Article ID: 1003-6326(2005)02-0314-05
Preparation and properties of Cu matrix composite reinforced by carbon nanotubes
CHEN Xiao-hua(陈小华)1, 2, LI Wen-hua(李文华)2, CHEN Chuan-sheng(陈传盛)2,
XU Long-shan(许龙山)2, YANG Zhi(杨 植)2, HU Jing(胡 静)2
(1. Institute of Materials Science and Engineering, Changsha University of Science and Technology, Changsha 410076, China;
2. College of Materials Science and Engineering, Hunan University, Changsha 410082, China)
Abstract: Cu matrix composites reinforced by carbon nanotubes(CNTs) were prepared. The effect of carbon nanotubes on mechanical and tribological properties of the Cu matrix composites were investigated. The chemical method for coating CNTs was reported. The morphology of the fracture surfaces and worn surface were examined by SEM. The results show that Cu/coated-CNTs composites have higher hardness, much better wear resistance and anti-friction properties than those of the reference Cu alloy (Cu-10Sn) and Cu/uncoated-CNTs composite sintered under the same conditions. The optimal mechanical properties of the composites occurred at 2.25%(mass fraction) of CNTs. The excellent wear resistance and anti-friction properties are attributed to the fiber strengthening effect of CNTs and the effect of the spherical wear debris containing carbon nanotubes on the tribo-surface.
Key words: carbon nanotube; copper; composite; tribological property CLC number: TQ127.1; O648.14
Document code: A
1 INTRODUCTION
Carbon nanotubes(CNTs) are attracting increasing scientific and technological interests with their novel properties and potential[1-3]. Tensile strength and elastic modulus of CNTs have been measured to be as high as 200GPa and 1000GPa, respectively, which are much higher than those of the whiskers[4]. The thermal conductivity of CNTs can be as high as 3000W/(m·K)[5], about seven times larger than that of copper (385W/(m·K)). In addition, a carbon nanotube can be either electrically conductive or semiconductive depending on its atomic structure.
These mechanical and physical properties make nanotubes an attractive candidate for use in composite material applications. As in most fiber or particle reinforced composite materials, in order to achieve the most efficient enhancement of properties, the reinforcement phase should be uniformly dispersed in the matrix. However, CNTs are strongly affected by Van der Waals forces due to their small size and large surface area. These forces give rise to the formation of aggregates, which make, dispersion of CNTs in matrix difficult, especially in metal matrix. In addition, pristine CNTs are well known for poor compatibility with other materials, which leads to severe structural inhomogeneities and poor connectivity between CNTs and matrix. Overcoming these problems is a critical issue in the fabrication of CNTs reinforced composite.
Substantial advances have been made, especially for CNTs/polymer composite prepared by repeated stirring[6, 7], solution-evaporation with high-energy sonication[8, 9], surfactant-assisted processing[10, 11] and interfacial covalent functionalization[12]. For the CNTs /metal composite, very few investigations have been made. Kuzumaki et al[13] characterized the processing and the mechanical properties of CNTs reinforced Al-based composite prepared by direct mixing followed by hot-pressing. This work indicates that the CNTs in the composites are not damaged during the preparation and that no reaction products at the CNTs/Al interface are visible after annealing for 24h at 983K. But the CNTs/Al composites have shown only a slight strength enhancement. This situation is mainly due to difficulty for dispersion of CNTs and well-adhered to the metal matrix. An et al[14] fabricated the alumina-CNTs composites by hot-pressing the alumina-CNTs powders which were synthesized in the catalytic decomposition of acetylene over alumina powders impregnated with iron catalysts. They found that the enhanced wear and mechanical properties in the range of 0-4% CNTs are a result of the CNT s behavior in reinforcing filler in composite, and did not reach the expected value. Reported studies seems to recommend additional studies. It may be speculated that significant improvement of dispersion ability of CNTs and compatibility of CNTs with metal matrix can be achieved by coating the nanotubes with metallic materials.
In this study, the electroless plating method is used to coat CNTs with nickel. The effect of coated CNTs addition on tribological behavior of copper composites is examined to provide essential information for applications.
2 EXPERIMENTAL
2.1 Preparation of CNTs and coating of CNTs with nickel
Carbon nanotubes CNTs were prepared by catalytic decomposition of acetylene. The catalysts were produced by concentrating nickel nitrate and magnesium nitrate solution to gelation and then grinding it to fine powder after sintered at 400℃.
CNTs growth was obtained on the catalyst at 700℃ with a flow rate of acetylene 40mL/min and nitrogen 400mL/min. The crude material was stirred in 3mol/L nitric acid and refluxed for 24h at 60℃, and then suspended and refluxed in 5mol/L HCl solution for 6h at 120℃. Then the CNTs were rinsed with distilled water and pure CNTs were obtained (Fig.1). Pre-activation was accomplished by dispersing acid-treated CNTs in a solution of 0.1mol/L SnCl2/0.1mol/L HCl for 30min, followed by rinsing in distilled water. The Sn2+ sensitized nanotubes were further activated in a aqueous solution of 0.0014mol/L PdCl2/ 0.25mol/L for another 30min. The activated nanotubes were washed with distilled water and then introduced into an electroless plating bath. The morphology and size of the nickel-coated nanotubes were analyzed by means of JEOL scanning electron microscope (SEM ).
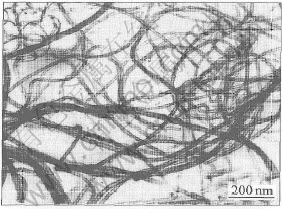
Fig.1 TEM image of acid treated carbon nanotubes
2.2 Preparation of Cu-CNTs composite
The coated CNTs powders or uncoated CNTs and Cu alloy(Cu-10Sn) powders with an average size of 15μm were mixed by ball milling for 30min, and then were hot-pressed in a graphite die in argon atmosphere at a temperature of 1100℃ under a pressure of 32MPa for 1h. Hardness was measured by a Vickers microhardness tester under a load of 5N. The fractured surfaces of hot-pressed specimens were examined by SEM.
The friction and wear tests were conducted by using a ring-on-plate tribometer in air at 20℃. The ring-on-plate configuration is shown in Fig.2. A composite plate is coupled with a bearing steel ring of 20mm in inner diameter and 30mm in outer diameter with a hardness of HRC 60. The plate specimens were fixed while the steel ring rotates over the plate. Rotation speed was controlled at 384r/min. A load of 50N was applied to the ring. The test duration was 10min. All experiments were run at room temperature under dry sliding conditions.
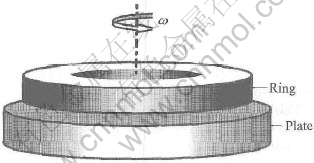
Fig.2 Ring-on-plate configuration
3 RESULTS AND DISCUSSION
Fig.3 shows the coating results which indicate that a layer of deposit is able to form on the surfaces of the CNTs. It can be seen that there are no voids or gaps on the nickel layer. The carbon nanotubes have been covered completely by a smooth and dense layer which is about 90-250nm in diameter.
Fig.4 shows the fractured surface morphologies of the hot-pressed samples of Cu/uncoated-CNTs and Cu/coated-CNTs . It can be seen that the specimen with uncoated CNTs has poor densification, and the CNTs are located in grain boundaries of copper in cluster (Fig.4(a)), while for Cu/coated-CNTs sample, coating CNTs with nickel has a positive influence on the densification. The CNTs are homogeneously distributed and does not adhere to each other at 2.25% of CNTs content. These indicate that CNTs can be effectively dispersed with CNTs coated by metallic materials. The CNTs tends to aggregate and locate in grain boundaries as the CNTs content is increased, which results in poorer cohesion between nanotubes and matrix(Fig.4(b)).
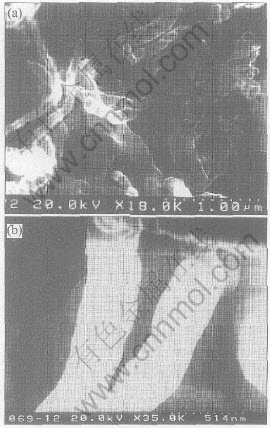
Fig.3 SEM images of nickel-coated carbon nanotubes
Table 1 shows the density, hardness and the wear loss of the three kinds of composites. It is seen that the density of Cu/uncoated-CNTs and Cu/coated-CNTs composite are lower than that of Cu alloy due to the existence of CNTs, while the hardness of the composites is higher than that of the specimen without CNTs. From Table 1 we can also see that the wear loss for Cu/uncoated-CNTs composite is the highest, as confirmed in Fig.4. It indicates the reinforcement role of coated CNTs in Cu-based composite. Fig.5 shows the hardness of the coated-CNTs composite increases with the increasing content of CNTs, and reaches the maximum value at 2.25% CNTs, which indicates the content of CNTs in Cu-based composite might af-[CM)][CM(22]fect the increase in hardness. Further addition of CNTs lower the microhardness significantly. This phenomenon is probably due to the difficulty in dispersing CNTs homogeneously in the composite and the problem of the poor cohesion between CNTs and the matrix.
Table 1 Density, microhardness and wear loss of Cu alloy and Cu/CNTs composite
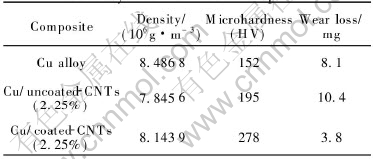
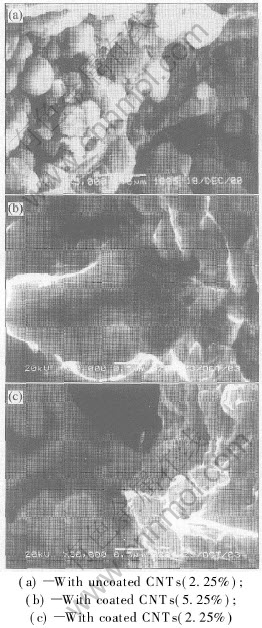
Fig.4 Fractured surface morphologies of hot-pressed copper composite
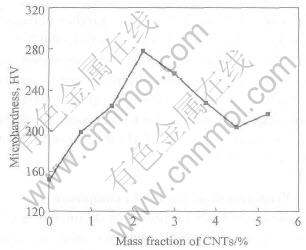
Fig.5 Variation of microhardness as function of CNTs content for Cu/coated-CNTs composite
The variation of wear loss and friction coefficient as a function of CNTs content are shown in Figs.6 and 7. Mass loss of the composite with about 2.25% coated-CNTs is decreased by nearly 51% as compared to that of the composite with 5.25% CNTs for coated-CNTs composite, while nearly 62% as compared to that of the composite with 2.25% CNTs for uncoated-CNTs listed in Table 1. Wear loss is significantly increased as the CNTs content is above 2.25%. This phenomenon can be also explained by the deterioration of the mechanical properties due to previously described difficulties in dispersing CNTs and poor cohesion. Figs.5 and 6 suggest that the addition of CNTs up to 2.25% has a positive on the reinforcement effect but negative influence on the densification at a higher CNTs content.
Fig.7 also shows that the friction coefficient decreases gradually as the CNTs content increases from 0 to 5.25% for coated-CNTs composite. The friction coefficient drop sharply at 0.75% CNTs content. Therefore, it can be concluded that CNTs which were coated by nickel can significantly improve the tribological performances of Cu-based composite.
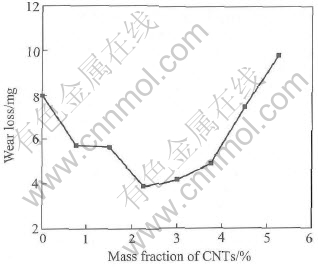
Fig.6 Variation of wear loss as function of CNTs for Cu/coated-CNTs composite
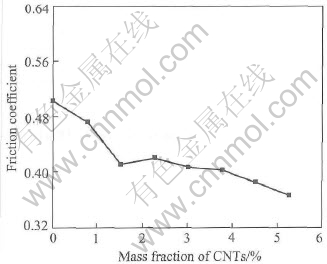
Fig.7 Variation of friction coefficient as function of CNTs content of Cu/coated-CNTs composite
The wear properties of the Cu-CNTs composite strongly depend on their constitution and microstructure. The CNTs disperse in the copper matrix block, or at least delay, the movement of dislocations in the metal copper and thus inhibit plastic deformation, which improves the wear behaviours of the composites. Furthermore, the addition of the CNTs can generate a lot of dislocations in the copper matrix and strengthen the copper matrix. During dry sliding wear, an existent stress at the interface resulted in plastic deformation for three composites to different extent. Because the uncoated-CNTs composite was softer than other two composites, asperity on the counterface could easily penetrate and cut into the surface. Fig.8(a) reveals the grooves and a considerable extent of peel ing, scaling on the worn surface of the uncoated CNTs composite, resulting in a certain amount of material loss. Addition of coated-CNTs to Cu-matrix considerably increases the hardness of the composites and results in reduction in the extent of plastic deformation of the matrix. The worn surface of coated-CNTs composites shown in Figs.8(b) and (c) exhibit shallow grooves, small scratches , cracks and crimples. On the worn surface of composite having 2.25% coated-CNTs shown in Fig.8(c), very little apparent cracks, scales and very fine scratches are presented. These results indicate that the composites having 2.25% coated-CNTs have excellent tribological properties better than others.
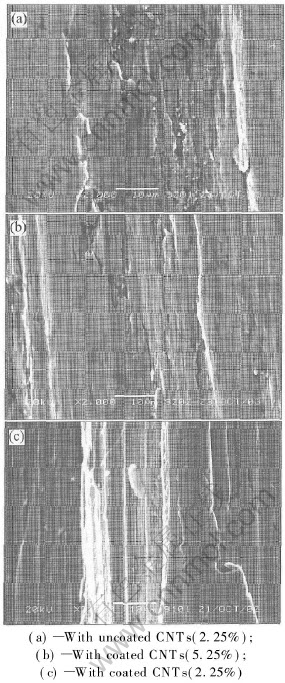
Fig.8 SEM micrographs of worn surface after wear tests of copper composite
As one dimension material with super strong mechanical properties, CNTs distribute in the composite with net-like structure, acting as load-bearing and load-transferring like steel backbone, apparently increase the stiffness and toughness of composite. On the other hand, the CNTs in the composite will slowly release on to the metal surface during the wear and friction test, and serve as spacers, preventing rough contact between the two mating metal surface, thereby slowing the wear rate considerably. In addition, in analogy to the well-known lubricating properties of Van der Waals-bonded graphite, the individual cylinders of CNTs might be expected to slide or rotate with respect to one another, and the broken CNTs produced during the test process would more easily or roll between the two mating metal surface, resulting in a decrease in the friction coefficient.
4 CONCLUSIONS
Copper-CNTs composites with CNTs ranging from 0 to 5.25% are fabricated to investigate the effect of uncoated CNTs and coated CNTs addition on wear resistance. The copper-CNTs composites were obtained by hot-pressing the mixture of Cu alloy and CNTs powders. The enhanced wear and mechanical properties are a result of the CNTs behaviour due to their good dispersion in copper matrix and good cohesion with copper matrix. The decrease in friction coefficient with increasing CNTs content is due to the lubricating properties of the CNTs and the rolling motion of CNTs at the interface between the two mating metal surface.
REFERENCES
[1]Collins P G, Zettle A, Bando H, et al. Nanotube nanodevice [J]. Science, 1997, 278:100-103.
[2]Tans S J, Devoret M H, Dai H, et al. Individual single-wall carbon nanotubes as quantum wires [J]. Nature, 1997, 386: 474.
[3]Kong J, Franklin N R, Zhou C W, et al. Nanotube molecular wires as chemical sensors [J]. Science, 2000, 287: 622-625.
[4]Treacy M M J, Ebbesen T W, Gibson J M, et al. Exceptionally high Youngs modulus observed for individual carbon nanotubes [J]. Nature, 1996, 381: 678-680.
[5]Berber S, Kwon Y K, Tomanek D. Unusually high thermal conductivity of carbon nanotubes [J]. Phys Rev Lett, 2000, 84: 4613-4616.
[6]JIA Zhi-jie, WANG Zheng-yuan, XU Cai-lu, et al. Study on poly(methyl methacrylate)/carbon nanotube composites [J]. Mater Sci Eng A, 1999, A271: 395-400.
[7]Sandler J, Shaffer M S P, Prasse T, et al. Development of a dispersion process for carbon nanotubes in an epoxy matrix and the resulting electrical properties [J]. Polymer, 1999, 40(21): 5967-5971.
[8]Perra P, Arup R B, Andreas J. Melt mixing of polycarbonate with multiwalled carbon nanotubes: microscopic studies on the state of dispersion [J]. European Polymer Journal, 2004, 40(1): 137-148.
[9]Ago H, Petritsch K, Shaffer M S P, et al. Composites of carbon nanotubes and conjugated polymers for photovoltaic devices [J]. Adv Mater, 1999, 11(15): 1281-1285.
[10]Coleman J N, Curren S, Dalton A B, et al. Percolation-dominated conductivity in a conjugated-polymer-carbon-nanotube composite [J]. Phys Rev B, 1998, 58(12): R7492-R7495.
[11]Musa I, Baxendale M, Amaratunga, et al. Properties of regioregular poly (3-octylthiophene)/multiwall carbon nanotube composites [J]. Synthetic Materials, 1999, 102(3): 1250-1252.
[12]Harry J B, Francisco P, Edgar A R, et al. SWNT-flled thermoplastic and elastomeric composites prepared by miniemulsion polymerization [J]. Nano Lett, 2002, 2(8): 802-805.
[13]Kuzumaki T, Miyazawa K, Ichinose H, et al. Processing of carbon nanotube reinforced aluminum composite [J]. J Mater Res, 1998,13(9): 2445-2449.
[14]An J W, You D H, Lim D S. Tribological properties of hot-pressed alumina-CNT composite [J]. Wear, 2003, 255: 677-681.
Foundation item: Projects(50372020; 59972031) supported by the National Natural Science Foundation of China; Project(01JJY2052) supported by Science Foundation of Hunan Province and Hunan University Research Foundation
Received date: 2004-11-20; Accepted date: 2005-01-18
Correspondence: CHEN Xiao-hua, Professor, PhD; Tel:+86-731-8821610; Fax: +86-731-8821483; E-Mail: hudacxh@sohu.com
(Edited by LONG Huai-zhong)