Trans. Nonferrous Met. Soc. China 23(2013) 1595-1601
Extrusion and precipitation hardening behavior of AZ91 magnesium alloy
M. THIRUMURUGAN, S. KUMARAN
Department of Metallurgical and Materials Engineering, National Institute of Technology, Tiruchirappalli 620 015, India
Received 27 July 2012; accepted 10 April 2013
Abstract: The as-cast AZ91 magnesium alloy was extruded at 250 °C, 300 °C and 350 °C, respectively with extrusion ratio of 4:1 followed by precipitation hardening treatment (T6). The coarse and segregated Mg17Al12 intermetallic precipitate in AZ91 magnesium alloy was refined and distributed uniformly in α-magnesium matrix by direct hot extrusion combined with precipitation hardening treatment. The dynamic recrystallization phenomenon, partial to full, was observed in this alloy with respect to the extrusion temperature. Ultimate tensile strength of this alloy is increased from 190 MPa (as cast) to 570 MPa after extrusion. The age hardening characteristics of AZ91 magnesium alloy is more dependent on the grain size. The peak hardnesses are obtained in the aging time of 35, 30 and 20 h for alloys extruded with ratio of 4:1 at three different temperatures. Uniform and fine precipitates of Mg17Al12 in AZ91 Mg matrix were confirmed by scanning electron microscope (SEM).
Key words: AZ91 magnesium alloy; Mg17Al12 precipitate; extrusion; precipitation hardening
1 Introduction
The most important property of magnesium alloys is high specific strength. This is the reason for the demand of magnesium alloys significantly in the automotive industries. Weight reduction improves the fuel efficiency and thus the exhaust emission is considerably reduced in automobiles [1]. However, due to the high production cost of magnesium alloys and relatively poor workability, in comparison with other engineering alloys, their application is more restricted. This is partly due to the moderate mechanical properties (i.e. yield stress) at room temperature, which are very less than those of aluminum alloys, resulting from the hexagonal close-packed structure of magnesium [2]. Among the magnesium alloys, Mg-Al-Zn and Mg-Al-Mn alloys are most commonly used in the automotive industry and particularly Mg-9Al-1Zn (mass fraction in %, AZ91) alloy occupies more than 50% of all the high pressure die castings because of its strength and castability [1]. Grain refinement is one of the most important methods for improving strength. In order to obtain desirable strength and good reliability, plastic working and subsequent heat treatment such as aging should be applied to the heat-treatable magnesium alloys which contain a large amount of alloying elements [3]. Better strength and ductility have been achieved in rheo-die cast AZ91 alloy at a temperature close to the solvus for a short exposure time [4]. So the extrusion at high temperatures induces dynamic recrystallization and grain growth. The grain boundaries must be pinned with the secondary-phase precipitates to inhibit grain growth. These precipitates can be present at triple points or grain boundaries before extrusion process [5,6]. However, during isothermal aging in the temperature range of 100-300 °C, the precipitation process appears to involve solely the formation of fine and coarse precipitates of the equilibrium phase (Mg17Al12). Commercial Mg wrought alloys are rarely heat treated after wrought processes, although most of them are precipitation hardening. This is because the age-hardening responses of commercial wrought alloys such as Mg-Zn (ZK) and Mg-Al-Zn (AZ) alloys are too low to achieve sufficient improvement by heat treatment after wrought processing [7]. Some studies were performed on cast AZ91 alloy, which was heat treated to various temperatures, to understand the solution treating behavior and hot forming characteristics of alloy [8]. The formation of the Mg17Al12 precipitate (β-phase) network is obviously detrimental to the mechanical properties, particularly ductility. Modification of the β-phase by the subsequent heat treatment is therefore essential to achieve the balanced mechanical properties. For this purpose, solution treatment at 430 °C followed by aging at 220 °C is usually applied for AZ91 alloys [9]. The modest age-hardening response of the AZ91 alloy might be partially attributed to the non-uniform distribution of the β continuous precipitates [10].
In this work, grain refinement and redistribution of the equilibrium precipitates by extrusion and precipitation hardening treatment were studied.
2 Experimental
The material chosen for this study was as-cast AZ91 magnesium alloy and the chemical composition of AZ91 Mg alloy is given in Table 1.
Table 1 Chemical composition of as-cast AZ91 magnesium alloy (mass fraction, %)

Identical cylindrical samples of 30 mm in diameter and 30 mm in height, by considering the aspect ratio of 1:1, were machined from the cast block for extrusion. Extrusion process of AZ91 magnesium alloys was processed at three different temperatures of 250, 300 and 350 °C with extrusion ratio 4:1 under controlled strain rate. The extrusion speed was maintained as 0.3 mm/s.
The microstructure of the extruded samples was studied by optical microscopy (MetaTech, Pune, India). The effect of extrusion temperature on mechanical properties of AZ91 magnesium alloy was examined by Zwick/Roell tensile testing machine. Then, the extruded samples were cut and subjected to precipitation hardening treatment in a muffle furnace with controlled environment. To avoid the oxidation of AZ91 alloys during precipitation hardening, the samples were packed in the graphite powders. The specimens were solutionized at 420 °C for 8 h and then artificially aged at 200 °C for various time intervals. Optical and scanning electron microscopes (Hitachi S3000-H) were used to study the microstructural evolution during solution and aging treatment. Zwick 3212001 Vickers micro hardness tester with load of 9.8 N was used for hardness measurements.
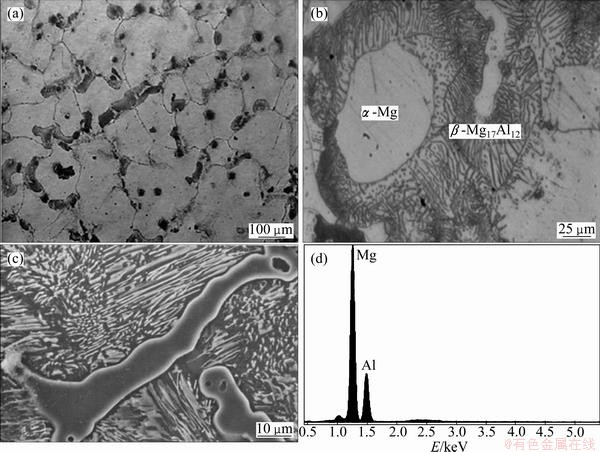
Fig. 1 Optical (a, b) and SEM (c) images and corresponding EDS result (d) of as-cast AZ91 magnesium alloy
3 Results and discussion
3.1 Microstructural analysis of as-cast AZ91 magnesium alloy
Micrographs of as-cast AZ91 magnesium alloy are shown in Fig. 1. The alloy consists of α-matrix grains (solid solution of Al and Zn in Mg) and Mg17Al12 (β-phase) intermetallic precipitate.
From the binary phase diagram of Mg-Al, Mg17Al12 is the equilibrium precipitate and the SEM image also confirms the morphology of the precipitates [11]. And further it is confirmed by XRD (see Fig. 2). Precipitates are randomly distributed along and adjacent to the grain boundaries. Due to the effect of coring during casting, Al-rich regions can be observed towards the periphery of each grain. The morphology of the precipitates is both continuous and discontinuous with lamellar pattern and lath, respectively.
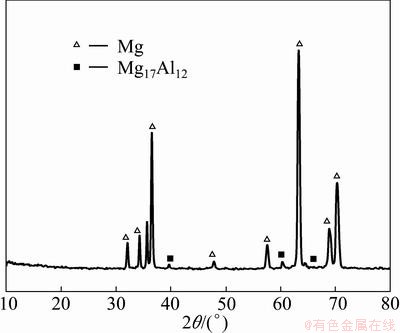
Fig. 2 XRD pattern of as-cast AZ91 alloy
The average size of the long lath precipitates is found to be about 10 mm in thickness and the length varies from 40 to 50 mm. The average grain size of the as-cast AZ91 Mg alloy is 350 μm
3.2 Structural changes during extrusion
Figure 3 shows the optical micrographs of AZ91 alloy extruded at different temperatures with extrusion ratio of 4:1.
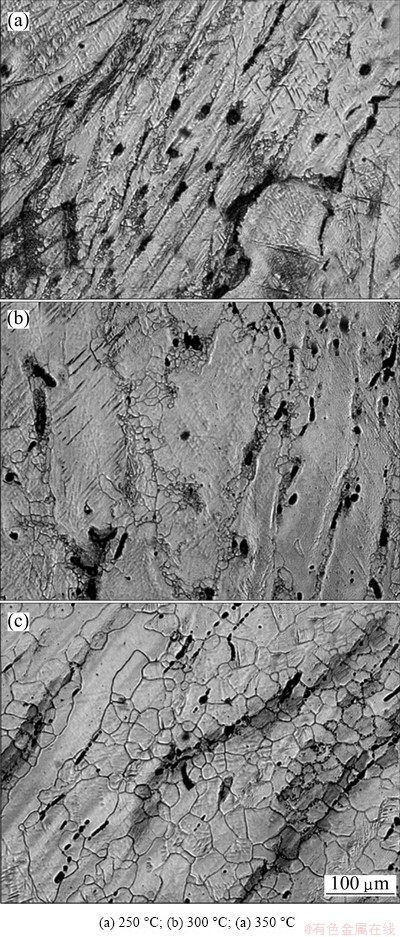
Fig. 3 Optical micrographs of AZ91 alloy extruded at different temperatures with extrusion ratio of 4:1 (along extrusion direction)
The grains are refined during the extrusion process and also the dynamic recrystallization adjacent to twin-matrix interfaces occurs. The samples extruded under different conditions exhibit dynamic recrystallized (DRX) grains. The DRX phenomenon is dependent on the temperature. The approximate grain size of α matrix and the amount and size of the precipitates were measured using linear intercept method. The exact measurement of grain size is not possible for the extruded magnesium alloys because it contains bimodal grains and DRX grains. The average grain sizes are 170, 200 and 230 μm for the alloys extruded at 250, 300 and 350 °C, respectively. It is bit difficult to measure the DRX grain size, because the DRX grains are mainly evolved adjacently to grain boundary or precipitates. However, the average DRX grain sizes are 27, 59 and 120 μm for the alloy extruded at 250, 300 and 350 °C, respectively. The amount of recrystallized grains is increased with the increase of extrusion temperature. The DRX grains are mainly evolved adjacently to the grain boundary regions, especially at the triple points, matrix– precipitate interface. Generally, the recrystallization phenomenon in Mg-based alloys is sluggish. However, the effect of temperature and deformation is considered to be the major factors on DRX phenomenon. The size of the DRX grain is finer for low temperature extrusion than that of the high temperature extrusion. The un-DRX grains in the alloy extruded at lower temperatures are elongated towards extrusion direction. Similarly, the equilibrium precipitate is fragmented and lined up to the extrusion direction. It is difficult to measure β phase, because it is broken heavily and spread randomly along the α-matrix. So, the amount of β phase was calculated by pseudo-color micrographs to differentiate the α-matrix and β phase. Figure 4 shows the comparison of amount of β phase with the matrix with respect to the processing temperatures. This reveals that the amount of β phase is decreased by increasing the processing temperature with the same extrusion ratio.
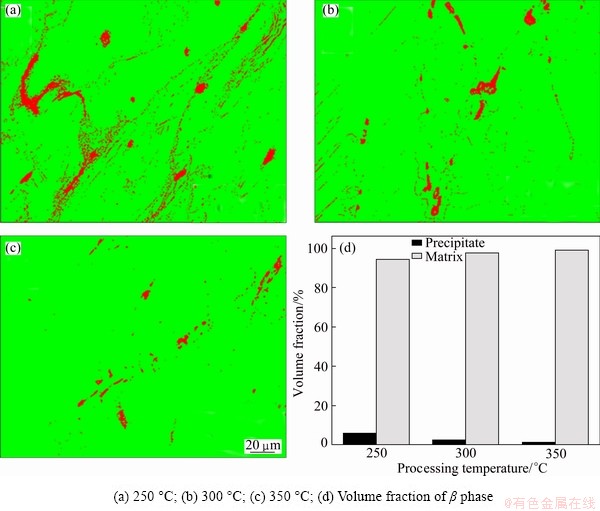
Fig. 4 Pseudo-color micrographs (a-c) and amount of β phase (d) in sample extruded at different temperatures with extrusion ratio of 4:1 along extrusion direction
Low temperature extrusion results in twins and slip bands. The alloy extruded at 300 °C reveals the accumulation of precipitates along and adjacent to the grain boundaries. It is also observed that the lamellar morphology transforms to random cluster morphology (Figs. 3(b) and (c)). The micrographs also exhibit a complete dynamic recrystallized, equiaxed grains adjacent to the grain boundary (Fig. 3(c)) for the alloy extruded at 350 °C. This is mainly attributed to the extrusion carried out at or above the recrystallization temperature. In general, the grain refinement in the extruded alloys is attributed to the plastic deformation. High temperature extrusion (350 °C) results in DRX grains predominantly and small fraction or islands of un-DRX, deformed grains. It is noteworthy to observe the partial dissolution of the equilibrium precipitates during high temperature extrusion.
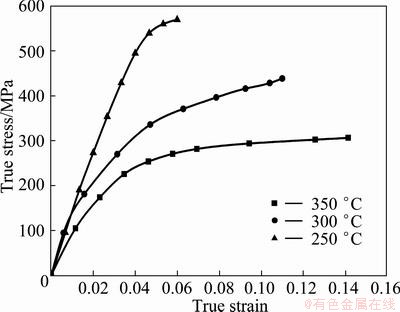
Fig. 5 True stress—strain curves of AZ91 magnesium alloy extruded at different temperatures with extrusion ratio of 4:1
3.3 Tensile behavior of extruded AZ91 Mg alloys
True ultimate tensile strength (UTS) and ductility of as-cast AZ91 Mg alloy are 190 MPa and 5%, respectively. Tensile behavior of the extruded AZ91 magnesium alloy is shown in Fig. 5. True ultimate tensile strength decreases with increasing the extrusion temperature. UTS of AZ91 Mg alloy is 560, 439 and 306 MPa for the alloy extruded at 250, 300 and 350 °C, respectively. However, the elongation of the extruded alloy is vice-versa, i.e., the ductility of the extruded alloy increases with increasing the extrusion temperature.
The improvement in ductility is attributed to the dynamic recovery and recrystallization during extrusion at higher temperature as well as the partial dissolution of precipitates. The sample extruded below recrystallization temperature (<300 °C) with extrusion ratio of 4:1, has higher UTS. This is mainly attributed to the presence of twins and strains. The redistribution of equilibrium precipitate is also an important factor to enhance the UTS. Another important reason would be the presence of DRX grains forming a necklace pattern on the deformed grains. The alloys extruded above the recrystallization temperature have strain-free equiaxed grains with controlled grain size. Therefore, there is a reduction in UTS compared with lower extrusion processing temperature. UTS of the alloy extruded at 350 °C decreases to 306 MPa and the elongation increases to 14% compared with those extruded at 250 °C with the same extrusion ratio. The extrusion temperature has significant effect on the strength and elongation. The average grain size increases and the volume fraction of the precipitate decreases with extrusion temperatures increasing [12]. The yield strength of the extruded AZ91 Mg alloy has a similar trend as UTS with increasing the extrusion temperature.
3.4 Precipitation hardening behavior of extruded AZ91 magnesium alloy
Figure 6 shows the microstructures of cast/extruded AZ91 Mg alloy under solutionized condition. These micrographs show the complete dissolution of Mg17Al12 precipitates into the α-matrix. The grain size of the cast alloy and solutionized cast AZ91 alloy is almost similar. There is no much change in the grain size during solution treatment of the cast alloy. But in the case of extruded alloy, there is a change in the grain size with respect to the extrusion temperature. The micrograph of the alloy extruded at 250 °C followed by solution treatment shows slightly finer grain size than the extruded one. This is mainly attributed to the recrystallization of cold/warm worked alloy during solution treatment. The other two conditions also show finer grain size over as-extruded alloy. The grain size is 150-200 μm for the extruded alloy with equilibrium precipitates.
After solutionizing treatment, the grain size is refined to less than 50 μm. This is due to the dominance of recovery and recrystallization. The hardness measurement of the solutionized alloy is in good agreement with the grain size. The hardness is in the range of HV50-60 after solution treatment.
Figure 7 shows the aging curves for the alloy extruded at three different temperatures with extrusion ratio 4:1. The determined peak hardnesses are HV 91 (35 h), HV 87 (30 h) and HV 85 (20 h) for the alloy extruded at different temperatures followed by solutionizing under identical conditions. The hardnesses obtained from different samples are more or less similar, but the peak hardnesses were obtained at different aging time.
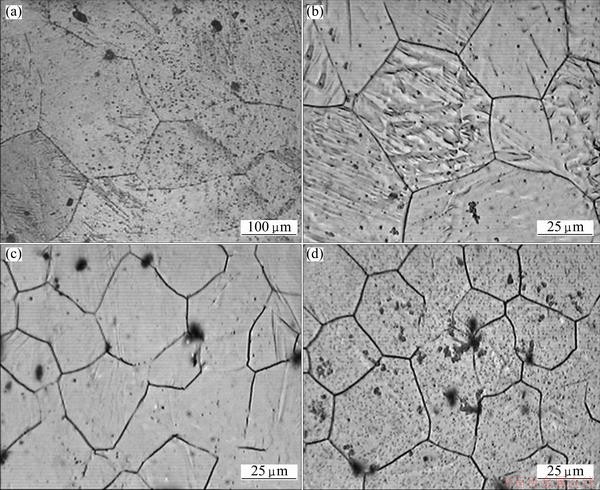
Fig. 6 Optical micrographs of solutionized cast alloy (a) and extruded alloy at 250 °C (b), 300 °C (c) and 350 °C (d)
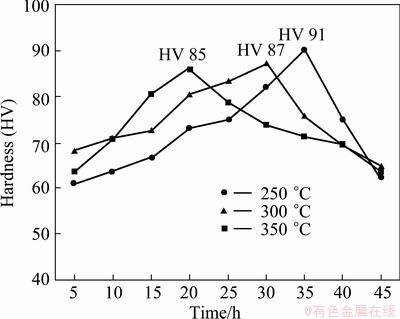
Fig. 7 Aging curves for alloy extruded at different temperatures with extrusion ratio of 4:1
The alloy extruded at 350 °C followed by solution treatment shows peak hardness at early time interval, at the other two extruding temperatures, the aging interval increases with decreasing the extrusion temperature. In another way, the grain size of the extruded alloy is an important factor for getting early peak hardness, namely, fine grained alloy exhibits peak hardness at early interval. It is in agreement with the microstructure. The grain size of the alloy under solutionized condition is 108 μm, and 70 μm for the alloy extruded at 250, 300 and 350 °C, respectively. This is because of more grain boundary area in fine grained materials; hence, the ejection of aluminium from supersaturated solution is fast and the same reactions with matrix elements form an equilibrium compound spread over the matrix. The hardness of the over-aged sample is also the same.
Figure 8 shows the SEM images of the aged samples. During aging, the β-phase precipitates form as discontinuous precipitation. The discontinuous precipitation is the cellular growth of alternating layers of β-phase where the continuous precipitation region ceases its growth early in the precipitation process. At higher magnification, the fine precipitates widely spread throughout the grain are clearly visible. This fine precipitates throughout the matrix are responsible for the increase in the hardness.
4 Conclusions
1) As-cast AZ91 alloy consists of α-Mg and both lath and cellular morphology of Mg17Al12 precipitates which are distributed along and adjacent to of the grain boundaries. After extrusion at different temperatures with extrusion ratio of 4:1, the cellular morphology transforms to cluster along the grain boundaries. High temperature extrusion at 350 °C exhibits more dynamic recrystallized grains adjacent to the grain boundaries.
2) Tensile behaviour of the extruded alloy is dependent on the extrusion temperature. The strength (true UTS and yield strength) decreases with increasing the extrusion temperature and the elongation is vice versa with the extrusion temperature. The higher strength at low extrusion temperatures is mainly attributed to the fine DRX grains which form a necklace pattern over the un-DRX grains and redistribution of Mg17Al12 precipitates. Higher ductility at higher extrusion temperatures is due to the dynamic recovery and recrystallization and a partial dissolution of Mg17Al12 precipitates in the matrix.
3) Solution treatment of extruded alloys reveals complete dissolution of Mg17Al12 equilibrium compound and magnitude of grain refinement during extrusion. Peak hardness of the aged alloy is increased from HV 50 to HV 90 due to uniform distribution of precipitate in the matrix along with grain refinement.
4) SEM images of the aged samples show uniform distribution of precipitates in both matrix and grain boundaries.
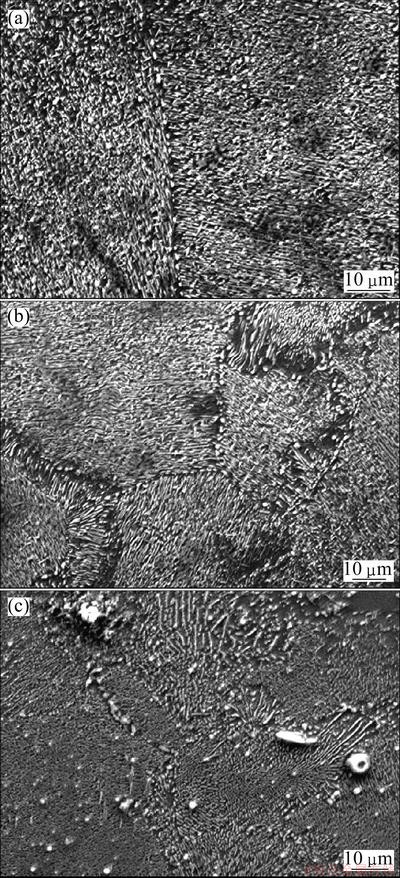
Fig. 8 SEM images of samples aged at 250 °C (a), 300 °C (b) and 350 °C (c)
References
[1] XU S W, MATSUMOTO N, KAMADO S, HONMA T, KOJIMA Y. Effect of Mg17Al12 precipitates on the microstructural changes and mechanical properties of hot compressed AZ91 magnesium alloy [J]. Mater Sci Eng A, 2009, 523: 47-52.
[2] MUSSI A, BLANDIN J J, SALVO L, RAUCH E F. Resistance to strain-induced damage of an ultrafine-grained magnesium alloy deformed in superplastic conditions [J]. Acta Mater, 2009, 54: 3801-3809.
[3] HONO K, MENDIS C L, SASAKI T T, OHISHI K. Towards the development of heat-treatable high-strength wrought Mg alloys [J]. Scripta Mater, 2010, 63, 7: 710-715.
[4] WANG Y, LIU G, FAN Z. A new heat treatment procedure for rheo-diecast AZ91D magnesium alloy [J]. Scripta Mater, 2006, 54: 903-908.
[5] SASAKI T T, YAMAMOTO K, HONMA T, KAMADO S, HONO K. A high-strength Mg-Sn-Zn-Al alloy extruded at low temperature [J]. Scripta Mater, 2008, 59: 1111-1114.
[6] CHANDRASEKARAN M, JOHN Y M S. Effect of materials and temperature on the forward extrusion of magnesium alloys [J]. Mater Sci Eng A, 2004, 381: 308-319.
[7] BOURGEOIS L, MUDDLE B C, NIE J F. The crystal structure of the equilibrium phase in Mg-Zn-Al casting alloys [J]. Acta Mater, 2001, 48: 1775-1787.
[8] WANG J G, LU P, WANG H Y, LIU J F, JIANG Q C. Semisolid microstructure evolution of the predeformed AZ91D alloy during heat treatment [J]. J Alloys Compd, 2005, 395: 108-112.
[9] CELOTTO S, BASTOW T J. Study of precipitation in aged binary Mg–Al and ternary Mg–Al–Zn alloys using 27Al NMR spectroscopy [J]. Acta Mater, 2001, 49: 41-47.
[10] KAYA A A, UZAN P, ELIEZER D, AGHION E. Electron microscopical investigation of as cast AZ91D alloy [J]. Mater Sci Technol, 2000, 16: 1001-1006.
[11] FRIEDRICH H E, MORDIKE B L. Magnesium technology, metallurgy, design data, applications [M]. New York: Berlin, Heidelberg, Springer, 2006.
[12] DING H, LIU L, KAMADO S. Study of the microstructure, texture and tensile properties of as-extruded AZ91 magnesium alloy [J]. J Alloys Compd, 2008, 456: 400-406.
AZ91镁合金的挤压和析出硬化行为
M. THIRUMURUGAN, S. KUMARAN
Department of Metallurgical and Materials Engineering, National Institute of Technology, Tiruchirappalli 620 015, India
摘 要:挤压比为4:1,将铸态AZ91镁合金分别在250,300 和 350 °C下进行挤压,随后进行析出硬化处理(T6)。经过热挤压和析出硬化处理后,铸态AZ91镁合金中粗大的和偏析Mg17Al12析出相被细化并均匀分布在α-镁基体中。在不同的挤压温度下合金中发生了部分或全部动态再结晶。经挤压后,该合金的极限抗拉强度从铸态的190 MPa增加到570 MPa。AZ91镁合金的时效硬化特征与晶粒尺寸有关。在250、300 和 350 °C下以4:1的挤压比挤压该合金后,获得峰值硬度的时效时间分别为35、30和20 h。SEM观察到在AZ91基体中存在均匀细小的Mg17Al12析出相。
关键词:AZ91镁合金;Mg17Al12析出相;挤压;析出硬化
(Edited by Xiang-qun LI)
Corresponding author: S. KUMARAN; Tel: +91-9944434705; Fax: +91-431-2500133; E-mail: kumara_rec@yahoo.co.in
DOI: 10.1016/S1003-6326(13)62636-9