
Dialkyl phosphinic acids: Synthesis and applications as extractant for nickel and cobalt separation
LI Lin-yan(李林艳)1, 2, XU Sheng-ming(徐盛明)1, JU Zhong-jun(居中军)1, ZHANG Zhang (张 章)3,
LIAO Fu-hui(廖复辉)2, LI Guo-bao(李国宝)2
1. Institute of Nuclear and New Energy Technology, Tsinghua University, Beijing 100084, China;
2. Beijing National Laboratory for Molecular Sciences, Beijing 100080, China;
3. College of Chemistry and Material Science, Sichuan Normal University, Chengdu 610066, China
Received 6 July 2009; accepted 30 December 2009
_____________________________________________________________________________________________________
Abstract: Three kinds of dialkylphosphinic acids (DAPAs), i.e. dihexylphophinic acid (DHPA), di-(2,4,4-trimethylpentyl) phosphinic acid (DTMPPA) and didecylphophinic acid (DDPA), were synthesized through free radical addition reaction. The influence of the types of initiator, reation time and reaction temperature on the yield of DAPAs were investigated. The products were characterized by NMR and MS. By using DHPA, DTMPPA and DDPA (10% in kerosene) as extractants, the extraction of Co2+ and Ni2+ in sulphate medium at different equilibrium pH values were measured. The results show that the maximum yield of DHPA, DTMPPA and DDPA can all be achieved at about 130 ℃ under the initiation of di-tert-butyl peroxide (DTBP). All the extraction of cobalt with respect to DHPA, DDPA and DTMPPA precedes that of nickel. The difference in pH1/2 value (defined as the pH at which 50% metal extraction occurs) between cobalt and nickel increases in the following sequence from large to small: DHPA, DDPA and DTMPPA, which indicates that the separation ability for cobalt and nickel ascends from DHPA, DDPA to DTMPPA.
Key words: dialkylphosphinic acid; free radical addition; extraction; cobalt/nickel separation
_____________________________________________________________________________________________________
1 Introduction
Organophosphorus acid extractants have been widely studied and applied in the fields of hydrometallurgy. For example, di-(2-ethylhexyl) phosphoric acid (D2EHPA) has been used to extract many metal ions from sulphate or chloride solution[1-2]; 2-ethylhexyl phosphonic acid mono 2-ethylhexyl ester (PC88A) exhibits high separation coefficient for cobalt and nickel[3]. In recent years, much attention has been paid to dialkyl phosphinic acids[4-6] because there are no ester-O atoms in their structures and thus exhibit higher pKa value, which makes their extraction for metal ions need low acidity of the water phase and thus they are easy to be stripped. The typical example, di-2, 4, 4-trimethylpentylphosphinic acid (trade name is Cyanex 272) possesses excellent extraction performance for separation of Co/Ni, rare earths and In/Ga etc[7-10].
Dialkyl phosphinic acids are mainly synthesized by Grignard reaction and free radical addition reaction[11-15]. As for Grignard reaction, three steps are needed, in which Grignard reagent is used for alkylation of phosphorus. Therefore, it needs rigorous reaction condition and has low yield, which restricts its industry application. In the case of free radical addition reaction, highly toxic phosphine is used to produce phosphorus free radical and olefin as alkyl resource, in which pressure is essential and olefinic polymerization is hard to avoid. As a result, highly purified dialkyl phosphinic acids are relatively difficult to be synthesized, and thus there is a lack of systematic research on the synthesis of dialkyl phosphinic acids and the relationship between their structures and extraction performance.
In this work, dihexylphosphinic acid (DHPA), didecylphosphinic acid (DDPA), and di-2, 4, 4- trimethylpentylphosphinic acid (DTMPPA) were synthesized with free radical addition reaction method by using hypophosphorous acid as phosphorous resource, and their synthetic condition and extraction performance were investigated in detail.
2 Experimental
2.1 Synthesis
2.1.1 DHPA
The procedure was similar to that reported by WO et al[14], with some modifications. Following was the general process.
10 g (0.094 3 mol) of NaH2PO2?H2O, 10 g of acetic acid, 19 g (0.226 mol) of 1-hexene and small amount of peroxide were charged into a 100 mL autoclaves. With stirring, the mixture was heated for a period of time. 50 mL of cyclohexane was added to the completed reaction mixture. Then, the mixture was washed by 20 mL of water twice to remove sodium acetate. The resultant organic phase was washed with 20 mL of 4% NaOH solution for 3-4 times to remove monoalkylphosphinic acids, and the aqueous layer was removed. The volatile components were removed by vacuum distillation. Then the waxy crude product was dispersed in proper quantity of water. Through centrifugation, the suspended liquor was divided into three phases in which the upper, middle and lower layers were polyene, sodium dihexyl phosphinic acid and aqueous phase, respectively. Then the upper and lower layers were removed. The middle layer was dissolved in 30 mL of cyclohexane, then was acidified and washed with 30 mL of 10% sulfuric acid solution and 30 mL water in turn, and the resultant aqueous phase was removed. White powders were obtained after removing the volatile components by vacuum distillation. The product was pure DHPA as confirmed by 1H-NMR, 31P-NMR (Nuclear magnetic resonance) and ESI-MS (Electronic spray ion/mass spectrum). Analysis: 1H-NMR δ (10-6): 0.84-0.88 (t, 6H, 2CH3), 1.24-1.38 (m, 16H, 8CH2), 1.62-1.65 (t, 4H, 2CH2—P),10.04 (s, 1H, P—OH); 31P NMR δ (10-6): 59.95 (s, P); ESI-MS m/z: 234.7 [M+H]+. The influence of the type of initiator, reaction time and reaction temperature on the reaction was investigated.
2.1.2 DTMPPA
The synthesis and post-treatment procedure was also identical to that of DHPA. The feed olefin was diisobutylene composed 80% α-type and 20% β-type. The product was colorless liquid which was pure DTMPPA as confirmed by 1H-NMR, 31P-NMR and ESI-MS. Analysis: 1H NMR δ (10-6): 0.88-0.94 (m, 24H, 8CH3), 1.10-1.14 (d, 4H, 2CH2),1.18-1.23 (m, 2H, 2CH),1.51-1.73 (m, 4H, 2CH2—P),9.93 (s, 1H, OH); 31P NMR δ (10-6): 60.06 (s, P); ESI-MS m/z: 291.1 [M+H]+, 313.2 [M+Na]+. The influence of the type of initiator, reaction time and reaction temperature on the reaction was also investigated.
2.1.3 DDPA
The synthesis and post-treatment procedure was identical to that of DHPA. The feed olefin was the mixture of 2, 7-dimethyl octene-1 and 3-methyl nonene-1 which was byproduct of a petrochemical plant. Because the boiling point of the feed olefin was about 160 ℃ which was higher than the reaction temperature, the reaction could be carried out under ordinary pressure. The product was amber oily liquid, which was a mixture of didecyl phosphinic acid containing 25% di-(2, 7-dimethyloctyl)phosphinic acid, di-(3-methylnonyl) phosphinic acid and 50% 2, 7-di-methyloctyl (3-methylnonyl)phosphinic acid as confirmed by 1H-NMR, 31P-NMR and ESI-MS. Analysis: 1H-NMR δ (10-6): 0.89 (m, 15H, 5CH3), 1.26 (s, 20H, 10CH2), 1.61 (m, 7H, 3CH, 2CH2P), 11.79(s, 1H, OH); 31P NMR δ (10-6): 60.86 (s, P); ESI-MS m/z: 346[M-H]-. The influence of the type of initiator, reaction time and reaction temperature on the reaction was also investigated.
2.2 Extraction procedure
DHPA, DDPA and DTMPPA were used as extractants. The sodium salts of the extractants (60% neutralised) were prepared by adding stoichiometric amount of concentrated NaOH solution to the extractants in kerosene and stirring the phases to form a single phase. These solutions were diluted with kerosene to 10% (volume fraction). The initial concentration of nickel(Ⅱ) and cobalt(Ⅱ) in the aqueous phase were 0.02 mol/L during the tests for determining the relationship between percentage extraction Ex and equilibrium pH value. All the extraction and stripping tests were carried out at room temperature with an aqueous to organic phase volume ratio of 1?1. The two phases were contacted for 5 min and the pH value of the aqueous phase was adjusted with 4 mol/L NaOH and 2 mol/L H2SO4 and was measured with a pH meter. The concentration of nickel(Ⅱ) and cobalt(Ⅱ) in the raffinates was measured with a Perkin Elmer Model 3100 Atomic Absorption Spectrophotometer (AAS), and that in the organic phase was obtained by mass balance.
3 Results and discussion
3.1 Mechanism of free radical addition reaction
In this study, dialkyl phosphinic acids were mainly synthesized with free radical addition reaction method, in which α-olefin reacted with hypophosphorous acid under the initiation of peroxide or azocompound, which is shown in Fig.1.

Fig.1 Synthetic route for dialkyl phosphinic acids
According to the reaction mechanism (Fig.2), the product contains monoalkyl phosphinic acid and dialkyl phosphinic acid. Meanwhile, olefin itself can polymerize under such condition, so there is always a certain amount of olefin polymers in the product. Therefore, strict control on reaction condition and effective post treatment are essential to improve the yield and purity of the product. For example, orthogonal tests were done to determine the optimal initiator, reaction temperature and reaction time. In order to remove the monoalkyl phosphinic acid byproduct, NaOH solution was chosen to wash the rough product during the post-treatment because the sodium salt of the monoalkyl phosphinic acid was more soluble in aqueous solution due to its less hydrophobic chain. Centrifugation was employed after the step of alkali-washing aiming at removing the polyene byproduct through mechanical separation.
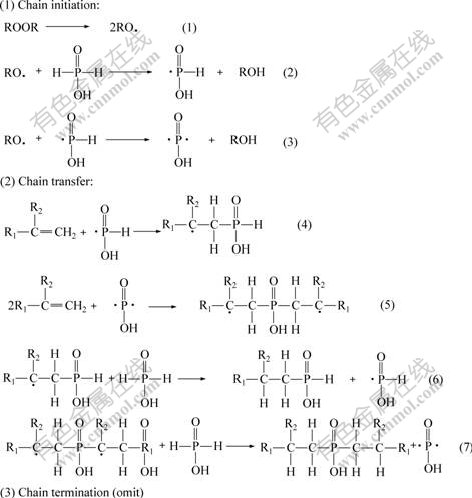
Fig.2 Reaction mechanism for synthesis of dialkyl phosphinic acids
3.2 Synthesis of DHPA
The influence of the type of initiator and reaction temperature on the reaction was investigated, which is shown in Fig.3.
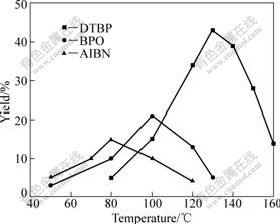
Fig.3 Influence of the type of initiator, reaction temperature on yield of DHPA (t=15 h)
From Fig.3, it can be seen that the optimal reaction temperatures are different when the reaction is initiated by different initiators. As for the initiator of zaodiisobutyronitrile (AIBN), benzoperoxide (BPO), and Di-tert-butyl peroxide (DTBP), the optimal reaction temperatures are 80, 100 and 130 ℃, respectively, during the same reaction time of 15 h. DTBP exhibits high initiation efficiency at about 130 ℃ compared with BPO and AIBN.
When the reaction temperature was controlled at 130 ℃ and DTBP was used as initiator, the influence of reaction time on the reaction was investigated. The result is given in Fig.4(a), which shows that the yield of DHPA increases with the time prolonging and reaches the maximum after 20 h.
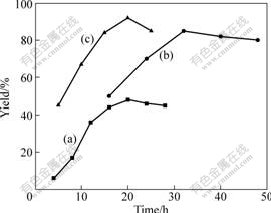
Fig.4 Influence of reaction time on yield of DHPA (a), DTMPPA (b) and DDPA (c) with reaction temperature of 130 ℃ and DTBP as initiator
3.3 Synthesis of DTMPPA
The influence of the type of initiator and reaction temperature on the synthesis of DTMPPA was investigated, which is shown in Fig.5. It can be seen that the regularity is similar to that in the synthesis of DHPA. Nevertheless, the yield of DTMPPA is much higher than that of DHPA under the same condition (initiated by DTBP at 130 ℃).
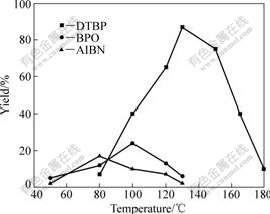
Fig.5 Influence of type of initiator and reaction temperature on yield of DTMPPA (t=15 h)
The influence of reaction time was also investigated when the reaction was initiated by DTBP at 130 ℃. The results are given in Fig.4(b), which shows that the completion of this reaction needs longer time (about 30 h) than that for DHPA.
3.4 Synthesis of DDPA
The influence of the type of initiator and reaction temperature on the synthesis of DDPA was investigated, which is shown in Fig.6. It can be seen the regularity is again similar to that in the synthesis of DHPA and DTMPPA. And the yield of DDPA is slightly higher than that of DTMPPA under the same condition (initiated by DTBP at 130 ℃).
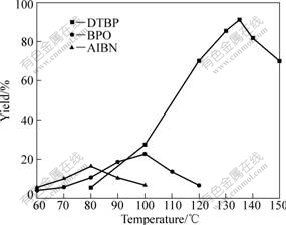
Fig.6 Influence of type of initiator and reaction temperature on yield of DDPA (t=15 h)
Fig.4(c) presents the influence of synthesis reaction initiated by DTBP at 130 ℃. The result also indicates that the yield of DDPA increases with the time prolonging and reaches the maximum after about 20 h.
3.5 Relationship between reaction condition and reactant
In the above-mentioned synthesis reaction, the two P—H bonds in H3PO2 molecule must be broken to form P-containing free radical which is added to the double bond of the α-olefin molecule. Due to the strong attraction from the double-bond oxygen and hydroxyl in H3PO2, the breakage of the two P—H bonds is difficult compared with that in PH3 molecule which is well initiated at about 60-90 ℃[11]. So, the formation of the P-containing free radical with P=O and —OH needs higher energy, i.e. the reaction between α-olefin and H3PO2 requires higher initiating temperature. In this study, it is found that the optimal reaction temperature for DHPA, DTMPPA and DDPA are all about 130 ℃, which indicates that the thorough breakage of the two P—H bonds in H3PO2 molecule occurs at about 130 ℃. Meanwhile, the participation of initiator which can supply relatively stable free radical is necessary to guarantee the going on wheels of the chain reaction as depicted in the mechanism scheme. When the initiator is heated, the —O—O— or —N=N— bond is broken forming free radical to initiate the reaction. The activity of BPO and AIBN is high and the temperatures for their half-life of 10 h are 73 and 65 ℃, respectively. When the reaction occurs at above 90 ℃, BPO and AIBN would decompose rapidly and the reaction cannot proceed consequently. So, when the reaction is initiated by BPO and AIBN, the yields of DHPA, DTMPPA and DDPA are all low. Whereas the activity of DTBP is relatively low and the temperature for its half-life of 10 h is 126 ℃. When the reaction occurs at 100oC below, it is quite slow because the formation speed of free radical is very low. When the reaction occurs at 120-140 ℃, DTBP has moderate rate and this temperature is propitious to the formation of P-containing free radical from H3PO2, which is in accord with the experimental result.
Under the same optimal condition, the yield of DHPA is much lower than that of DTMPPA and DDPA because it is easy for the straight-chain olefin (1-hexene) to self-polymerize through free radical. During the post treatment, much polymer is indeed found and separated through centrifugation. In the case of DTMPPA and DDPA, the steric hindrance caused by the branched chain on the feed olefins impedes the self-polymerization of olefins. Therefore, the yield of DTMPPA and DDPA is very high.
It is noticeable that the synthesis reaction of DHPA and DTMPPA must be carried out in sealed autoclave because the boiling point of 1-hexene and diisobutylene is much lower than 130 ℃, which requires the synthetic equipment to be both aciduric and pressure-proof. Whereas the boiling point of mixed decylene is higher than 130 ℃, the synthesis of DDPA can be performed under normal pressure, which has no special request to the equipment except acid-resistant.
3.6 Extraction
In order to evaluate the separation ability for nickel and cobalt, the influence of equilibrium pH value on extraction percentage of nickel and cobalt was investigated.
Extraction of 0.02 mol/L cobalt(Ⅱ) and nickel(Ⅱ) from an aqueous solution was carried out using excess of sodium salts of DHPA, DTMPPA and DDPA in kerosene (10% of volume fraction). The cobalt and nickel extraction and corresponding equilibrium pH value were measured. The data are plotted in Fig.7. It is found that all the extraction of cobalt with respect to DHPA, DDPA and DTMPPA precedes that of nickel. The difference in pH1/2 value between cobalt and nickel increases from large to small is in the following sequence: DHPA, DDPA and DTMPPA, which indicates that the separation ability for cobalt and nickel ascends from DHPA, DDPA to DTMPPA. The result can be explained by the extracted complex structure of cobalt and nickel. Cobalt tends to take four-coordination with dialkyl phosphinic acid, whereas nickel tends to take six-coordination with dialkyl phosphinic acid. As a result, the influence of steric hindrance of the extractants on the coordination with nickel is higher than that on cobalt. For example, DTMPPA with the highest steric hindrance is most difficult to coordinate with nickel.
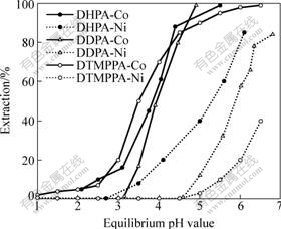
Fig.7 Effect of equilibrium pH on extraction of cobalt and nickel using 10% NaDHPA, NaDDPA and NaDTMPPA
4 Conclusions
1) Three kinds of dialkylphosphinic acids, i.e. DHPA, DTMPPA and DDPA, are synthesized with the method of free radical addition by using α-olefin and hypophosphorous acid as starting materials.
2) The maximum yield of DHPA, DTMPPA and DDPA can all be achieved at about 130 ℃ under the initiation of di-tert-butyl peroxide (DTBP) during proper reaction time.
3) This synthesis method has much practical significance because it avoids the use of highly toxic PH3, and its synthetic route and post-treatment is simple. Especially for DDPA, the feed olefin is the byproduct of petrochemical industry, and its synthesis need not be carried out in sealed vessel because of the higher boiling point of the mixed decylene.
4) The separation ability for nickel and cobalt of DHPA, DTMPPA and DDPA improves with the increase of steric hindrance of the alkyl chain, i.e. separation coefficient β(DHPA)<β(DDPA)<β(DTMPPA).
References
[1] LEE M S, AHN J G, LEE E C. Solvent extraction separation of indium and gallium from sulphate solutions using D2EHPA [J]. Hydrometallurgy, 2002, 63(3): 269-276.
[2] LEE G S, UCHIKOSHI M, MIMURA K, ISSHIKI M. Separation of major impurities Ce, Pr, Nd, Sm, Al, Ca, Fe, and Zn from La using bis(2-ethylhexyl)phosphoric acid (D2EHPA)-impregnated resin in a hydrochloric acid medium [J]. Separation and Purification Technology, 2010, 71(2): 186-191.
[3] LUO Lin, WEI Jian-hong, WU Gen-yi, TOYOHISA F, ATSUSHI S. Extraction studies of cobalt (Ⅱ) and nickel(Ⅱ) from chloride solution using PC88A [J]. Transactions of Nonferrous Metals Society of China, 2006, 16(3): 687-692.
[4] PRESTON J S. Solvent extraction of cobalt and nickel by organophosphorus acids I. Comparison of phosphoric, phosphonic and phosphonic acid systems [J]. Hydrometallurgy, 1982, 9(2): 115-133.
[5] FLETT D S. Solvent extraction in hydrometallurgy: The role of organophosphorus extractants [J]. Journal of Organometallic Chemistry, 2005, 690(10): 2426-2438.
[6] NISHIHAMA S, SAKAGUCHI N, HIRAI T, KOMASAWA I. Extraction and separation of rare earth metals using microcapsules containing bis(2-ethylhexyl)phosphinic acid [J]. Hydrometallurgy, 2002, 64(1): 35-42.
[7] GUPTA B, MUDHAR N, SINGH I. Separations and recovery of indium and gallium using bis(2,4,4-trimethylpentyl)phosphinic acid (Cyanex 272) [J]. Separation and Purification Technology, 2007, 57(2): 294-303.
[8] SWAIN B, JEONG J, LEE J, LEE G H. Separation of cobalt and lithium from mixed sulphate solution using Na-Cyanex 272[J]. Hydrometallurgy, 2006, 84(3/4): 130-138.
[9] GANDHI M N, DEORKAR N V, KHOPKAR S M. Solvent extraction separation of cobalt(II) from nickel and other metals with cyanex 272 [J]. Talanta, 1993, 40(10): 1535-1539.
[10] SALEH M I, BARI M F, SAAD B. Solvent extraction of lanthanum(Ⅲ) from acidic nitrate-acetato medium by Cyanex 272 in toluene [J]. Hydrometallurgy, 2002, 63(1): 75-84.
[11] RORBERTSON A J, Di-2, 4, 4-trimethylpentylphosphinic acid and its preparation: US Patent, 4374780[P]. 1983-02-22.
[12] ROBERT H W, LYLE A. H., Di-n-alkylphosphine oxides. I. Synthesis [J]. Journal of the American Chemical Society, 1952, 74(21): 5418-5420.
[13] ROBERT H W, LYLE A H, Disubstituted phosphine oxides and disubstituted phosphinic acids. II. The Di-n-akyl series [J]. Journal of the American Chemical Society, 1955, 77(12): 3411-3412.
[14] WO S, MONROE T N J, CAMPO F D, PLEASANT M S C. Process for the preparation of highly purified dialkyl phosphinic acids: US Patent, 7049463 B2[P]. 2006-05-23.
[15] SUGIYA M, WATANABE T, TAKEUCHI K. Bis(1,1,3,3- tetramethylbutyl) phosphinic acid compound, a production method thereof and an extracting agent: US Patent, 5925784[P]. 1999-07-20.
_________________________
Foundation item: Projects(2007CB613506) supported by the National Basic Research Program of China; Projects(50674060, 50734005) supported by the National Natural Science Foundation of China
Corresponding author: XU Sheng-ming; Tel: 86-10-62773585; E-mail: smxu@tsinghua.edu.cn
(Edited by CHEN Can-hua)