Ping, OZKAN C, FENG Gao, OZKAN M, HUANG Tuo, CHU Bing-feng, LI Qing, HE Rong, HU Guo-han. Synthesis and characterization of polyamidoamine dendrimer-coated multi-walled carbon nanotubes and their application in gene delivery systems [J]. Nanotechnology, 2009, 20(12): 125101.
[7] SINGH R, PANTAROTTO D, MCCARTHY D, CHALOIN O, HOEBEKE J, PARTIDOS C D, BRIAND J P, PRATO M, BIANCO A, KOSTARELOS K. Binding and condensation of plasmid DNA onto functionalized carbon nanotubes: Toward the construction of nanotube-based gene delivery vectors [J]. J Am Chem Soc, 2005, 127(12): 4388-4396.
[8] PANTROTTO D, SINGH R, MCCARTHY D, ERHARDT M, BRIAND J P, PRATO M, KOSTARELOS K, BIANCO A. Functionalized carbon nanotubes for plasmid DNA gene delivery [J]. Angew Chem: Int Ed, 2004, 43: 5242-5246.
[9] ZANGMEISTER R A, MASLAR J E, OPDAHL A, TARLOV M J. Adsorption behavior of DNA-wrapped carbon nanotubes on self-assembled monolayer surfaces [J]. Langmuir, 2007, 23(11): 6252-6256.
[10] KRAJCIK R, JUNG A, HIRSCH A, NEUHUBER W, ZOLK O. Functionalization of carbon nanotubes enables non-covalent binding and intracellular delivery of small interfering RNA for efficient knockdown of genes [J]. Biochem Biophys Res Commun, 2008, 369: 595-602.
[11] YU Jun-rong, GROSSIORD N, KONING C E, LOOS J. Controlling the dispersion of multi-wall carbon nanotubes in aqueous surfactant solution[J]. Carbon, 2007, 45(3): 618-623.
[12] WHITSITT E A, ANDREW R B. Silica coated single walled carbon nanotubes [J]. Nano Letters, 2003; 3(6): 775-778.
[13] RYABENKO A G, DOROFEEVA T V, ZVEREVA G I. UV-VIS-NIR spectroscopy study of sensitivity of single-wall carbon nanotubes to chemical processing and Van-der-Waals SWNT/SWNT interaction. Verification of the SWNT content measurements by absorption spectroscopy [J]. Carbon, 2004, 42(8-9): 1523-1535.
[14] BAI Ying-chen, LIN Dao-hui, WU Feng-chang, WANG Zheng-yu, XING Bao-shan. Adsorption of triton X-series surfactants and its role in stabilizing multi-walled carbon nanotube suspensions [J]. Chemosphere, 2010, 79(4): 362-367.
[15] KAM NWS, LIU Zhuang, DAI Hong-jie. Functionalization of carbon anotubes via cleavable disulfide bonds for efficient intracellular delivery of siRNA and potent gene silencing [J]. J Am Chem Soc, 2005, 127: 12492-12493.
[16] XIAO Qi, WANG Ping-hua, JI Ling-ling, TAN Xiao-ke, OUYANG Lin-li. Dispersion of carbon nanotubes in aqueous solution with cationic surfactant CTAB [J]. Journal of Inorganic Materials, 2007, 22(6): 1122-1126. (in Chinese)
[17] HAM H T, CHOI Y S, CHUNG I J. An explanation of dispersion states of single-walled carbon nanotubes in solvents and aqueous surfactant solutions using solubility parameters [J]. Journal of Colloid and Interface Science, 2005, 286(1): 216-223.
[18] WANG Feng, YANG Jing-he, WU Xia, WANG Fei, DING Hong-hong. Investigation of the interaction between curcumin and nucleic acids in the presence of CTAB [J]. Spectrachimica Acta Part A, 2007, 67: 385-390.
[19] CUI H F, VASHIST S K, AL-RUBEANAN K, LUONG J H, SHEU F S. Interfacing carbon nanotubes with living mammalian cells and cytotoxicity issues[J]. Chem Res Toxicol, 2010, 23(7): 1131-1147.
[20] ZHANG L W, ZENG L, BARRON A R, MONTERIO-RIVIERE N A. Biological interaction of functionalized single-wall carbon nanotubes in human epidermal keratinocytes [J]. Int J Toxicol, 2007, 26: 103-113.
[21] QIU Yang, LIU Ying, WANG Li-ming, XU Li-geng, BAI Ru, JI Ying-lu, WU Xiao-chun, ZHAO Yu-liang, LI Yu-feng, CHEN Chun-ying. Surface chemistry and aspect ratio mediated cellular uptake of Au nanorods [J]. Biomaterials, 2010, 31: 7606-7619.
十六烷基三甲基溴化铵修饰的单壁碳纳米管和
寡核苷酸的结合及细胞毒性
阎雪彬1, 2, 谷永红2, 黄 东2, 甘 丽2, 邬力翔3, 黄利华2, 陈哲东1, 黄苏萍1, 周科朝1
1. 中南大学 粉末冶金国家重点实验室,长沙 410083;
2. 中南大学 湘雅三医院,长沙 410013;
3. 中南大学 湘雅医学院,长沙 410013
摘 要:应用十六烷基三甲基溴化铵(CTAB)作为表面活性剂修饰单壁碳纳米管,研究CTAB修饰后的单壁碳纳米管的分散情况和表面电荷情况;观察在场发射扫描电子显微镜和透射电子显微镜下的形貌,同时还研究CTAB修饰后的单壁碳纳米管与小干扰RNA结合的最佳浓度配比, 以及CTAB功能修饰后的碳纳米管对培养的人脐静脉内皮细胞的毒性。结果表明:CTAB修饰后的单壁碳纳米管分散良好,CTAB吸附到单根或成束的碳纳米管管壁上,表面带正电荷;与带负电荷的寡核苷酸分子小干扰RNA可以结合,并且CTAB-SWNT与小干扰RNA结合比例达到1:1.5到1:2之间时基本饱和;缺乏CTAB的单壁碳纳米管不能结合小干扰RNA;没有分散的单壁碳纳米管具有更大的细胞毒性,CTAB可以改善SWNTs的分散性,从而减轻单壁碳纳米管的细胞毒性。
关键词:单壁碳纳米管;十六烷基三甲基溴化铵;寡核苷酸;细胞毒性
(Edited by YANG Hua)
Foundation item: Project (30770838) supported by the National Natural Science Foundation of China; Project (2008WK2003) supported by Hunan Science and Technology Foundation, China; Project (2010QZZD006) supported by the Key Program of Central South University Advancing Front Foundation, China; Project (200806) supported by Opening Foundation of State Key Laboratory of Powder Metallurgy, Central South University, China
Corresponding author: GU Yong-hong; Tel: +86-731-88618326; E-mail: huangdong6619@yahoo.com.cn
DOI: 10.1016/S1003-6326(11)60826-1