
Ferrous ion oxidation by Thiobacillus ferrooxidans
immobilized on activated carbon
ZHOU Ji-kui(周吉奎)1, QIN Wen-qing(覃文庆)2, NIU Yin-jian(钮因健)2, LI Hua-xia(李花霞)1
1. Zhengzhou Research Institute of CHALCO, Zhengzhou 450041, China;
2. School of Resources Processing and Bioengineering, Central South University, Changsha 410083, China
Received 21 September 2005; accepted 13 March 2006
Abstract: The immobilization of Thiobacillus ferrooxidans on the activated carbon particles as support matrix was investigated. Cycling batch operation results in the complete oxidation of ferrous iron in 8 d when the modified 9 K medium is set to flow through the mini-bioreactor at a rate of 0.104 L/h at 25 ℃. The oxidation rate of ferrous iron with immobilized T. ferrooxidans is 9.38 g/(L·h). The results show that the immobilization of T. ferrooxidans on activated carbon can improve the rate of oxidation of ferrous iron. The SEM images show that a build-up of cells of T. ferrooxidans and iron precipitates is formed on the surface of activated carbon particles.
Key words: Thiobacillus ferrooxidans; immobilization; activated carbon; biooxidation; precipitate; bioreactor
1 Introduction
One of the main applications of biotechnology to hydrometallurgy is based on the ability of bacteria such as Thiobacillus ferrooxidans, Thiobacillus thiooxidans and Leptospirillum ferrooxidans to oxidize sulfide minerals to soluble components[1]. Both direct and indirect mechanisms of bacterial leaching have been proposed. In direct mechanism, the bacteria attach on the surface of the mineral, and attack the mineral at the point of attachment by the enzymatic system of bacteria, oxidize them to sulfate. In indirect mechanism, the role of bacteria is to oxidize ferrous ions to ferric ions, and the ferric ions serve as a strong leaching agent of sulfate in the minerals. The direct mechanism of bacterial leaching sulfide mineral can be represented by the following overall reaction:
(1)
Whereas the indirect mechanism requires the presence of ferric ions in solution:
(2)
(3)
In both cases, the bacteria can oxidize elemental sulfur to sulphate acid:
(4)
At the iron oxidation step, a high density of cells of bioleaching bacteria is essential for rapid oxidation. For this reason, many studies of T. ferrooxidans have been carried out to improve the rate of Fe(Ⅱ) oxidation.
The immobilization technology of cells and enzymes has rapidly developed and has many applica- tions[2]. The immobilization of cells is characterized by maintaining a stable high density of cells in or on the support matrix. There are many matrices used to immobilize the cells of T. ferrooxidans by means of adhesion and entrapment[3,4]. For example, sand[5], ion-exchange resin, activated carbon[6], nickel alloy fibre[7] and low-grade ore[8] have been used as adhesion matrices, and agar, polyurethame foam[9], calcium alginate[10], к-carrageenan and gerlite have been used as entrapment matrices[8].
Because the immobilization of T. ferrooxidans can increase the density of cells and improve the oxidative rate of Fe(Ⅱ) and sulfide, it has been applied to treat acid mine-drainage, bioleach metal sulfide and remove H2S from sour gases.
In order to improve the kinetics of bacterial leaching of sulfide concentrates such as spheralite and chalopyrite, the IBES (Indirect Bioleaching with Effects Separation) can be separated into chemical and biological reactions using different reactors so that both stages can be individually optimized[11-13]. In the stage of chemical oxidation of metal sulfide, ferric irons are the lixiviant agent (Eqn.(2)). In the stage of biological reaction, the bacteria oxidize ferrous iron and elemental sulfur (Eqns.(3) and (4)) to ferric iron and sulphuric acid respectively. The regenerated ferric iron is then used in new chemical oxidation.
The aim of this study is to determine the effect of activated carbon used for immobilization of T. ferrooxidans on the rapid iron oxidation.
2 Experimental
2.1 Microorganism and culture medium
The bacterial strain used in this study (T. ferrooxidans) was obtained from the Dabaoshan Mines of Guangdong, China. T. f culture was maintained on modified 9K medium with a reduced iron content: (NH4)2SO4 3.0 g/L; K2HPO4 0.5 g/L; KCl 0.1 g/L; Ca(NO3)2 0.01 g/L; FeSO4·7H2O 22.5 g/L. The bacterial cultures were activated and maintained at 30 ℃ using a bacterial incubator shaker at 200 r/min. Subcultures were carried out every 3 d to keep the bacteria in the loga- rithmic phase of growth.
2.2 Support material
The granular activated carbon (2-4 mm size fraction) was used as the immobilization matrix. The activated carbon immobilizes T. ferrooxidans by adhesion.
2.3 Immobilization procedure
Cells of T. ferrooxidans were immobilized on activated carbon according to following procedure: 25 g activated carbon which was washed with H2SO4 solution of pH 1.5 was added into a 500 mL flask containing 240 mL 9K medium and 10 mL T. ferrooxidans culture in the logarithmic phase of growth. The flask was shaken at 200 r/min on an orbital shaker at 30 ℃. When total ferrous iron oxidation conversion was obtained, the flask was drained and the media was replaced with 250 mL fresh 9K medium. Several batches were carried out until the speed of ferrous iron oxidation was stable[14].
2.4 Analytical method
The oxidation of ferrous sulfate by T. ferrooxidans was monitored by determining the remainder ferrous iron concentration at various intervals. The method of potassium bichromate titration was used. 2 mL sample was placed in a 50 mL beaker. Then 10 mL of 30% mixture acid of phosphoric acid and sulfuric acid with ratio of 1:1 was added. This sample was titrated with potassium bichromate until the solution changed in color from achromaticity to purple. A calibration curve of known Fe(Ⅱ) concentration was used to calculate the iron concentrations. The concentration of Fe(Ⅲ) was estimated by subtracting the Fe(Ⅱ) concentration measured regularly at an interval throughout the leaching period from the total iron concentration.
2.5 Mini-reactor experiment
25 g of inoculated activated carbon was packed into a glass column of 5.2 cm in length with a diameter of 4.1 cm. 5 L of 9K medium containing 110 g FeSO4·7H2O was set to flow through the column at a rate of 0.104 L/h at 25 ℃. The working volume in column was 12.5 mL. The ferrous sulfate oxidation by immobilized T. ferrooxidans was monitored by measuring ferrous concentration. The experiment continued until the rate of ferrous oxidation was 100%.
2.6 Preparation of samples for SEM observation
The activated carbon samples were removed from mini-reactor in 1 cm below the top at the termination of experiment. The samples were gently washed with sulfuric acid of pH 2.0 and fixed for 1 h with 2.5% glutaraldehyde dissolved in 0.05 mol/L sulfuric acid. After fixation, the samples were rinsed for three times with sulfuric acid of pH 2.0, followed by dehydrated and air-dried. The samples were then fixed on specimen stubs, gold-coated and then observed with SEM at 30 kV.
3 Results and discussion
3.1 Liquid culture oxidation
In order to study the oxidation of ferrous sulfate by immobilized cells of T. ferrooxidans on activated carbon, the liquid culture oxidation was carried out in a 250 mL flask containing 100 mL 9K medium (Fe(Ⅱ) concentra- tion is 4.5 g/L ) with 10%(volume fraction) inoculum. The original density of T. ferrooxidans was about 5.0×106 cells/mL. The culture was incubated at 30 ℃ on a rotary shaker at 200 r/min. As shown in Fig.1, it needed 28 h for all ferrous irons to be oxidized, and the density of bacteria reached 5.2×107 cells/mL in the logarithmic phase of growth.
When the ferrous iron was completely oxidized, the culture was filtered using Millipore filter of 0.22 μm. The used filter was rinsed with 10 mL uninoculated 9K medium in order to collect the total cells of T. ferrooxidans. The number of cells in the logarithmic phase of growth in 100 mL 9K medium was about 5.2×109 cells. After this 10 mL inoculum (7.5×109cells) was placed in a 250 mL flask containing 90 mL 9K medium and 2.25 g FeSO4·7H2O, the culture was incubated at 30 ℃ on a rotary shaker at 200 r/min. In Fig.2, the time of complete oxidation of ferrous iron was only 9 h, resulting in the Fe2+ oxidation rate of 0.5g/(L·h).
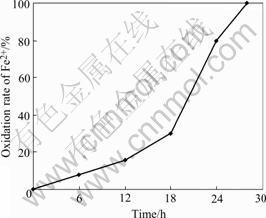
Fig.1 Oxidation of Fe2+ by freely suspended T. ferrooxidans with 10% inoculum
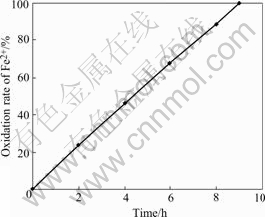
Fig.2 Oxidation of Fe2+ in 9K medium containing 7.5×109 logarithmic cells
3.2 Immobilized cells oxidation
The activated carbon with inoculated cells of T. ferrooxidans was packed into a glass column, the mass of the activated carbon was 25 g (as shown in Fig.3). The mini-reactor took 30 d to stabilize the oxidation rate of ferrous iron. In Fig.4, the batch operation of mini-reactor resulted in the complete oxidation of ferrous iron after 4 batches or 8 d, resulting in the Fe2+ oxidation rate of 9.38 g/(L·h). The oxidation rate was calculated using the working volume, 12.5 mL.
3.3 Immobilized cells on activated carbon
From Fig.5, it can be seen that T. ferrooxidans formed biofilm on the surface of activated carbon particles. This biofilm consisted of bacteria and jarosite. There were some sites where the adhesion of cells of T. ferrooxidans occurred preferentially. Furthermore, if we considered that some cells of T. ferrooxidans were entrapped in precipitates (jarosite), the calculated adhered biomass per gram of activated carbon was less than actual biomass[15].
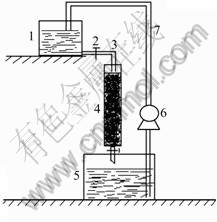
Fig.3 Packed-bed mini-reactor containing activated carbon inoculated with T. ferrooxidans: 1 Fresh medium; 2 Switch; 3 Inlet pipe; 4 Column; 5 Effluent culture; 6 Recycle pump; 7 Recycle pipe
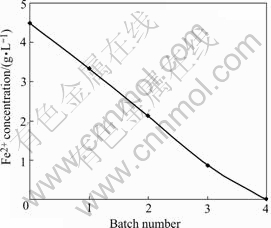
Fig.4 Oxidation of Fe2+ by immobilized cells in mini-reactor
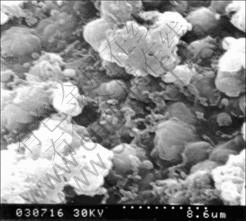
Fig.5 SEM photo of biofilm formed on activated carbon particles
4 Conclusions
1) For the purpose of improving the rate of iron oxidation, a high density of cells of iron-oxidizing bacteria is important. The use of immobilized T. ferrooxidans has been addressed as a promising method to improve the rate of iron oxidation due to its high and stable level of biomass.
2) The activated carbon has a very large surface area relative to its volume, which favours the natural tendency of the cells of T. ferrooxidans to adhere to solid surfaces, and produces a high rate of colonization. In this study, the oxidation rate of iron is 9.38 g/(L·h).
3) The formation of biofilm is not homogeneous over the full surface of activated carbon particles. There are some sites where the adhesion of cells of T. ferrooxidans occurs preferentially. The mini-bioreactor takes 30 d to stabilize the oxidation rate of ferrous irons because it requires a long time to reach the maximal biomass of cells adhered on the surface of activated carbon particles and to adapt the changed environment.
References
[1] SHI Shao-yuan, FANG zhao-heng. Bioleaching of marmatite flotation concentrate by Acidithiobacillus ferrooxidans and Leptospirillum ferrooxidans [J]. Trans Nonferrous Met Soc China, 2004, 14(3): 569-575.
[2] GARCIA M J, PALENCIA I, CARRANZA F. Biological ferrous iron oxidation in packed-up columns with low-grade sulfide minerals as support [J]. Process Biochem, 1989, 24: 84-87.
[3] GRISHIN S I, TUOVINEN O H. Fast kinetics of Fe2+ oxidation in packed-up reactors [J]. Appl Environ Microbiol, 1998, 54: 3092-3100.
[4] MYERSON A S, KLINE P. The adsorption of Thiobacillus ferrooxidans on solid particles [J]. Biotechnol Bioeng, 1983, 25: 1669-1676.
[5] WOOD T A, MURRAY K R, BURGES J G. Ferrous sulfate oxidation using Thiobacillus ferrooxidans cells immobilized on sand for the purpose of treating acid mine-drainage [J]. Appl Microbiol Biotechnol, 2001, 56: 560-565.
[6] KAI T, SUENAGE Y, MIGITA A, TAKAHASHI T. Kinetic model for simultaneous leaching of zinc sulfide and manganese dioxide in the presence of iron-oxidizing bacteria [J]. Chem Eng Sci, 2000, 55: 3429-3436.
[7] GOMEZ J M, CANTERO D, WEBB C. Immobilisation of Thiobacillus ferrooxidans cells on nickel alloy fibre for ferrous sulfate oxidation [J]. Appl Microbiol Biotechnol, 2000, 54: 335-340.
[8] FU Jian-hua, HU Yue-hua, QIU Guan-zhou, LIU Jian-she, XU Jing. Biofilm forming and leaching mechanism during bioleaching chalcopyrite by Thiobacillus ferrooxidans [J]. Trans Nonferrous Met Soc China, 2004, 14(2): 383-387.
[9] ARMENTIA H, WEBB C. Ferrous sulfate oxidation using Thiobacillus ferrooxidans cells immobilized in polyurethane foam support particles [J]. Appl Microbiol Biotechnol, 1992, 36: 697-700.
[10] LANCEY E D, TUOVINEN O. Ferrous ion oxidation by Thiobacillus ferrooxidans immobilized in calcium alginate [J]. Appl Microbiol Biotechnol, 1984, 20: 94-99.
[11] KAI T, TAKAHASHI T, SHIRAKAWA Y, KAWABATE Y. Decrease in iron oxidizing activity of Thiobacillus ferrooxidans adsorbed on activated carbon [J]. Biotechnol Bioeng, 1990, 36: 1105-1109.
[12] CARRANZA F, IGLESLAS N. Application of IBES process to a Zn sulfide concentrate: Effect of Cu2+ ion [J]. Miner Eng, 1998, 11(4): 385-390.
[13] CARRANZA F, IGLESLAS N, ROMERO R, PALENCIA I. Kinetics improvement of high-grade sulfides bioleaching by effects separation [J]. TEMS Microbiol Reviews, 1993, 11: 129-138.
[14] HARVEY P I, CRUNDWELL F K. Growth of Thiobacillus ferrooxidans: A novel experimental design for batch growth and bacterial leaching studies [J]. Appl Environ Microbiol, 1997, 63(7): 2586-2597.
[15] NEMATI M, WEBB C. Effect of ferrous iron concentration on the catalytic activity of immobilized cells of Thiobacillus ferrooxidans [J]. Appl Microbiol Biotechnol, 1996, 46: 250-255.
(Edited by YUAN Sai-qian)
Corresponding author: ZHOU Ji-kui; Tel: +86-731-68918301; E-mail: zjk@rilm.com.cn