
J. Cent. South Univ. (2021) 28: 2003-2009
DOI: https://doi.org/10.1007/s11771-021-4667-0

Separation of potassium from sodium in alkaline solution by solvent extraction with 4-tert-butyl-2-(α-methylbenzyl) phenol
XING Peng(邢鹏)1, WANG Cheng-yan(王成彦)1, 2, CHEN Yong-qiang(陈永强)1, MA Bao-zhong(马保中)1
1. School of Metallurgical and Ecological Engineering, University of Science and Technology Beijing, Beijing 100083, China;
2. School of Metallurgical and Chemical Engineering, Jiangxi University of Science and Technology, Ganzhou 341000, China
Central South University Press and Springer-Verlag GmbH Germany, part of Springer Nature 2021
Abstract: This work investigated the separation of potassium from sodium in alkaline solution using substituted phenol-based extractants. Superior potassium extraction was achieved with 4-tert-butyl-2-(α-methylbenzyl) phenol (t-BAMBP) than 4-sec-butyl-2-(α-methylbenzyl) phenol (BAMBP). The optimum conditions for the extraction were 1 mol/L t-BAMBP, 3:1 volumetric phase ratio (O/A), and two extraction stages. After cross-current extraction, the extraction ratio of potassium reached 90.8%. After scrubbing with deionised water at phase ratio of 4:1 and scrubbing stage of 4, a sodium scrubbing efficiency of 88.2% was obtained. After stripping using 1 mol/L H2SO4 at phase ratio of 3:1, the stripping efficiency of potassium reached 94.2%. The potassium/sodium (K/Na) concentration ratio increased 14.3 times from 0.15 in the feed solution to 2.3 in the stripping solution. The efficient separation of potassium from sodium in alkaline solution was achieved via solvent extraction with t-BAMBP.
Key words: 4-tert-butyl-2-(α-methylbenzyl) phenol (t-BAMBP); potassium; sodium; solvent extraction; separation
Cite this article as: XING Peng, WANG Cheng-yan, CHEN Yong-qiang, MA Bao-zhong. Separation of potassium from sodium in alkaline solution by solvent extraction with 4-tert-butyl-2-(α-methylbenzyl) phenol [J]. Journal of Central South University, 2021, 28(7): 2003-2009. DOI: https://doi.org/10.1007/s11771-021-4667-0.
1 Introduction
Potassium is one of the three essential nutrients for crop growth, making potassium fertilisers irreplaceable in high-efficiency agricultural production [1]. The two primary sources of potassium are water-soluble and non-water-soluble potassium minerals. Water-soluble potassium minerals include sylvite (potassium chloride), carnallite, polyhalite and arcanite. These are often found in salt lake brine, underground brine, and seawater and are accompanied by other elements, including magnesium, sodium, lithium, rubidium, and caesium. Non-water-soluble potassium minerals are primarily composed of potassium-containing aluminosilicates, such as potassium feldspar, alunite, and glauconite [2]. Water-soluble potassium minerals are currently the main source of the global potash supply. However, the water-soluble potassium resources are severely deficient while the non-water-soluble potassium resources are rich in some countries, such as India and China. The ever-growing demand for potassium fertiliser has rendered the exploitation of non-water-soluble potassium mines critical importance [3, 4].
In recent years, there has been an increasing focus on the extraction of potassium from non-water-soluble potassium minerals. Multiple studies have shown that hydrothermal leaching using sodium hydroxide is particularly effective for the decomposition of potassium feldspar [5-8]. However, the separation of sodium and potassium from an alkaline leaching solution is challenging owing to similarities between their properties. MA et al [6] proposed to separate sodium and potassium by carbonisation crystallisation. However, as the solubility of sodium carbonate is much lower than that of potassium carbonate, it is difficult to crystallise potassium. The extraction and separation of potassium from aqueous solutions by ion exchange and adsorption has also been reported [9-12]. However, these two methods are generally only applicable to solutions with low potassium concentrations. While relatively few studies describe the use of solvent extraction reagents for the extraction and separation of potassium, it is a promising method due to its high extraction capacity. The solvent extraction of potassium using calixarene crown ethers has been reported [13-15] to show good selectivity for potassium in picric acid; unfortunately, this method is limited by the high cost and toxicity of the reagents. In contrast, substituted phenols are less toxic, and have shown promising results for extracting rubidium and caesium from solution [16, 17]. However, the use of substituted phenols for the extraction and separation of potassium and sodium has yet to be reported.
In this work, 4-tert-butyl-2-(α-methylbenzyl) phenol (t-BAMBP) was used for the separation of potassium from sodium in a Na–K alkaline solution resembling the leaching liquor of potassium feldspar.Factors affecting the extraction, scrubbing, and stripping were investigated. To assess its extraction performance, t-BAMBP was compared to 4-sec-butyl-2-(α-methylbenzyl) phenol (BAMBP).
2 Materials and methods
2.1 Materials
To simulate a potassium feldspar leaching solution (K 11.7 g/L, Na 75.5 g/L, OH- 61 g/L), a synthetic K–Na alkaline solution was prepared by dissolving potassium hydroxide (analytical reagent (AR) grade) and sodium hydroxide (AR grade) in deionised water. The active component of the extractant t-BAMBP was above 90%. Xylene was used as the diluent. Stripping solutions were prepared by diluting 98% sulphuric acid in deionised water.
2.2 Procedures and methods
The K/Na extraction separation tests were conducted in a separatory funnel and mechanically shaken. Initial experiments examining the effect of time on extraction separation indicated that 2 min was sufficient to reach extraction equilibrium, and this was used throughout this work. Unless otherwise stated, all experiments were performed at room temperature ((25±1) °C) with an organic/aqueous (O/A) phase ratio of 1:1. After phase disengagement, the aqueous and organic phases were separated. The loaded organic (LO) phase was then stripped with 2 mol/L H2SO4. All aqueous samples were analyzed using inductively coupled plasma optical emission spectrometry (ICP-OES). The separation factor, β, was calculated using Eq. (1):
(1)
where [K]org, [Na]org, [K]aq, and [Na]aq represent potassium and sodium concentrations in organic and aqueous phases, respectively.
3 Results and discussion
3.1 Extraction of potassium
3.1.1 Comparison of t-BAMBP against BAMBP
In order to compare the extraction performance of t-BAMBP and BAMBP, the K/Na separation was carried out from the alkaline solution using 1 mol/L t-BAMBP or BAMBP, respectively. The extraction ratio obtained using t-BAMBP was higher than that for BAMBP, indicating that t-BAMBP is a more effective K extractant than BAMBP (Figure 1).
The substituted phenol-based extraction of alkali metal ions is proposed to occur via cation exchange between the hydrogen ion of the phenolic hydroxyl group and the alkali metal ions [17]. Substituted phenol based-extractants interact with alkali metals in the order of Cs>Rb>K>Na>Li [17], making them useful reagents for isolating potassium in a K/Na solution.
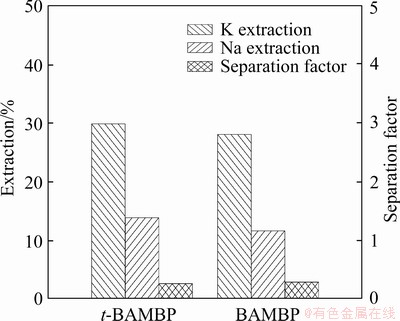
Figure 1 K/Na extraction and separation by t-BAMBP/BAMBP
The Fourier transform infrared (FTIR) spectrum of the pre-extraction t-BAMBP organic phase shows four distinct vibration bands: νO—H (3550 cm-1), ν=C—H (3050 cm-1), ν—C—H(2950 cm-1), and δCH2 (1460 cm-1) (Figure 2). In comparison, the intensity of the νO—H and ν=C—H bands of BAMBP was notably lower. Following the extraction of potassium, there was an observable shift and intensity decrease of the absorption peak of the phenolic hydroxyl group. Based on the changes of the νO—H band following extraction, it could be concluded that t-BAMBP was better able to extract potassium from the alkaline solution than BAMBP. A comparison of the molecular structures of the two extractants (Figure 3), suggests that this difference in extraction performance arises from the superior ability of the tert-butyl group to ionise the phenolic hydroxyl.
3.1.2 Effect of alkalinity
Previous studies have shown that the extraction of alkali metals is affected by the alkalinity of the solution [18]. The alkaline condition is favorable for the ionization of the phenolic hydroxyl group.In this work, solution alkalinity was varied to explore its effect on the K/Na separation using t-BAMBP. Using a constant t-BAMBP concentration (1.0 mol/L), the alkalinity of the solution was adjusted with H2SO4 (Figure 4). The extraction efficiency of potassium decreased from 29.9% to 28.7%, whereas the separation factor increased from 2.66 to 3.72 with a decrease in the initial OH- concentration from 60.9 to 28.0 g/L. Overall, the effect of alkalinity on the extraction efficiency and separation factor was small. Therefore, the alkalinity of the K-Na alkaline solution was not adjusted in subsequent extraction tests.
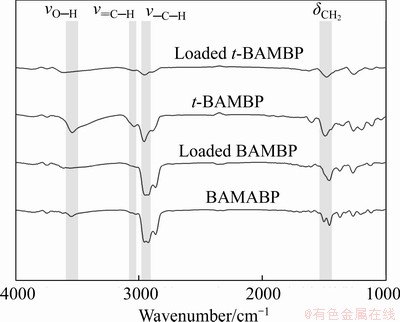
Figure 2 FTIR spectra of pre-extraction and loaded organic phases
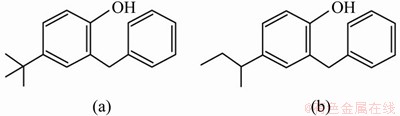
Figure 3 Structures of t-BAMBP (a) and BAMBP (b)
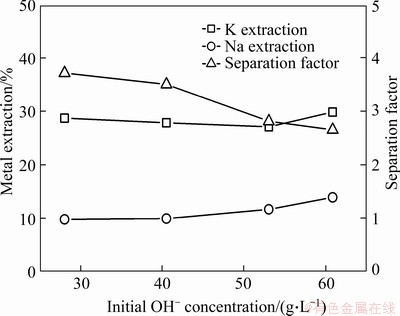
Figure 4 Effect of solution alkalinity on extraction and separation of potassium and sodium
3.1.3 Effect of t-BAMBP concentration
A stoichiometric quantity of extractant is often insufficient to obtain a high metal extraction ratio [19-21]. To investigate the impact of extractant concentration on the extraction and separation of potassium and sodium, the t-BAMBP concentration (moles of t-BAMBP/volume of the organic phase) was varied from 0.5 to 2.0 mol/L. Increasing the t-BAMBP concentration led to an increase in the extraction efficiencies of both potassium and sodium with the maximum value for the separation factor occurring at a t-BAMBP concentration of 1.0 mol/L (Figure 5). In particular, when the t-BAMBP concentration was increased to 1.5 mol/L, phase disengagement deteriorated due to an increase in the viscosity of the organic phase, and thus the optimum t-BAMBP concentration was determined to be 1.0 mol/L.
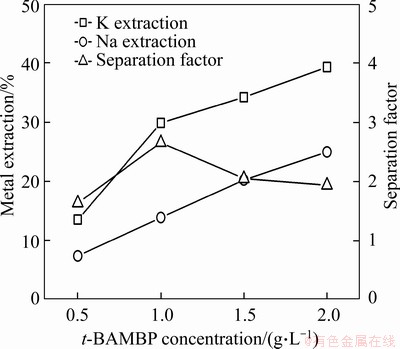
Figure 5 Effect of t-BAMBP concentration on extraction and separation of potassium and sodium
3.1.4 Effect of temperature
Previous studies have shown that temperature affects the solvent extraction of metals [22-24]. To investigate this effect, the separation factor and extraction ratio of potassium and potassium were determined over a temperature range of 25-55 °C. The separation factor increased with increasing temperature throughout the temperature range (Figure 6). The extraction ratio of potassium and sodium increased slightly with increasing temperature up to 35 °C and then decreased. The high temperature increases the volatilisation of the organic phase, which is unfavourable. Therefore, room temperature was preferable for the extraction.
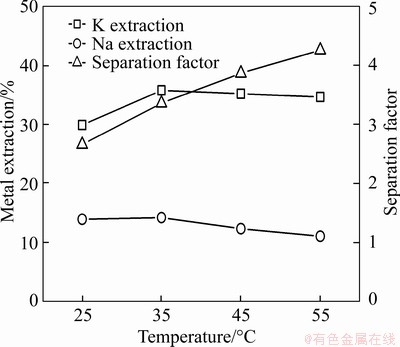
Figure 6 Effect of temperature on extraction and separation of potassium and sodium
3.1.5 Effect of phase ratio
The phase ratio (O/A) was varied in the range of 1:1-4:1 to investigate its impact on the extraction and separation of potassium and sodium. With increasing phase ratios, the extraction efficiencies of potassium and sodium substantially increased, while the separation factor increased only slightly (Figure 7). However, when the phase ratio was increased to 4:1, phase disengagement deteriorated. Therefore, the optimum phase ratio was determined to be 3:1.
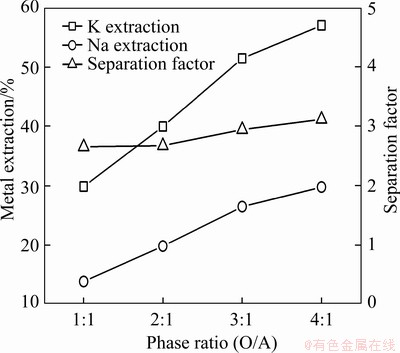
Figure 7 Effect of phase ratio (O/A) on extraction and separation of potassium and sodium
3.1.6 Cross-current extraction
As single-stage extraction is insufficient for complete recovery of potassium, cross-current extraction tests were conducted at various extraction stages (1-3) using the optimal condition determined above (t-BAMBP 1 mol/L, 3:1 phase ratio). As shown in Figure 8, increasing the extraction stages was beneficial to the extraction of potassium. However, the increase in sodium extraction was even greater than potassium extraction when the extraction stage was increased to 3. The maximum separation factor was obtained at the 2nd extraction stage, which was determined to be the optimum condition for these extractions. After a two-stage cross-current extraction, the extraction ratio of potassium reached 90.8%.
3.2 Scrubbing of sodium
In the two-stage cross-current extraction, approximately 40% of the sodium in the K-Na solution was co-extracted. To separate the remaining sodium from the potassium, the LO phase required scrubbing. As potassium is easily scrubbed by acid, deionised water was chosen as the sodium scrubbing agent. The effect of the phase ratio on scrubbing was investigated over an O/A range of 1:1-8:1. As shown in Figure 9, Na scrubbing efficiency was found to decrease with increasing phase ratio. Potassium scrubbing also decreased with increasing phase ratio up to 4:1 but remained constant with further increases. The optimum phase ratio for scrubbing was therefore determined to be 4:1 to maximise sodium scrubbing while minimising potassium scrubbing.
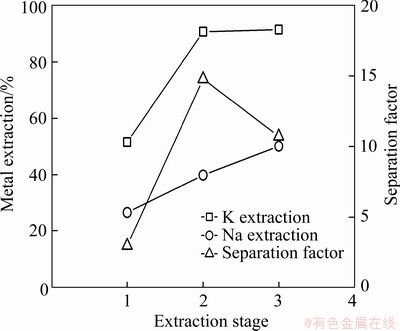
Figure 8 Effect of extraction stage on extraction and separation of potassium and sodium
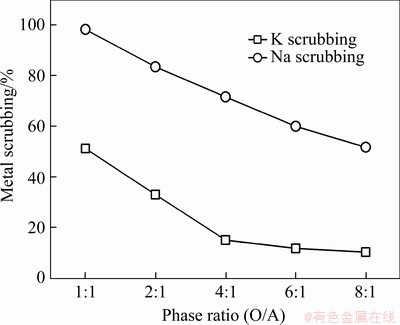
Figure 9 Effect of phase ratio (O/A) on scrubbing of potassium and sodium
In order to determine the number of scrubbing stages, a scrubbing isotherm was prepared (Figure 10). The theoretical scrubbing stages for sodium removal were determined to be 4. On the basis of the sodium scrubbing isotherm, a counter-current scrubbing was conducted and decreased the sodium concentration in the LO phase to 0.6 g/L, corresponding to a sodium scrubbing efficiency of 88.2%.
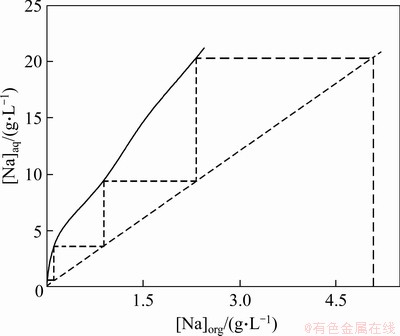
Figure 10 McCabe-Thiele diagram for sodium scrubbing by deionised water
3.3 Stripping of potassium
After the scrubbing of sodium, the potassium in the LO phase was stripped by sulphuric acid solution. To investigate the effects of H2SO4 concentration on potassium stripping, stripping tests were performed using a phase ratio (O/A) of 3:1 and H2SO4 concentrations from 0.1 to 1.5 mol/L. The stripping efficiency of potassium increased from 40.6% to 94.2% with an increase in the H2SO4 concentration from 0.1 to 1.0 g/L (Figure 11). The optimum H2SO4 concentration for potassium stripping was therefore determined to be 1 mol/L.
Following the extraction, scrubbing, and stripping tests, a total recovery of 77% potassium was obtained, with the concentration ratio of potassium to sodium (K/Na) increasing by 14.3 times from 0.15 in the feed solution to 2.3 in the stripping solution. As the solubility of potassium sulphate is less than that of sodium sulphate, the potassium sulphate can be isolated via crystallisation from the stripping solution.
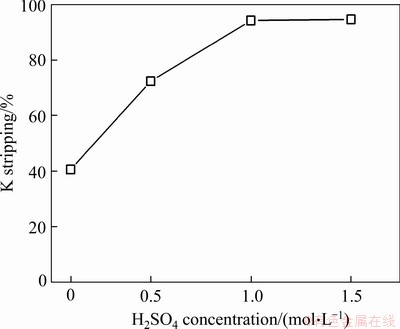
Figure 11 Effect of H2SO4 concentration on stripping of K
4 Conclusions
K/Na separation in alkaline solution was successfully achieved by solvent extraction using a substituted phenol-based extractant in xylene. This work showed that t-BAMBP outperformed BAMBP in potassium extraction. Neither the alkalinity nor the temperature of the solution had a strong effect on the extraction of potassium. The optimum conditions for the extraction were: 1 mol/L t-BAMBP, 3:1 volumetric phase ratio (O/A), and two extraction stages. After a two-stage cross-current extraction, the extraction ratio of potassium reached 90.8%. After a four-stage counter-current scrubbing with deionised water at a phase ratio of 4:1, a sodium scrubbing efficiency of 88.2% was obtained. A 94.2% potassium stripping efficiency was accomplished with 1 mol/L H2SO4 at phase ratio (O/A) of 3:1. Under these optimised conditions, the total recovery of potassium was 77% and the potassium/sodium (K/Na) concentration ratio increased by 14.3 times from 0.15 in the feed liquid to 2.3 in the stripping solution. Solvent extraction using t-BAMBP is therefore an effective method for the separation of potassium from sodium in an aqueous solution with high alkalinity and sodium concentration.
Contributors
The overarching research goals were developed by WANG Cheng-yan and MA Bao-zhong. XING Peng performed the experiments, collected and analyzed the data. The research activity planning and execution were managed by CHEN Yong-qiang. The initial draft of the manuscript was written by XING Peng. WANG Cheng-yan and MA Bao-zhong reviewed and edited the manuscript.
Conflict of interest
XING Peng, WANG Cheng-yan, CHEN Yong-qiang, and MA Bao-zhong declare that they have no conflict of interest.
References
[1] LANZERSTORFER C. Potential of industrial de-dusting residues as a source of potassium for fertilizer production–A mini review [J]. Resources, Conservation and Recycling, 2019, 143: 68-76. DOI: 10.1016/j.resconrec.2018.12.013.
[2] SINGH Y P, TANVAR H, KUMAR G, DHAWAN N. Investigation of planetary ball milling of sericite for potash recovery [J]. Powder Technology, 2019, 351: 115-121. DOI: 10.1016/j.powtec.2019.04.013.
[3] JENA S K, DHAWAN N, RAO D S, MISRA P K, MISHRA B K, DAS B. Studies on extraction of potassium values from nepheline syenite [J]. International Journal of Mineral Processing, 2014, 133: 13-22. DOI: 10.1016/j.minpro.2014. 09.006.
[4] ZHOU Jun-feng, MA Jia-yu, QIN Yuan-hang, WU Zai-kun, YANG Li, LYU Ren-liang, WANG Tie-lin, WANG Wei-guo, WANG Cun-wen. Leaching and concentration of P and K from P-K associated ore by using a sequential leaching process with leachate recycling [J]. Separation and Purification Technology, 2019, 221: 195-200. DOI: 10.1016/j.seppur.2019.03.099
[5] SU Shuang-qing, MA Hong-wen, CHUAN Xiu-yun. Hydrothermal decomposition of K-feldspar in KOH- NaOH-H2O medium [J]. Hydrometallurgy, 2015, 156: 47-52. DOI: 10.1016/j.hydromet.2015.05.014.
[6] MA Xi, YANG Jing, MA Hong-wen, LIU Chang-jiang. Hydrothermal extraction of potassium from potassic quartz syenite and preparation of aluminum hydroxide [J]. International Journal of Mineral Processing, 2016, 147: 10-17. DOI: 10.1016/j.minpro.2015.12.007.
[7] WANG Zhao, ZHANG Quan-you, YAO Ying, JIA Yong-zhong, XIE Bing-jun. The extraction of potassium from K-feldspar ore by low temperature molten salt method [J]. Chinese Journal of Chemical Engineering, 2018, 26(1): 845-851. DOI: 10.1016/j.cjche.2017.09.006.
[8] XING Peng, WANG Cheng-yan, MA Bao-zhong, WANG Ling, ZHANG Wen-juan, CHEN Yong-qiang. Rubidium and potassium extraction from granitic rubidium ore: Process optimization and mechanism study [J]. ACS Sustainable Chemistry & Engineering, 2018, 6: 4922-4930. DOI: 10.1021/acssuschemeng.7b04445.
[9] KOMATSU Y, FUJIKI Y, SASAKI T. Ion-exchange separation of sodium and potassium ions on dihydrogen tetratitanate hydrate fibers at various temperatures [J]. Solvent Extraction and Ion Exchange, 1993, 11: 159-169. DOI: 10.1080/07366299308918150.
[10] YUAN Jun-sheng, ZHAO Ying-ying, LI Qing-hui, JI Zhi-yong, GUO Xiao-fu. Preparation of potassium ionic sieve membrane and its application on extracting potash from seawater [J]. Separation and Purification Technology, 2012, 99: 55-60. DOI: 10.1016/j.seppur.2012.08.020.
[11] JIN Nan, MENG Chun-xia, HOU Jin. Preparation and characterization of merlinoite for potassium extraction from seawater [J]. Journal of Industrial and Engineering Chemistry, 2014, 20: 1227-1230. DOI: 10.1016/j.jiec.2013.08.003.
[12] AN Sha-sha, LIU Jie, WANG Ji-hong, WANG Mu-cun, JI Zhi-yong, QI Shan-shan, YUAN Jun-sheng. Synthesis and characterization of a plat sheet potassium ion sieve membrane and its performances for separation potassium [J]. Separation and Purification Technology, 2018, 212: 834-842. DOI: 10.1016/j.seppur.2018.11.079.
[13] SATA T, TANIMOTO M, KAWAMURA K, MATSUSAKI K. Electrodialytic separation of potassium ions from sodium ions in the presence of crown ether using a cation-exchange membrane [J]. Colloid and Polymer Science, 278: 57-60. DOI: 10.1007/s003960050008.
[14] AGNIHOTRI P, SURESH E, GANGULY B, PAUL P, GHOSH P K. Study of the competitive binding of mixed alkali and alkaline earth metal ions with dibenzo-30- crown-10 [J]. Polyhedron, 2005, 24: 1023-1032. DOI: 10.1016/j.poly.2005.01.022.
[15] LI Y, HUSZTHY P, MOCZAR I, SZEMENYEI B, KUNSAGI-MATE S. Solvent effect on the complex formation of a crown ether derivative with sodium and potassium ions. Thermodynamic background of selectivity [J]. Chemical Physics Letters, 2012, 556: 94-97. DOI: 10.1016/j.cplett.2012.11.056.
[16] LI Z, PRANOLO Y, ZHU Z, CHENG C Y. Solvent extraction of cesium and rubidium from brine solutions using 4-tert-butyl-2-(α-methylbenzyl)-phenol [J]. Hydrometallurgy, 2017, 171: 1-7. DOI: 10.1016/j.hydromet.2017.03.007.
[17] WANG Jia-wei, CHE De-hua, QIN, Wei. Extraction of rubidium by t-BAMBP in cyclohexane [J]. Chinese Journal of Chemical Engineering, 2015, 23: 1110-1113. DOI: 10.1016/j.cjche.2015.04.005.
[18] LIU Shi-ming, LIU He-hui, HUANG Yun-jing, YANG Wei-jun. Solvent extraction of rubidium and cesium from salt lake brine with t-BAMBP-kerosene solution [J]. Transactions of Nonferrous Metals Society of China, 2015, 25: 329-334. DOI: 10.1016/S1003-6326(15)63608-1.
[19] WEJMAN-GIBAS K, PILSNIAK-RABIEGA M, OCHROMOWICZ K. Solvent extraction of zinc(II) from ammonia leaching solution by LIX 54-100, LIX 84 I and TOA [J]. Physicochemical Problems of Mineral Processing, 2017, 53(1): 202-211. DOI: 10.5277/ppmp170117.
[20] WANG Zhi-jian, DING Feng-hua, ZHAN Jing, ZHANG Chuan-fu. Solvent extraction mechanism and precipitation stripping of bismuth (III) in hydrochloric acid medium by tributyl phosphate [J]. Journal of Central South University, 2016, 23: 3085-3091. DOI: 10.1007/s11771-016-3373-9.
[21] ZHANG Xue-kai, ZHOU Kang-gen, CHEN Wei, LEI Qing-yuan, HUANG Ying, PENG Chang-hong. Recovery of iron and rare earth elements from red mud through an acid leaching-stepwise extraction approach [J]. Journal of Central South University, 2019, 26: 458-466. DOI: https://doi.org/10.1007/s11771-019-4018-6.
[22] XING Peng, WANG Cheng-yan, JU Zhong-jun, LI Dun-fang, YIN Fei, CHEN Yong-qiang, XU Sheng-ming, YANG Yong-qiang. Cobalt separation from nickel in sulfate aqueous solution by a new extractant: Di-decylphosphinic acid (DDPA) [J]. Hydrometallurgy, 2012, 113-114: 86-90. DOI:10.1016/j.hydromet.2011.12.005.
[23] BALESINI-AGHDAM A A, YOOZBASHIZADEH H, MOGHADDAM J. Direct hydrometallurgical separation of Zn(II) from brine leaching solution of zinc filter cake by simple solvent extraction process [J]. Physicochemical Problems of Mineral Processing, 2019, 55(3): 667-678. DOI: 10.5277/ppmp18179.
[24] SANGHAMITRA P, NIHARBALA D, SUJATA M. Separation of copper and iron from chloride media using Cyanex 921 in kerosene[J]. Journal of Central South University, 2014, 21: 1752-1755. DOI: 10.1007/s11771-014- 2120-3.
(Edited by YANG Hua)
中文导读
4-叔丁基-2-(α-甲基苄基)苯酚萃取分离碱液中的钾和钠
摘要:本文研究了在碱性溶液中用取代酚类萃取剂分离钾和钠。4-叔丁基-2-(α-甲基苄基)苯酚(t-BAMBP)比4-仲丁基-2-(α-甲基苄基)苯酚(BAMBP)具有更好的提钾效果。最佳萃取条件为:1 mol/L t-BAMBP,相比(O/A)3:1,萃取级数两级。通过错流萃取,钾的萃取率达90.8%。用去离子水按4:1的相比进行4级洗涤,钠的洗涤率为88.2%。用1 mol/L H2SO4按3:1的相比反萃,钾的反萃率达94.2%。钾/钠(K/Na)浓度比从料液中的0.15增加到了反萃液中的2.3,增加了14.3倍。使用t-BAMBP进行溶剂萃取,可实现碱液中钾和钠的高效分离。
关键词:t-BAMBP;钾;钠;溶剂萃取;分离
Foundation item: Projects(52034002, U1802253) supported by the National Natural Science Foundation of China; Project(2019YFC1908401) supported by the National Technology Support Project of China
Received date: 2019-09-24; Accepted date: 2020-12-20
Corresponding author: WANG Cheng-yan, PhD, Professor; Tel: +86-10-62332271; E-mail: chywang@yeah.net; ORCID: https://orcid. org/0000-0003-3982-6208; MA Bao-zhong, PhD, Professor; Tel: +86-10-62333170; E-mail: bzhma_ustb@ yeah.net; ORCID: https://orcid.org/0000-0003-3907-9097