Trans. Nonferrous Met. Soc. China 23(2013) 2094-2099
Anti-oxidation properties of ZrB2 modified silicon-based multilayer coating for carbon/carbon composites at high temperatures
He-jun LI, Xi-yuan YAO, Yu-lei ZHANG, Dong-jia YAO, Shao-long WANG
State Key Laboratory of Solidification Processing, Northwestern Polytechnical University, Xi’an 710072, China
Received 30 January 2013; accepted 6 May 2013
Abstract: To improve the anti-oxidation ability of silicon-based coating for carbon/carbon (C/C) composites at high temperatures, a ZrB2 modified silicon-based multilayer oxidation protective coating was prepared by pack cementation. The phase composition, microstructure and oxidation resistance at 1773, 1873 and 1953 K in air were investigated. The prepared coating exhibits dense structure and good oxidation protective ability. Due to the formation of stable ZrSiO4-SiO2 compound, the coating can effectively protect C/C composites from oxidation at 1773 K for more than 550 h. The anti-oxidation performance decreases with the increase of oxidation temperature. The mass loss of coated sample is 2.44% after oxidation at 1953 K for 50 h, which is attributed to the decomposition of ZrSiO4 and the volatilization of SiO2 protection layer.
Key words: C/C composites; coating; ZrB2; anti-oxidation properties
1 Introduction
Carbon/carbon (C/C) composites are attractive materials for thermo-structural components in engines and aerospace vehicles [1,2]. However, the oxidation problem at temperatures above 773 K limits their application in oxygen-containing atmosphere [3,4].
Silicon-based ceramic coatings, especially MoSi2–SiC coating, have been widely used for oxidation protection for C/C composites at high temperatures [5-7]. The formation of SiO2 glassy film on the coating surface has very low oxygen permeability (10-13 g/(cm·s) at 1473 K and 10-11 g/(cm·s) at 2473 K) [8], which can prevent oxygen from diffusing into C/C composites [9,10]. However, with oxidation time increasing or oxidation temperature rising, the MoSi2-SiC coating exhibits poor oxidation resistance because of the formation of the holes and bubbles in SiO2 glassy film. Moreover, the unitary SiO2 glass would volatilize slowly, resulting in the consumption of coating materials [11-14]. Therefore, the formation of a stable vitreous film without holes and bubbles throughout the oxidation process is essential. To solve this problem, ZrB2 ceramic is introduced to silicon-based coating.
ZrB2 has an extremely high melting point (3313 K), low coefficient of thermal expansion (5.5×10-6 K-1), high thermal shock resistance, retention of strength at elevated temperatures and stability in extreme environment [15]. ZrB2-based ceramics are considered the potential candidate materials used in hypersonic vehicles and reusable atmospheric re-entry vehicle [16,17]. ZrO2 can be formed for the oxidation of ZrB2 in air at high temperatures. It reacts with SiO2 to form thermally stable ZrSiO4 during the oxidation of silicon-based coating [18-20]. Adding ZrB2 to MoSi2-SiC coating can be expected to improve the stability of SiO2 glass and improve the service time of MoSi2-SiC coating at high temperatures.
Pack cementation method is an effective technique to prepare dense silicon-based anti-oxidation coatings, which can provide strong interfacial bonding in a simple and rapid way [21,22]. SiC ceramic is widely used as an internal buffer layer because of its good anti-oxidation property and compatibility with C/C composites. In addition, to solve the mismatch of thermal expansion coefficient between SiC inner layer and ZrB2 modified MoSi2-SiC layer, a ZrB2 modified SiC-Si coating is designed as middle layer, which is expected to decrease the formation of penetrable cracks in coating. A scheme of multilayer coating structure is designed as shown in Fig. 1.
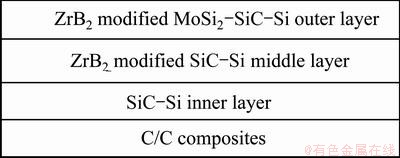
Fig. 1 Designed schematic structure of silicon-based multilayer coating for C/C composites
The aim of this work is to develop a ZrB2 modified silicon-based multilayer coating, which is expected to improve the oxidation resistance of silicon-based coating for C/C composites. The crystalline structure and morphology of the coating were studied. The oxidation resistance performances at 1773, 1873 and 1953 K were investigated. The oxidation mechanism of prepared coating was also discussed.
2 Experimental
Two dimensional C/C composites with density of 1.70 g/cm3 were used as substrates. The composites were mechanically abraded using 320 and 800 grit SiC papers, then cleaned with distilled water and dried at 373 K. The first step was to prepare SiC bonding layer on C/C composites by pack cementation with Si, graphite and Al2O3 powders at 1973-2273 K for 2 h in argon protective atmosphere [11]. The SiC-Si-ZrB2 middle layer was prepared at 2073–2373 K for 2 h in argon protective atmosphere, using Si, B2O3, graphite and ZrB2. The preparation details were reported in Ref. [23]. The outer coating was prepared at 2173–2473 K for 2 h with the powder composition of 40%-65% Si, 20%-35% MoSi2, 8%-15% graphite and 20%-30% ZrB2 in mass fraction.
A MoSi2 resistance heated furnace was used for isothermal oxidation test. The samples were exposed to air at 1773, 1873 and 1953 K. During oxidation, the samples were weighed at room temperature by an electronic precision balance with a sensitivity of ±0.1 mg. Mass loss Δm% was calculated by the following equation:
(1)
where m0 and m1 are the mass of coated samples before and after oxidation, respectively.
The crystalline structure and morphology of the as-obtained multilayer coating and oxidized coating were characterized using X-ray diffraction (XRD, X’ Pert PRO) and scanning electron microscopy (SEM, JSM-6430) equipped with energy dispersive spectroscopy (EDS).
3 Results and discussion
3.1 Microstructure of multilayer coating
The XRD pattern of obtained coating surface by pack cementation (Fig. 2(a)) shows that the coating is composed of SiC, Si, MoSi2 and ZrB2. The backscattering electron image of coating surface (Fig. 2(b)) indicates the formation of a dense structure. The prepared coating has three kinds of crystalline particles. The XRD and EDS analyses show that the white phase is a mixture of MoSi2 and ZrB2. The gray black and gray phases are SiC and Si, respectively.
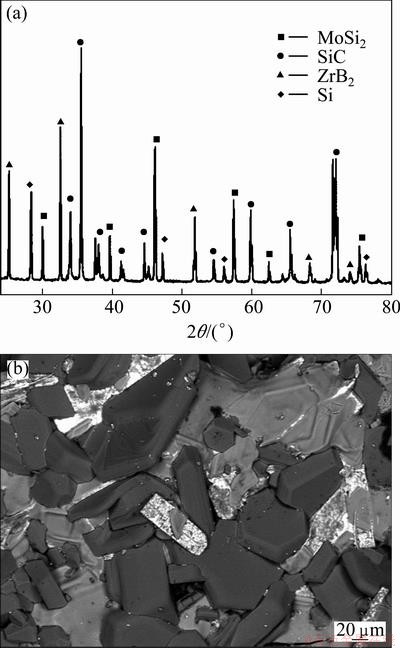
Fig. 2 XRD pattern (a) and SEM image (b) of multilayer coating obtained by pack cementation
Figure 3 shows the cross-section SEM image of the as-received multilayer coating. The coating is about 120 μm in thickness. No penetration cracks or large holes can be observed in the coating. In addition, no obvious interface can be found between layers, which illustrates the good bonding. Therefore, the dense ZrB2 modified silicon-based multilayer coating is obtained by pack cementation.
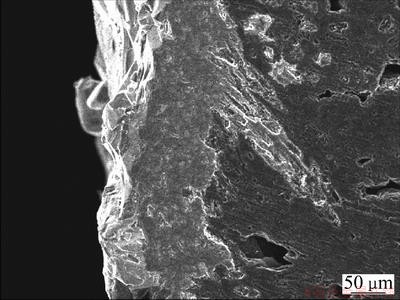
Fig. 3 Cross-section SEM image of coated C/C composites
3.2 Oxidation resistance of multilayer coating at 1773 K
Figure 4 illustrates the isothermal oxidation curves of coated C/C composites at 1773 K. The SiC/MoSi2- SiC-Si coating provides 150 h oxidation protection for C/C composites at 1773 K. After the introduction of ZrB2 to silicon-based coating, the coated multilayer coating shows better oxidation resistance ability. It can efficiently protect C/C composites from oxidation at 1773 K for more than 550 h with corresponding mass gain of 1.25%.
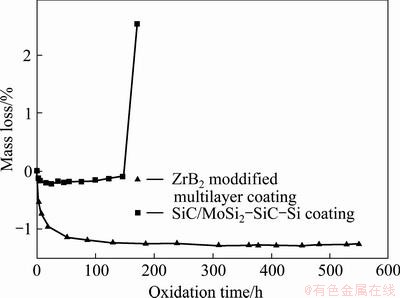
Fig. 4 Isothermal oxidation curves of coated samples in air at 1773 K
As shown in Fig. 4, the oxidation curve of ZrB2 modified coating strictly obeys the exponential damping law during oxidation. At initial oxidation stage, the coating materials are exposed to air and react with oxygen directly. SiC, Si, MoSi2 and ZrB2 are respectively oxidized according to Eqs. (2)–(6). The oxidation of SiC, Si and ZrB2 leads to a mass gain. The oxidation of MoSi2 leads to a mass loss due to the volatilization of MoO3. The mass change of the samples is determined by the mixed oxidation process of SiC, Si, ZrB2 and MoSi2. Because the mass gain covers the mass loss, the mass gain of coated sample reaches 1.14% after oxidation for 51 h, and SiO2 glass is formed on the surface. With increasing oxidation time, SiO2 grows continuously and covers the whole surface, which can provide a long-term oxidation protection for C/C composites. Then, the oxidation speed of the coated sample becomes slow. The coated sample exhibits slight mass gain compared with initial oxidation stage.
SiC(s)+2O2(g)=SiO2(l)+CO2(g) (2)
2SiC(s)+3O2(g)=2SiO2(l)+2CO(g) (3)
Si(s)+O2(g)=SiO2(l) (4)
2ZrB2(s)+5O2(g)=2ZrO2(s)+2B2O3(g) (5)
2MoSi2(s)+7O2(g)=2MoO3(g)+4SiO2(l) (6)
A smooth SiO2 glass thin film is formed on the surface of coated sample after oxidation at 1773 K for 550 h (Fig. 5(a)). The film plays an important role on sealing microcracks in the coating at high temperatures. Compared with the MoSi2–SiC–Si coating after oxidation at 1773 K for 200 h reported by LI et al [5], there are no holes or bubbles in this glass layer. The microcracks in the coating caused by the crystallization of amorphous silica and the thermal expansion coefficient mismatch of coatings and SiO2 can self-seal during oxidation test. So, the effect of microcracks on oxidation protection can be neglected. The XRD pattern of the coating after oxidation (Fig. 5(b)) shows that the coating is composed of SiO2, ZrSiO4 and SiC. Figure 5(c) shows the backscattered electron image of oxidized coating surface. The white particles characterized by EDS are mainly composed of Zr, Si and O. Combined with XRD analysis, the white phase in the coating can be distinguished as ZrSiO4, which is embed in SiO2 glassy film in the form of granules with 1-5 μm in size. ZrSiO4 is formed when SiO2 reacts with ZrO2 generated from the oxidation of ZrB2 according to Eq. (7).
ZrO2(s)+SiO2(l)=ZrSiO4(s) (7)
The high-thermally stable ZrSiO4 phase dispersed in the SiO2 film in granular shape can improve the stability of SiO2 film at high temperatures [23], and the formation of continuous SiO2 glass layer with ZrSiO4 dispersants decreases the diffusion rate of oxygen [24,25]. The oxidation protection of SiO2 glass is enhanced by dispersed ZrSiO4 particulars. So, the ZrB2 modified coating with ZrSiO4-SiO2 compound glass after oxidation exhibits better anti-oxidation performance than SiC/MoSi2-SiC-Si coating.
The cross-section SEM image of the coated sample oxidized at 1773 K for 550 h is shown in Fig. 6. No holes or penetrating cracks can be observed in the coating, which infers that the multilayer coating remains intact and the C/C matrix is almost not oxidized after oxidation. In addition, the thickness of the multilayer coating decreases from 120 to 100 μm which indicates that the consumption rate of coating is very slow.
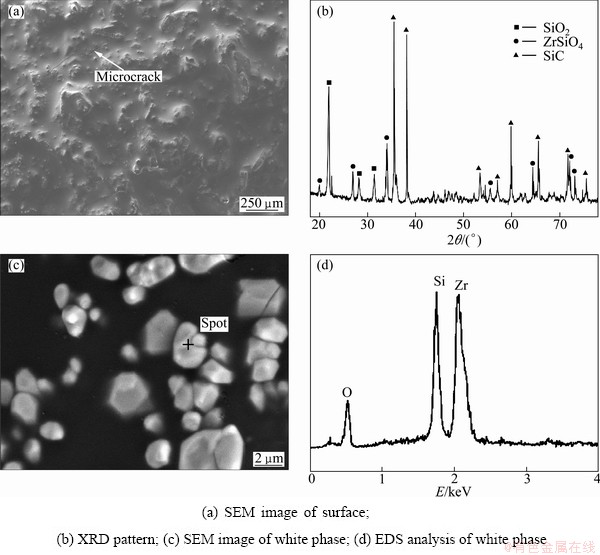
Fig. 5 SEM images and XRD pattern of coated samples after oxidation in air at 1773 K for 550 h
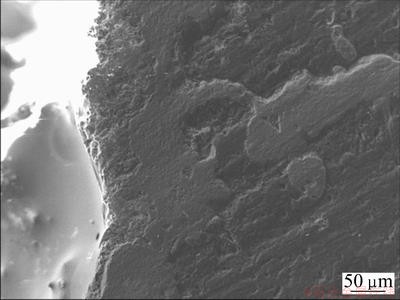
Fig. 6 SEM image of cross-section of coated sample oxidized in air at 1773 K for 550 h
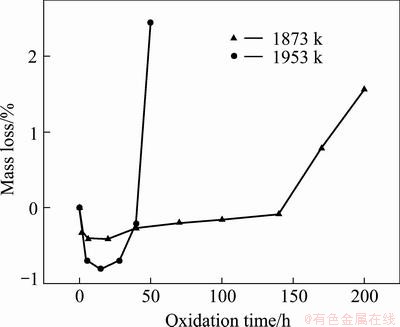
Fig. 7 Isothermal oxidation curves of coated samples in air at 1873 and 1953 K
3.3 Oxidation resistance of multilayer coating at 1873 and 1953 K
Figure 7 illustrates the mass loss curves of the coated C/C composites in air at 1873 and 1953 K. The coated sample exhibits mass gain first and then mass loss during oxidation test at 1873 K. The oxidation behavior can be divided into three stages. At initial oxidation stage, the coated sample exhibits mass gain because of the generation of oxides on the coating surface, which is similar to the oxidation at 1773 K. From 20 to 140 h, the mass starts to decrease. After 140 h, the mass loss increases quickly with time. The mass loss is up to 1.56% after oxidation for 200 h. From the isothermal oxidation curve at 1953 K, it can be seen that the sample shows mass loss quickly after oxidation for 28 h. The mass loss of coated sample is 2.44% after 50 h.
The surface and cross-section micrographs of the coating after oxidation at 1873 K for 200 h and 1953 K for 50 h are shown in Fig. 8. It can be seen that similar ZrSiO4-SiO2 structures are formed on the surface, as shown in Figs. 8(a) and (b). And some bubbles caused by the escape of gas (like SiO2, CO, CO2 and B2O3) can be observed on the coating surface after oxidation at 1953 K (Fig. 8(b)). The SEM images of the cross-section (Figs. 8(c) and (d)) show the existence of oxidation marks of C/C matrix at the end of penetrating cracks. When the coated samples are taken out of the furnace for mass, the coating suffers higher stress at 1873 and 1953 K compared with oxidation at 1773 K, which results in the formation of penetrating cracks in the coating. Oxygen can diffuse through these cracks and result in the oxidation of C/C composites. In addition, some oxidation holes in the coating can be observed after oxidation at 1953 K for 50 h as shown in Fig. 8(d), which may be attributed to the excessive pressure of oxidation gas.
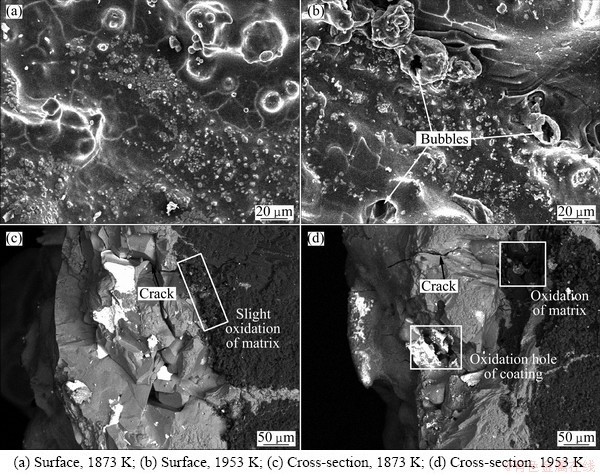
Fig. 8 Surface and cross-section OM images of multilayer coating after oxidation at 1873 K for 200 h and 1953 K for 50 h
ZrSiO4 starts to decompose at 1829 K, and its decomposition reaction accelerates significantly with the increase of temperature [18]. The XRD patterns of coated samples after oxidation at 1873 and 1953 K (Fig. 9) infer that the amount of ZrSiO4 in coating reduces after oxidation at 1953 K. With increasing temperature, the decomposition of ZrSiO4 reduces the stability of SiO2 glass, and also accelerates the volatilization of SiO2, which results in the reduction of anti-oxidation performance of coated sample at 1953 K.
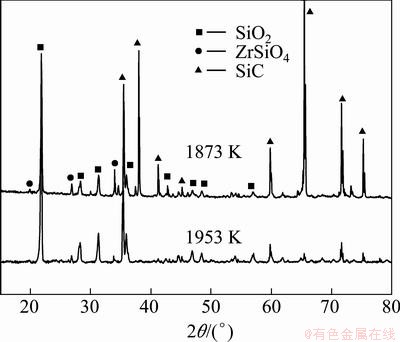
Fig. 9 XRD patterns of multilayer coating after oxidation at 1873 K for 200 h and 1953 K for 50 h
4 Conclusions
1) A dense ZrB2 modified silicon-based multilayer coating is successfully prepared on the surface of C/C composites by pack cementation. The introduction of ZrB2 can improve the anti-oxidation performance of silicon-based coating for C/C composites.
2) The coating can effectively protect C/C composites from the oxidation at 1773 K for more than 550 h. It is attributed to the formation of stable ZrSiO4-SiO2 complexes which effectively improves the stability of SiO2 glassy film.
3) The mass loss is 1.56% and 2.44% after oxidation at 1873 K for 200 h and 1953 K for 50 h, respectively. With increasing temperature, the decomposition of ZrSiO4 and volatilization of SiO2 results in the decrease of anti-oxidation performance.
References
[1] BUCKLEY J D. Carbon-carbon, an overview [J]. American Ceramic Society Bulletin, 1988, 67(2): 364-368.
[2] WINDHORST T, BLOUNT G. Carbon–carbon composites: A summary of recent developments and applications [J]. Materials & Design, 1997, 18(1): 11-15.
[3] JACOBSON N S, CURRY D M. Oxidation microstructure studies of reinforced carbon/carbon [J]. Carbon, 2006, 44(7): 1142-1150.
[4] YANG Xin, SU Zhe-an, HUANG Qi-zhong, CHAI Li-yuan. Preparation and oxidation resistance of mullite/SiC coating for carbon materials at 1150 °C [J]. Transactions of Nonferrous Metals Society of China, 2012, 22(12): 2997-3002.
[5] LI He-jun, XUE Hui, WANG Yong-jie, FU Qian-gang, YAO Dong-jia. A MoSi2-SiC-Si oxidation protective coating for carbon/carbon composites [J]. Surface & Coatings Technology, 2007, 201(24): 9444-9447.
[6] ZHANG Yu-lei, LI He-jun, FU Qian-gang, LI Ke-zhi, HOU Dang-she, FEI Jie. A Si-Mo oxidation protective coating for C/SiC coated carbon/carbon composites [J]. Carbon, 2007, 45(5): 1105-1106.
[7] LI Guo-dong, XIONG Xiang, HUANH Ke-long. Ablation mechanism of TaC coating fabricated by chemical vapor deposition on carbon-carbon composites [J]. Transactions of Nonferrous Metals Society of China, 2009, 19(s3): 689-695.
[8] MORIMOTO T, OGURA Y, KONDO M, UEDA T. Multilayer coating for carbon–carbon composites [J]. Carbon, 1995, 33(4): 351-357.
[9] HUANG Jian-feng, LI He-jun, XIONG Xin-bo, ZENG Xie-rong, LI Ke-zhi, FU Ye-wei, HUANG Min. Process on the oxidation protective coating of carbon–carbon composites [J]. New Carbon Materials, 2005, 20(4): 373-379. (in Chinese)
[10] CAIRO C A A,
M L A, SILVA C R M, BRESSIANI J C. Functionally gradient ceramic coating for carbon–carbon antioxidation protection [J]. Journal of the European Ceramic Society, 2001, 21(3): 325-329.
[11] FU Qian-gang, LI He-jun, SHI Xiao-hong, LI Ke-zhi, SUN Guo-dong. Silicon carbide coating to protect carbon/carbon composites against oxidation [J]. Scripta Materialia, 2005, 52(9): 923-927.
[12] FENG Tao, LI He-jun, FU Qian-gang, ZHANG Yu-lei, SHI Xiao-hong. Microstructure and anti-oxidation properties of multi-composition ceramic coatings for carbon/carbon composites [J]. Ceramic International, 2011, 37(1): 79-84.
[13] HUANG Min, LI Ke-zhi, LI He-jun, FU Qian-gang, SUN Guo-dong. Double-layer oxidation protective SiC/Cr–Al–Si coating for carbon–carbon composites [J]. Surface & Coatings Technology, 2007, 201(18): 7842-7846.
[14] ZHANG Yu-lei, LI He-jun, FU Qian-gang, YAO Xi-yuan, LI Ke-zhi, JIAO Geng-sheng. An oxidation protective Si-Mo-Cr coating for C/SiC coated carbon/carbon composites [J]. Carbon, 2008, 46(1): 179-182.
[15] FAHRENHOLTZ W G, HILMAS G E, TALMY I G, ZAYKOSKI J A. Refractory diborides of zirconium and hafnium [J]. Journal of the American Ceramic Society, 2007, 90(5): 1347-1364.
[16] MONTEVERDE F, SAVINO R. Stability of ultra-high-temperature ZrB2–SiC ceramics under simulated atmospheric re-entry conditions [J]. Journal of the European Ceramic Society, 2007, 27(16): 4797-4805.
[17] HU Ping, WANG Guo-lin, WANG Zhi. Oxidation mechanism and resistance of ZrB2-SiC composites [J]. Corrosion Science, 2009, 51(11): 2724-2732
[18] KAISER A, LOBERT M, TELLE R. Thermal stability of zircon (ZrSiO4) [J]. Journal of the European Ceramic Society, 2008, 28(11): 2199-2211.
[19] WU Ding-xing, DONG Shao-ming, DING Yu-sheng, ZHANG Xiang-yu, WANG Zhen, ZHOU Hai-jun. Preparation and property of SiC/(ZrB2-SiC/SiC)4 anti-oxidation coating for Cf/SiC composites [J]. Journal of Inorganic Materials, 2009, 24(4): 836-840. (in Chinese)
[20] ZHANG Wu-zhuang, ZENG Yi, GBOLOGAH L, XIONG Xiang, HUANG Bai-yun. Preparation and oxidation property of ZrB2–MoSi2/SiC coating on carbon/carbon composites [J]. Transactions of Nonferrous Metals Society of China, 2011, 21(7): 1538-1544.
[21] FENG Tao, LI He-jun, FU Qian-gang, SHEN Xue-Tao, WU Heng. Microstructure and oxidation of multi-layer MoSi2–CrSi2–Si coatings for SiC coated carbon/carbon composites [J]. Corrosion Science, 2010, 52(9): 3011-3017.
[22] ZHANG Yu-lei, LI He-jun, LI Ke-zhi, FEI Jie, ZENG Xie-rong. C/SiC/Si-Mo-Cr multilayer coating for carbon carbon composites for oxidation protection [J]. New Carbon Materials, 2012, 27(2): 105-109.
[23] YAO Xi-yuan, LI He-jun, ZHANG Yu-lei, WU Heng, QIANG Xin-fa. A SiC–Si–ZrB2 multiphase oxidation protective ceramic coating for SiC-coated carbon/carbon composites [J]. Ceramics International, 2012, 38(3): 2095-2100.
[24] GAO Dong, ZHANG Yue, FU Jing-ying, XU Chun-lai, SONG Yang, SHI Xiao-bin. Oxidation of zirconium diboride–silicon carbide ceramics under an oxygen partial pressure of 200 Pa: Formation of zircon [J]. Corrosion Science, 2010, 52(10): 3297-3303.
[25] CHERNIAK D J, WATSON E B. Diffusion in zircon [J]. Reviews in Mineralogy and Geochemistry, 2003, 51(1): 113-143.
炭/炭复合材料ZrB2改性硅基复合涂层的高温抗氧化性能
李贺军,姚西媛,张雨雷,姚栋嘉,王少龙
西北工业大学 凝固技术国家重点实验室,西安 710072
摘 要:为提高硅基涂层的高温抗氧化性能,采用包埋法在炭/炭(C/C)复合材料表面制备ZrB2改性硅基涂层,研究涂层的相组成、微观形貌及其在1773、1873和1953 K时的抗氧化性能。所制备的涂层结构致密,具有良好的抗氧化性能。在1773 K下形成的稳定的ZrSiO4–SiO2氧化保护膜对C/C复合材料提供550 h的氧化保护。涂层试样的抗氧化性能随温度的提高而降低,在1953 K下氧化50 h其质量损失为2.44%,这主要归因于ZrSiO4的高温分解和SiO2保护膜随温度升高的挥发。
关键词:炭/炭复合材料;涂层;ZrB2;抗氧化性能
(Edited by Jing-hua FANG)
Foundation item: Projects (51221001, 50972120) supported by the National Natural Science Foundation of China; Project (73–QP–2010) supported by the Research Fund of the State Key Laboratory of Solidification Processing of Northwestern Polytechnical University, China; Project (B08040) supported by Program of Introducing Talents of Discipline to Universities, China
Corresponding author: He-jun LI; Tel: +86-29-88495764; Fax: +86-29-87783767; E-mail: lihejun@nwpu.edu.cn
DOI: 10.1016/S1003-6326(13)62701-6