J. Cent. South Univ. Technol. (2010) 17: 1148-1154
DOI: 10.1007/s11771-010-0611-4
Luminescent properties dependence of water-soluble CdTe quantum dots on stabilizing agents and reaction time
LI Zheng(李峥)1, 2, WANG Yong-xian(汪勇先)1, ZHANG Guo-xin(张国欣)1, HAN Yan-jiang(韩彦江)1, 2
1. Radiopharmaceutical Centre, Shanghai Institute of Applied Physics, Chinese Academy of Sciences,
Shanghai 201800, China;
2. Graduate University, Chinese Academy of Sciences, Beijing 100039, China
? Central South University Press and Springer-Verlag Berlin Heidelberg 2010
Abstract: The influence of stabilizing agents and reaction time on the luminescent properties of water-soluble CdTe quantum dots (QDs) was discussed. The thioglycolic acid (TGA)-CdTe ODs were characterized by TEM, XRD and FTIR. It is found that larger-size QDs can be synthesized more easily when L-cysteine (Cys) or golutathione (GSH) is chosen as stabilizing agent and TGA is proper to prepare highly luminescent QDs because of the effect between Cd2+ and sulfhydryl group. Furthermore, the absorption wavelength, full width at half maximum (FWHM), stokes shift, photoluminescence (PL) quantum yield and PL stability of TGA-CdTe are strongly dependent on reaction time, in which the absorption wavelength changes against reaction time with an exponential function. The TGA-CdTe QDs prepared at 2 h possess more excellent luminescent properties.
Key words: CdTe quantum dots; stabilizing agent; reaction time; luminescent property
1 Introduction
Semiconductor quantum dots (QDs) with excellent luminescent properties have been paid much attention in biological imaging due to their broad excitation spectra, narrow emission bandwidth, high quantum yields, broad stokes shift and reduced tendency to photobleach compared with organic dyes [1-3]. The two existent general strategies of QDs preparation are an organometallic synthesis in highly boiling organic solvents [4-5] and the synthesis in an aqueous medium using thiols as stabilizing agents [6-7]. Although QDs prepared by organometallic route possess excellent luminescent properties and monodispersity, they are generally capped with hydrophobic ligands. After the substitution of the surface-capping molecules by hydrophilic molecules, the photoluminescence of QDs would decrease markedly, furthermore, it is rather complicated and time-consuming [8-9]. So, direct aqueous synthesis is used widely in order to improve the water-stability and biological compatibility of QDs. Moreover, direct synthesis in a water-phase system is more reproducible, less expensive, and less toxic [10-11].
CdTe QDs have become the subject of numerous investigations because of its high quantum yield, multicolor availability and functionality. The quantum yield value of 65% at room temperature was reported for organometallically prepared CdTe QDs [12]. Since the appearance of first report on the aqueous synthesis of CdTe QDs in 1996 [13], sufficient progress has been made to obtain water-soluble QDs with excellent luminescent properties (i.e., emission wavelength, full width at half maximum (FWHM), quantum yield, and stability). With the knowledge regarding nanocrystal growth [4, 14], the luminescent properties can be controlled to a certain extent, which is mainly dependent on QDs size and size distribution. Furthermore, different molar ratios of ligands (thioglycolic acid) to monomers (Cd2+ ions) in the precursor solution [15], pH value [16], synthesized in air or in nitrogen [7], coated by denatured BSA (dBSA) [17], Cd/Te molar ratio [18-19] and core-shell construction [20] also play an important role in the luminescent properties of QDs.
As reported previously, CdTe QDs were capped with thiols stabilizing agents through the reaction between Cd2+ and sulfhydryl group compound (SR) [15, 21] by the following equations.
Cd2+ + SR-
(Cd-SR)+ (1)
(Cd-SR)+ + SR-
Cd(SR)2 (2)
Therefore, different stabilizing agents could lead to different surface structures and affect the nucleation and growth kinetics of QDs [22]. In addition, it is generally believed that CdTe QDs with different emission wavelengthes could be prepared at different reaction time [7, 23]. However, there is not a special report to describe various luminescent properties of CdTe QDs synthesized in water-phase system at different reaction time systematically. In this work, CdTe QDs were prepared in water-phase system using thioglycolic acid (TGA), glutathione (GSH) and L-cysteine (Cys) as stabilizing agents. The growth rate and luminescent properties of water-soluble CdTe QDs with different stabilizing agents were discussed. In particular, the change trends of various luminescent properties of TGA-CdTe QDs versus reaction time were discussed. In addition, the TGA-CdTe QDs were characterized by TEM, XRD and FTIR.
2 Experimental
2.1 Chemicals
Tellurium powder (99.8%) was purchased from Aldrich (USA). TGA (90%), GSH (BR), Cys (BR), CdCl2·2.5H2O (AR), NaOH(AR) and NaBH4 (AR) were obtained from Shanghai Chemical Reagents Company of China and used as received. Deionized water was used throughout.
2.2 Preparation of water-soluble CdTe QDs with different stabilizing agents
Water-soluble CdTe QDs were prepared according to the procedure described elsewhere [7] with some changes. Briefly, NaHTe solution, prepared from tellurium powder and NaBH4, was added to N2-saturated cadmium chloride solution in the presence of stabilizing agent. The molar ratio of Cd2+:Te2-: stabilizing agents was fixed at 1.0:0.5:2.0. Then, the solution was adjusted to pH 9.0-9.5 with 1 mol/L NaOH solution. After mixing, the reaction solution was heated to 100 ℃ and refluxed for different time.
2.3 Characterizations and apparatus
UV-vis absorption spectra of CdTe QDs were obtained by using a Hitachi U-3010 spectrophotometer. The photoluminescence (PL) spectra of CdTe QDs were monitored by using a Hitachi F-4500 FL spectrophotometer and an excitation wavelength of 450 nm was used in this process. The TEM images were taken by Hitachi H-800 transmission electron microscope. XRD measurements were carried out by X-ray diffractometer D/MAX-2200. FTIR measurement was carried out on ThermoNicolet Avatar 370 Fourier transform infrared spectrometer in the range of 500- 4 000 cm-1. All measurements were carried out at room temperature without post-processing.
3 Results and discussion
3.1 Photoluminescence (PL) spectra of CdTe QDs with different stabilizing agents
As shown in Fig.1, all of the three compounds contain one sulfhydryl group in one molecule, so they would be potential stabilizing agents to prepare water-soluble CdTe QDs. The multicolor fluorescence is one of the most fascinating properties of QDs. Fig.2 shows the PL spectra of CdTe QDs with different stabilizing agents. Although the redshifts of emission wavelength would be observed in the PL spectra of different QDs when reaction went on, the PL intensity of TGA-CdTe QDs was higher than that of other two CdTe QDs obviously. Besides, TGA- and GSH-CdTe QDs possessed their higher PL intensity when reaction time was 2 h and the PL intensity of Cys-CdTe QDs was higher when reaction time was 0.5 h. Fig.3 shows the emission wavelengths of different CdTe QDs versus reaction time. It is found that the growth rate of GSH-CdTe QDs was faster than that of TGA- and Cys-CdTe QDs. Furthermore, the TGA-CdTe QDs would not grow as the reaction went on when its emission wavelength reached 620 nm in our experiment. As above description, the stabilizing agents can be used to form the Cd-SR and Cd(SR)2 complex compounds, in which the dithio-complexed Cd2+ ions have a better stabilization than monothio-Cd2+ complex, to control the amount of free cadmium ions via the complex equilibrium process [15]. The amount of monothio complex would be dominant when the stabilizing agent with long carbon chains is used because of the effect of steric hindrance. It could lead to an increasing concentration of free Cd2+ ions, which can provide a fast growth of CdTe QDs and lead to the coarse surface and low PL intensity. Furthermore, there would be enough occupation of surface sites by using stabilizing agent with short carbon chains. It would effectively replace Te atoms on the surface and lead to highly luminescent CdTe QDs [24]. TGA-Cd2+ is more stable than GSH-Cd2+, which would generate less free Cd2+ in post-reaction stage. So, there are not enough smaller particles to dissolve and provide the monomers for building the large ones. Therefore, the TGA-CdTe QDs would not grow to large ones when its emission wavelength reached 620 nm. As a whole, larger-size QDs can be synthesized more easily when L-Cys or GSH is chosen as stabilizing agent and TGA is proper to prepare highly luminescent QDs.
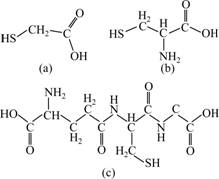
Fig.1 Structures of three stabilizing agents: (a) TGA; (b) L-Cys; (c) GSH
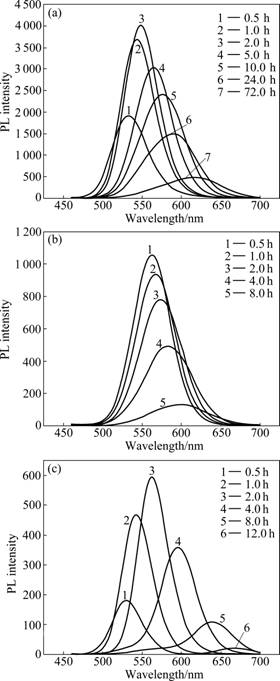
Fig.2 PL spectra of CdTe QDs with different stabilizing agents prepared at different reaction time: (a) TGA-CdTe; (b) L-Cys- CdTe; (c) GSH-CdTe
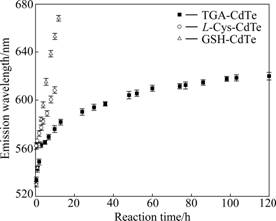
Fig.3 Emission wavelengths of different CdTe QDs versus reaction time
3.2 UV-vis absorption spectra of TGA-CdTe QDs
Due to the quantum confinement, the band gaps of QDs would change with the variation of particle sizes and further influence the absorption wavelength of the samples [4, 25]. As shown in Fig.4(a), the absorption wavelength of TGA-CdTe QDs shifted to red as the reaction went on. However, the intensity of absorption peak would decrease with its red shift. When the absorption wavelength was plotted as a function of reaction time, an overall shape of the relationship (Fig.4(b)) would emerge, which was the best fitted by an exponential function:
λabs=465.6+48.7[1-exp(-t/34.1)]+60.0[1-exp(-t/2.0)] (3)
where λabs is the absorption wavelength of a given sample of as-prepared QDs, nm; and t is the reaction time, h.
As size reduces, the oscillator strength is concentrated into just a few transitions and the electronic excitation shifts to high energy [26], which would lead to a shorter absorption wavelength. As shown in Fig.4(b), the absorption wavelength shifted to red relatively rapidly (from 490.0 to 541.5 nm) during the first 13 h because there were plenty of monomers in the precursor solution and nucleation took place rapidly right after the reactants were mixed. Subsequently (from 13 to 74 h), the wavelength moved more slowly (from 541.5 to 570.2 nm). At the end of reaction, the average size would reach a dynamic equilibrium [27] and the absorption wavelength kept at about 570 nm from 74 to 96 h (the corresponding PL emission wavelength changes from 611 to 617 nm).
According to Eq.(4) in Ref.[4], the variation of sizes versus reaction time could be shown by Fig.4(b). It was similar to the growth curve of CdSe [28] in highly boiling organic solvents. So, the growth mechanism of CdTe QDs in aqueous medium would be the same as in organic solvents. The nucleation occurs rapidly right after mixing and continues until the monomer concentration drops below a critical value. Then, the QDs
would grow through the process of Ostwald ripening.
D=(9.812 7×10-7)γ3-(1.714 7×10-3)γ2+
(1.006 4)γ-194.84 (4)
where D is the size of a given CdTe sample, nm; and γ is the absorption wavelength of the corresponding sample, nm.
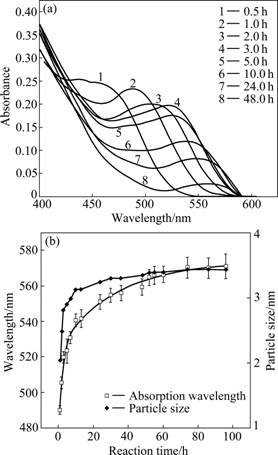
Fig.4 UV-vis absorption spectra of TGA-CdTe QDs prepared at different reaction time (a), and absorption wavelengths and particle sizes of TGA-CdTe QDs at different reaction time (b)
3.3 Full wave at half maximum (FWHM) and stokes shift of TGA-CdTe QDs
The FWHM is determined by the size distributions of CdTe QDs solution. There exists a critical size at any given monomer concentration. QDs smaller than the critical size would dissolve and larger ones grow at rates dependent strongly on size [28]. The critical size is smaller at the stage of nucleation. The smaller QDs grow faster than the larger ones because the QDs present in solution are all slightly larger than the critical size. When the monomer concentration is depleted due to growth, the critical size becomes larger, and then the distribution would broaden because some smaller QDs are shrinking and eventually disappear, while larger ones are still growing. So, as shown in Fig.5, the FWHM was “focused” from 72 to 48 nm during the first 2 h and then became broader as the reaction went on and reached 90 nm at about 86 h. After this, FWHM would keep stable because of the dynamic equilibrium in size distributions.
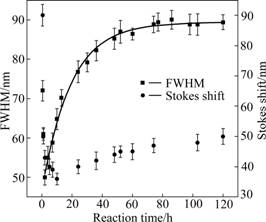
Fig.5 FWHM and stokes shift of TGA-CdTe QDs versus reaction time
Nucleation happens fast at the beginning of reaction and the particles have high specific surface area. It would lead to a frequent electron-hole-exchange interaction and make the excited-state electron lost energy easily and result in a broader stokes shift [29]. As shown in Fig.5, the stokes shift was 91 nm at the beginning stage of reaction. Then, it changes from 91 to 36 nm during the first 10 h because the distance between the first optically active state and the optically forbidden ground exciton state decreases with increasing size, leading to a decrease of the stokes shift in the luminescence [30]. Then, it would increase to 50 nm slowly due to the exciton– phonon coupling phenomenon when the particle is lager enough [31].
3.4 Photoluminescence (PL) quantum yield and stability of TGA-CdTe QDs
Solutions of both standard (Quinine sulfate) and samples (CdTe QDs) were adjusted to exhibit the same low absorption intensity at excitation wavelength of 350 nm. All quantum yields (η) were then determined from the following equation:
ηCdTe=ηQuinine sulfate(ACdTe/AQuinine sulfate) (5)
where ACdTe and AQuinine sulfate are the integrated PL spectra of the CdTe samples and the quinine sulfate standard, respectively. Fig.6(a) shows the influence of reaction time on the PL quantum yield of TGA-CdTe QDs. It is found that the quantum yield increases from 15% to 27% in the first 2 h because the surface defect decreases with the growth of QDs. Then, the effect of quantum confinement would become weaker as the size of QDs becomes larger, so the quantum yield would decrease with an exponential function as the reaction went on [15].
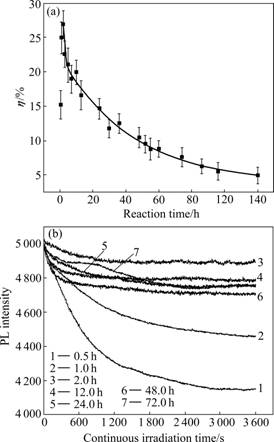
Fig.6 Photoluminescence (PL) quantum yield (η) (a) and stability (b) of TGA-CdTe QDs prepared at different reaction time
Stronger photoluminescence stability is an excellent property of QDs compared with organic dyes as labeling materials. The TGA-CdTe QDs prepared at different reaction time were adjusted to exhibit the same PL intensity at their emission wavelength, respectively. The PL intensity at their respective emission wavelength versus continuous irradiation time is shown in Fig.6(b). It is found that QDs prepared at 0.5 and 1.0 h exhibited weaker photoluminescence stability under continuous irradiation. Their PL intensity decreased markedly from 0 to 3 600 s. As the reaction continued, the QDs became more stable. The PL intensity of them decreased more slowly from 0 to 1 000 s. Subsequently, the PL intensity would keep stable even under continuous irradiation for 1.0 h.
3.5 Characterizations
As discussed above, the luminescent properties of TGA-CdTe QDs prepared at 2 h are more excellent. It has higher PL quantum yield, narrower FWHM and stronger PL stability. The TEM, XRD, SAED and FTIR analyses of this sample are shown in Fig.7. The TEM image shows that it was not sufficiently monodisperse and well separated because QDs made directly in water often easily aggregate [32]. However, it is found that the average size of the QDs was about 2.9 nm through the PCS analysis, which was close to the result in Fig.4(b). The XRD peaks at 23.9?, 41.1? and 46.6? corresponded to the crystal planes (111), (220) and (311), respectively, suggesting that the nanoparticles are in the cubic zinc blende form. The FTIR spectrum of TGA-capped CdTe QDs is shown in Fig.7(c), in which two intense bands were seen at 3 240 and 1 710 cm-1, respectively. Since OH and C=O stretching vibrations are known to be generally intense in FTIR spectrum, the intense band at 3 240 and 1 710 cm-1 can readily be assigned to the OH and C=O oscillator of TGA, respectively. Two weak bands also appeared at 3 006 and 2 860 cm-1, where CH stretching vibrations were expected. On the other hand, the weak band at 1 030 cm-1 can be assigned to a C—S stretching vibration. This proved that the QDs were capped with TGA through the reaction between Cd2+ and sulfhydryl group.
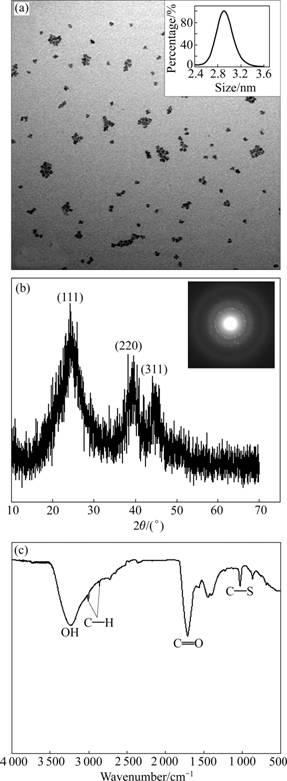
Fig.7 TEM (a), XRD and SAED (b), and FTIR (c) of TGA- CdTe QDs prepared at 2 h
4 Conclusions
(1) The growth rate and luminescent properties of CdTe QDs are dependent on the type of stabilizing agents. As a whole, larger-size QDs can be synthesized more easily when L-Cys or GSH is chosen as ligand stabilizing agents and TGA is proper to prepare high luminescent QDs.
(2) The luminescent properties of TGA-QDs are strongly dependent on the reaction time because of quantum confinement effect and surface effect. The absorption wavelength, FWHM, stokes shift, PL quantum yield and PL stability would vary with the change of reaction time regularly. The luminescent properties of TGA-CdTe QDs prepared at 2 h are more excellent. It has higher PL quantum yield, narrower FWHM and stronger PL stability.
References
[1] CHEN Liang-dong, LIU Jia, YU Xue-feng, HE Man, PEI Xiao-feng, TANG Zhao-you, WANG Qu-quan, PANG Dai-wen, LI Yan. The biocompatibility of quantum dot probes used for the targeted imaging of hepatocellular carcinoma metastasis [J]. Biomaterials, 2008, 29(31): 4170-4176.
[2] GAO X H, CUI Y Y, LEVENSON R M, CHUNG L W K, NIE S M. In vivo cancer targeting and imaging with semiconductor quantum dots [J]. Nat Biotechnol, 2004, 22(8): 969-976.
[3] CAI W B, SHIN D W, CHEN K, GHEYSENS O, CAO Q Z, WANG S X, GAMBHIR S S, CHEN X Y. Peptide-labeled near-infrared quantum dots for imaging tumor vasculature in living subjects [J]. Nano Lett, 2006, 6(4): 669-676.
[4] YU W W, QU L H, GUO W Z, PENG X G. Experimental determination of the extinction coefficient of CdTe, CdSe, and CdS nanocrystals [J]. Chem Mater, 2003, 15(14): 2854-2860.
[5] LIU Y S, SUN Y H, VERNIER P T, LIANG C H, CHONG S Y C, GUNDERSEN M A. pH-sensitive photoluminescence of CdSe/ZnSe/ZnS quantum dots in human ovarian cancer cells [J]. J Phys Chem C, 2007, 111(7): 2872-2878.
[6] KAPITONOV A M, STUPAK A P, GAPONENKO S V, PETROV E P, ROGACH A L, EUCHM?LIER A. Luminescence properties of thiol-stabilized CdTe nanocrystals [J]. J Phys Chem B, 1999, 103(46): 10109-10113.
[7] LIU Y F, CHEN W, JOLY A G, WANG Y Q, POPE C, ZHANG Y B, BOVIN J O, SHERWOOD P. Comparison of water-soluble CdTe nanoparticles synthesized in air and in nitrogen [J]. J Phys Chem B, 2006, 110(34): 16992-17000.
[8] TALAPIN D V, ROGACH A L, SHEVCHENKO E V, KORNOWSKI A, HAASE M, WELLER H. Dynamic distribution of growth rates within the ensembles of colloidal II-VI and III-V semiconductor nanocrystals as a factor governing their photoluminescence efficiency [J]. J Am Chem Soc, 2002, 124(20): 5782-5790.
[9] MATTOUSSI H, MAURO J M, GOLDMAN E R, ANDERSON G P, SUNDAR V C, MIKULEC F V, BAWENDI M G. Self-assembly of CdSe-ZnS quantum dot bioconjugates using an engineered recombinant protein [J]. J Am Chem Soc, 2000, 122(49): 12142-12150.
[10] GAPONIK N, TALAPIN D V, ROGACH A L, HOPPE K, SHEVCHENKO E V, KORNOWSKI A, EYCHM?LLER A, WELLER H. Thiol-capping of CdTe nanocrystals:An alternative to organometallic synthetic routes [J]. J Phys Chem B, 2002, 106(29): 7177-7185.
[11] ZHANG Hao, ZHOU Zhen, YANG Bai. The influence of carboxyl groups on the photoluminescence of mercaptocarboxylic acid-stabilized CdTe nanoparticles [J]. J Phys Chem B, 2003, 107(1): 8-13.
[12] TALAPIN D V, HAUBOLD S, ROGACH A L, KORNOWSKI A, HAASE M, WELLER H. A novel organometallic synthesis of highly luminescent CdTe nanocrystals [J]. J Phys Chem B, 2001, 105(12): 2260-2263.
[13] ROGACH A L, KATSIKAS L, KORNOWSKI A, SU D S, EYCHM?LLER A, WELLER H. Synthesis and characterization of thiol-stabilized CdTe nanocrystals [J]. Ber Bunsen-Ges Phys Chem, 1996, 100(11): 1772-1778.
[14] YU W W, WANG Y A, PENG X G. Formation and stability of size-, shape-, and structure-controlled CdTe nanocrystals: Ligand effects on monomers and nanocrystals [J]. Chem Mater, 2003, 15(22): 4300-4308.
[15] GUO Jia, YANG Wu-li, WANG Chang-chun. Systematic study of the photoluminescence dependence of thiol-capped CdTe nanocrystals on the reaction conditions [J]. J Phys Chem B, 109(37): 17467-17473.
[16] MANDAL A, TAMAI N. Influence of acid on luminescence properties of thioglycolic acid-capped CdTe quantum dots [J]. J Phys Chem C, 2008, 112(22): 8244-8250.
[17] WANG Q, KUO Y C, WANG Y W, SHING G, RUENGRUGLIKIT C, HUANG Q R. Luminescent properties of water-soluble denatured bovine serum albumin-coated CdTe quantum dots [J]. J Phys Chem B, 2006, 110(34): 16860-16866.
[18] DENG Da-wei, QIN Yuan-bin, YANG Xi, YU Jun-sheng, PAN Yi. The selective synthesis of water-soluble highly luminescent CdTe nanoparticles and nanorods: The influence of the precursor Cd/Te molar ratio [J]. J Cryst Growth, 2006, 296(2): 141-149.
[19] DAGTEPE P, CHIKAN V. Effect of Cd/Te ratio on the formation of CdTe magic-sized quantum dots during aggregation [J]. J Phys Chem A, 2008, 112(39): 9304-9311.
[20] HE Yao, LU Hao-ting, SAI Li-man, LAI Wen-yong, FAN Qu-li, WANG Lian-hui, HUANG Wei. Microwave-assisted growth and characterization of water-dispersed CdTe/CdS core-shell nanocrystals with high photoluminescence [J]. J Phys Chem B, 2006, 110(27): 13370-13374.
[21] WANG Fang-bin, FAN Mei-yi, LIU You-nian, WANG Jian-xiu, ZENG Dong-ming, HUANG Ke-long. Fabrication of ferrocenyl glutathione modified electrode and its application for detection of cadmium ions [J]. Journal of Central South University of Technology, 2008, 15(1): 44-48.
[22] PAN Dao-cheng, JI Xiang-ling, An Li-jia, LU Yun-feng. Observation of nucleation and growth of CdS nanocrystals in a two-phase system [J]. Chem Mater, 2008, 20(11): 3560-3566.
[23] GERHARDS C, DROST C S, SGOBBA V, GULEI D M. Conjugating luminescent CdTe quantum dots with biomolecules [J]. J Phys Chem B, 2008, 112(46): 14482-14491.
[24] BORCHERT H, TALAPIN D V, GAPONIK N, MCGINLEY C, ADAM S, LOBO A, M?LLER T, WELLER H. Relations between the photoluminescence efficiency of CdTe nanocrystals and their surface properties revealed by synchrotron XPS [J]. J Phys Chem B, 2003, 107(36): 9662-9668.
[25] ALIVISATOS A P. Semiconductor clusters, nanocrystals, and quantum dots [J]. Science, 1996, 271(5251): 933-937.
[26] MURRAY C B, BAWENDI M G, KAGAN C R. Synthesis and characterization of monodisperse nanocrystals and close-packed nanocrystal assemblies [J]. Annu Rev Mater Sci, 2000, 30: 545-610.
[27] TALAPIN D V, ROGACH A L, HAASE M, WELLER H. Evolution of an ensemble of nanoparticles in a colloidal solution: Theoretical study [J]. J Phys Chem B, 2001, 105(49): 12278-12285.
[28] PENG X G, WICKHAM J, ALIVISATOS A P. Kinetics of II-VI and III-V colloidal semiconductor nanocrystal growth: “Focusing” of size distributions [J]. J Am Chem Soc, 1998, 120(21): 5343-5344.
[29] CONDE J P, BHATTACHARJEE A K, CHAMARRO M, LAVALLARD P, PETRIKOV V D, LIPOVSKII A A. Photoluminescence stokes shift and exciton fine structure in CdTe nanocrystals [J]. Phys Rev B, 2001, 64(11): 113303.
[30] EFROS A L, ROSEN M, KUNO M, NIRMAL M, NORRIS D J, BAWENDI M. Band-edge exciton in quantum dots of semiconductors with a degenerate valence band: Dark and bright exciton states [J]. 1996, 54(7): 4843-4856.
[31] MACHOL J L, WISE F W, PATEL R C, TANNER D B. Vibronic quantum beats in PbS microcrystallites [J]. Phys Rev B, 1993, 48(4): 2819-2822.
[32] CAI W B, HSU A R, LI Z B, CHEN X Y. ?Are quantum dots ready for in vivo imaging in human subjects [J]. Nanoscale Res Lett, 2007, 2(6): 265-281.
(Edited by YANG You-ping)
Foundation item: Projects(10805069, 10405034) supported by the National Natural Science Foundation of China
Received date: 2009-12-23; Accepted date: 2010-04-08
Corresponding author: WANG Yong-xian, Professor; Tel: +86-21-59556884; E-mail: yongxianwangsinap@163.com