Catalytic mechanism of Cu(p-OTs)2/ethanolamine as mimetic enzyme
SONG Ji-guo (宋继国), SHEN Pei-kang (沈培康)
(State Key Laboratory of Optoelectronic Materials and Technologies,School of Physics and Engineering, Zhongshan University, Guangzhou 510275, China)
Abstract: The electrochemical behaviors of various copper salts complexes coordinated with equal molar ethanolamine were studied, and those of Cu(p-OTs)2 and Cu(p-OTs)2/ethanolamine(1∶1) complex in CH3OH or DMF were characterized. The results show that the reduction of Cu(Ⅱ) in Cu(p-OTs)2 is via one two-electron step mechanism both in CH3OH and DMF. The reduction mechanism transforms to two one-electron steps in the case of Cu(p-OTs)2/ethanolamine(1∶1) in DMF. However, it does not change in CH3OH. All the Cu(Ⅱ)/ethanolamine(1∶1) with the electrochemical reactions are through two one-electron steps, and can act as mimetic enzyme to oxidize 1,1′-bi-2-naphthol. The Cu(Ⅱ)/ethanolamine(1∶1) with electrochemical reactions through one two-electron step could not act as mimetic enzyme. It is concluded that the transformation between centre Cu(Ⅱ) and Cu(Ⅰ) is the crucial condition for the catalytic activity of copper-amine complex.
Key words: copper p-toluenesulfonate; mimetic enzyme; 1,1′-bi-2-naphthol; electrochemistry CLC number: O646
Document code: A
1 INTRODUCTION
Most enzymes contain copper ion. The studies on copper containing mimetic enzymes are an important embranchment in biomimetic chemistry. Blue copper proteins have the functions of carrying oxygen and adding oxygen[1-4]. Tyrosinase is a copper-containing monooxygenase[5] and some model compounds have been synthesized[6, 7]. Arrays consisting of organic free radicals proximate to metal centers have been identified as important components of active sites in enzymes that catalyze multielectron redox reactions.
The literature reports that copper chloride/amine can catalyze the oxidation of phenols to benzoquinones by O2[8, 9]. It is recently reported that the complex of CuCl2/ethanolamine(1∶1) can catalyze the oxidation of 1, 1′-bi-2-naphthol in methanol with high yield[10]. In order to deeply understanding the role of copper in the enzyme catalysis, we have studied the catalytic activity of different Cu(Ⅱ)/ethanolamine(1∶1) complex for the oxidation of 1, 1′-bi-2-naphthol at the presence of O2 in various media. This paper also presents the electrochemical results of Cu(p-OTs)2 and Cu(p-OTs)2/ethanolamine (1∶1) in N, N-dimethylformamide(DMF) or methanol(CH3OH), respectively.
2 EXPERIMENTAL
The electrochemical measurements were carried out on a French VoltaLab 80 electrochemical workstation (Radiometer Analytical). The working electrode was a Pt (99.9 %) wire (0.073cm2) and the counter electrode was a Pt (99.9 %) sheet. The saturated calomel electrode (SCE) was used as reference electrode. All the potential values in this paper are quoted versus SCE. Purified argon was bubbled through the electrolytic solution to remove oxygen. The electrochemical mea-surements were carried out in an argon atmosphere at room temperature.
1H-NMR was recorded in a Varian Unity INOVA-500 spectrometer in CDCl3 with TMS as the internal standard. Mass spectra (MALDI-TOF-MS) were taken on REFLEX 3 Bruker matrix assisted laser desorption/ionization time of flight mass spectrometry. Elemental analyses were conducted on a Perkin-Elmer 204 elemental analyzer. Melting points were determined on a Thiele tube.
N,N-Dimethylformamide(DMF) and CH3OH was distilled under vacuum after added 4A molecular sieves to remove water. The supporting electrolyte tetrabutyl ammonium perchlorate (n-Bu)4NClO4 or TBAP) was prepared according to Ref.[11]. Copper p-toluenesulfonate was prepared by the reaction of CuO (99.95 %) and p-CH3C6H4SO3H·H2O. The hydrated product was determined as Cu(p-OTs)2·6H2O by means of thermogravimetry. The structure of Cu(p-OTs)2·6H2O was also proved by X-ray crystallography. The freshly prepared copper p-toluene sulfonate can lose all crystal water easily at 120℃ under 0.5-1kPa and preserved in a desiccator containing anhydrous CaCl2. All the reagents were analytical pure. Aqueous solutions were prepared by double distilled-deionized water.
Cu(p-OTs)2/ethanolamine (1∶1) complex can catalyze the oxidation of 1,1′-bi-2-naphthol as mimetic enzyme. The general procedure is described as follows: 50mg 1,1′-bi-2-naphthol was added to a flask containing 2mL DMF solvent, then mixed with 20mL 0.01mol/L Cu(p-OTs)2/ethanolamine(1∶1) complex and dissolved in DMF. The solution was stirred at 60℃ and oxygen was bubbled through the solution. 2mL ammonia solution and 50mL water were added to the solution and extracted with chloroform (3×10mL) after the reaction completed. The main product peri-xanthenoxanthene with mass of 10.5mg, yield of 21 % was then isolated by evaporation of CHCl3 (Reaction 1). The final product was dried by anhydrous CaCl2 followed by column chromatography on silica gel using petroleum ether and ethyl acetate (10∶1) as eluant. 1HNMR (500MHz, CDCl3, δ ppm): 6.63-6.65 (m, 2 H, H-1, H-7), 6.91 (d, 2 H, J=9.2 Hz, H-3, H-9), 7.07-7.09 (m, 4 H, H-2, H-5, H-8, H-11), 7.29 (d, 2 H, J=8.9 Hz, H-4, H-10); MALDI-TOF-MS: 283 ([M+H]+), analytical calculation: for C20H10O2: C 85.09, H 3.57, experiment: C 84.97, H 3.61; melt point: 238-239℃ (literature: 238℃).
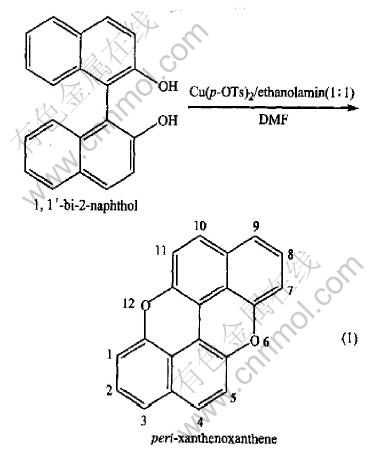
However, the Cu(p-OTs)2/ethanolamine (1∶1) complex is inactive towards the oxidation of 1,1′-bi-2-naphthol in CH3OH(monitored by thin layer chromatography, TLC) under the same conditions as in DMF. The oxidation of 1,1′-bi-2-naphthol can be catalyzed by the Cu(p-OTs)2/ethanolamine(1∶1) complex in CH3OH/DMSO (1∶1) solution and gives the same product as in Ref.[10].
3 RESULTS AND DISCUSSION
3.1 Electrochemical behavior of Cu(p-OTs)2 in CH3OH
There are two kinds of electroreduction processes of Cu2+ in different solutions. The first one is that Cu2+ is reduced to Cu by two one-electron processes. It appears two cathodic peaks on the CV curve, which is called “two one-electron steps” in this paper. Another one is that Cu2+ is reduced to Cu directly by one two-electron process. In this case, only one cathodic peak appears on the CV curve, which is a “one two-electron step” process, the reactions are as follows.
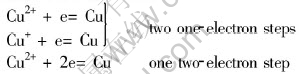
Fig.1(a) shows the cyclic voltammograms of Cu(p-OTs)2/TBAP/CH3OH system on a Pt electrode at different scan rates. There is only one pair of redox peak on the curve. The potential corresponds to the cathodic stripping of Cu. The surface of Pt electrode appears yellow compact deposit after 5min electrolysis at the potential of cathodic peak. There is no electroactive species between -200 and 600mV after Cu2+ was changed to Na+ (Fig.1(b)). The results show that the cathodic peak is corresponding to the electroreduction process of Cu2+ to Cu. It is proved that the electrochemical reduction of Cu(p-OTs)2 in CH3OH is through one two-electron step. The potential of cathodic peak, Epc in Fig.1 changes with the scan rate. The relationship between the cathodic peak current (Ip) and the square root of the scan rate (v1/2) is linear as shown in the inset of Fig.1. For the irreversible electrode process[12]:
│Ep-Ep/2│=1.857RT/(αnαF)(1)
where Ep, Ep/2, α, nα and T are the peak potential, half peak potential, transfer coefficient and electron number in the rate-determining step and temperature, respectively. R=8.314J·mol·L-1, F=96485C·mol-1.
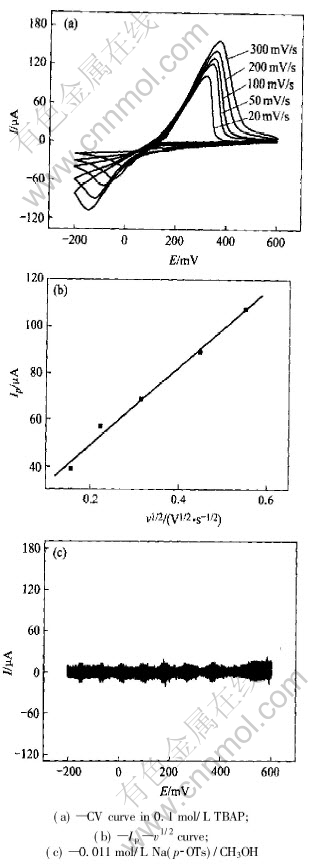
Fig.1 Cyclic voltammograms of Pt electrode (0.073cm2) at different scan rates in 0.1mol/L TBAP/0.011mol/L Cu(p-OTs)2/CH3OH
The data of αnα is 1.08. The transfer coefficient α is calculated as 0.54 when nα equal 2. The data of α is usually about 0.5 or less than 0.5, so it is reasonable. The electron number transferred in the cathodic peak is 2.
The irreversible charge transfer process obeys the Randles-Sevcik equation[12]:
Ip=0.4958nF3/2(αnαDv/RT)1/2Ac (2)
where Ip, n, D, v, A and c are the peak current, electron number, diffusion coefficient, scan rate, area of work electrode and Cu2+ concentration.
According to the slope of the line in the inset of Fig. 1 and Eqn.(2), the diffusion coefficient of Cu(Ⅱ) in Cu(p-OTs)2/TBAP/CH3OH at 298K is calculated as 1.44×10-7cm2·s-1.
Fig.2 shows the chronoamperometric curves in Cu(p-OTs)2/TBAP/CH3OH solution at different potential steps. The plot of I-t1/2 shows a linear relationship (Fig.2(b)). The diffusion coefficient of the reactant can also be calculated from[12]:

where I, Kf and t are the limit diffusion coefficient, reactive rate constant and time respectively. At a given slope datum of the line in the inset of Fig.2, the calculated value is 4.36×10-7cm2·s-1, which is lager than that calculated by the result from the linear sweep potential voltammetry.
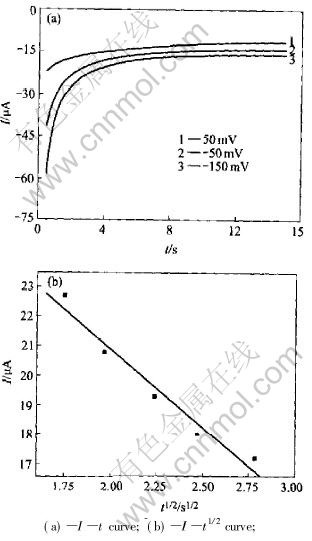
Fig.2 Chronoamperometric curves on Pt electrode in 0.1mol/L TBAP and 0.011mol/L Cu(p-OTs)2/CH3OH at different potential steps
Fig.3 is the cyclic voltammogram of Pt electrode in Cu(p-OTs)2/TBAP/CH3OH after adding equivalent ethanolamine. The result shows that the reduction process of Cu2+ is through one step because only one pair of redox peak appears in Fig.3. The changes of cathodic peak potential and anodic peak potential indicate that the copper/amine complex has formed.
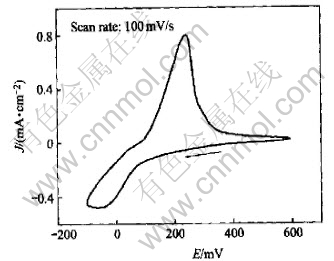
Fig.3 Cyclic voltammogram of Cu(p-OTs)2/ ethanolamine (1∶1) in CH3OH solution
Cu(p-OTs)2 has been shown to have octahedral coordination of copper ions with a [CuO6] skeleton in which OTs- acts as tridentate bridging ligand through the three oxygen atoms bonded to sulfur[13]. The solvating power of CH3OH is weak, therefore, the Cu(p-OTs)2 can not be solvated well and Cu(Ⅱ) and OTs- exist as tight ion pair. Fig.4 shows the CV of Cu(p-OTs)2/ethanolamine in CH3OH/DMSO (1∶1) solution. The electroreduction of Cu(Ⅱ) transforms two one-electron steps because the solvated power of DMSO is stronger enough to make the Cu(I) stable.
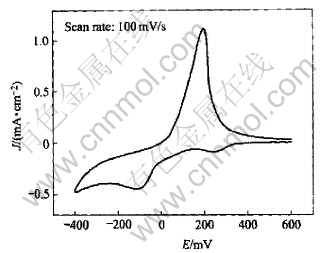
Fig.4 Cyclic voltammogram of Cu(p-OTs)2/ethanolamine(1∶1) in CH3OH/DMSO (1∶1) solution
3.2 Electrochemical behavior of Cu(p-OTs)2
The cyclic voltammograms of Pt electrode in Cu(p-OTs)2/TBAP/DMF with different scan rates are shown in Fig.5(a). Only one pair of redox peak appears. Metal Cu is deposited on the surface of the electrode after 5min electrolysis at the cathodic peak potential. It shows that the cathodic peak is corresponding to the reduction of Cu2+ to Cu and the reductive reaction is controlled by one two-electron step mechanism in DMF. The transfer coefficient α is 0.48 calculated using Eqn.(1) and nα=2.
According to the slope of the linear curve Fig.5(b) and Eqn.(2), the diffusion coefficient of Cu(Ⅱ) in Cu(p-OTs)2/TBAP/DMF at 298K is calculated as 0.49×10-7cm2·s-1. It shows that the diffusion coefficient of Cu(Ⅱ) decreases with the increasing viscosity of the solvent. The viscosity of DMF is 7.96×10-3 poise and is larger than that of CH3OH, which is 5.43×10-3 poise.
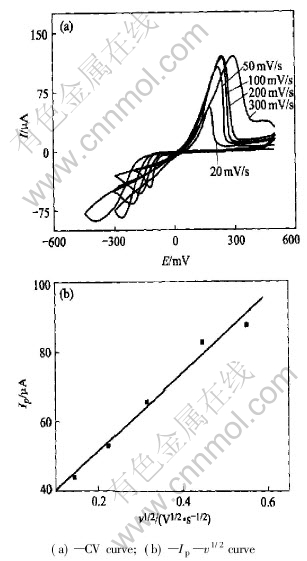
Fig.5 Cyclic voltammograms of Pt electrode (0.073cm2) at different scan rates in 0.1mol/L TBAP and 0.012mol/L Cu(p-OTs)2/DMF
Fig.6 shows the cyclic voltammogram of Pt electrode in Cu(p-OTs)2/TBAP/DMF after adding equivalent ethanolamine. Two cathodic peaks (202mV and -105mV) appear during the reduction in contrast to one peak in the solution without ethanolamine. It is presumed that both ethanolamine and DMF make the Cu(Ⅰ) stable with stronger solvated power than CH3OH.
3.3 Performance of Cu(Ⅱ)/ethanolamine (1∶1) as mimetic enzyme
The oxidative mechanism of 1,1′-bi-2-naphthol catalyzed by Cu(Ⅱ)/ ethanolamine (1∶1) complex can be concluded as shown in Fig.7. The previous literature has reported the catalytic mechanism based on the intermediate obtained[10]. But the coordination of mimetic enzyme and substrate, and the valence change of metal ion of active center in mimetic enzyme were not discussed. These points are very important to understand reactive
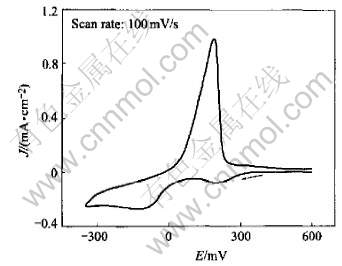
Fig.6 Cyclic voltammogram of Cu(p-OTs)2/ ethanolamine (1∶1) in DMF solution
processes. Further experimental data are needed to demonstrate the catalytic mechanism. The complexes of various copper salts with ethanolamine have been tested and summarized in Table 1. The complexes that the electroreduction is by two steps can be used as mimetic enzyme to catalyze the oxidation of 1,1′-bi-2-naphthol. However, the complexes that behave one step reduction mechanism do not have catalytic activity.
Table 1 Performance of different cupric salt/ethanolamine(1∶1) complexes
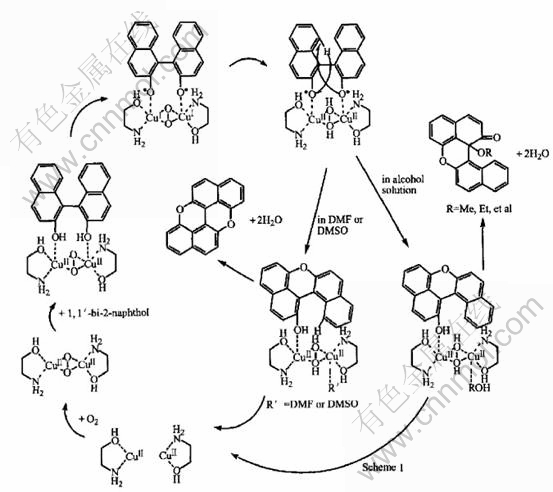
Fig.7 Oxidative mechanism of 1,1′-bi-2-naphthol catalyzed by Cu(Ⅱ)/ethanolamine
The derivatives of xanthone consist in natural products widely and have many physiological activities as astringent, antispasmodic and antibiotic agent[14] and so on. This work uses the complex of Cu(p-OTs)2/ethanolamine (1∶1) as mimetic enzyme to synthesize compounds with physiological activities. In the present work, Cu(p-OTs)2/ethanolamine (1∶1) was firstly applied for the catalytic reaction. The results show that the complex of Cu(p-OTs)2/ethanolamine (1∶1) can catalyze the oxidation of 1,1′-bi-2-naphthol in DMF. However, it does not work in CH3OH.
4 CONCLUSIONS
1) The electrochemical behaviors of Cu(p-OTs)2 was studied on platinum electrode in CH3OH and DMF solutions for the first time. The results show that the electrochemical reduction of Cu(p-OTs)2 proceeds by one two-electron step in CH3OH and DMF solution. However, the reaction transforms to two one-electron steps by the addition of ethanolamine in DMF.
2) The complex of Cu(p-OTs)2/ethanolamine (1∶1) can catalyze the oxidation of 1,1′-bi-2-naphthol in DMF. This reaction does not work in CH3OH. Summarizing the electrochemical behaviors of various Cu(Ⅱ)/ethanolamine complexes, it can be concluded that the electron transfer from 1,1′-bi-2-naphthol to O2 becomes easily through the transformation between the centre Cu(Ⅱ) and Cu(Ⅰ), so it can catalyze the oxidation of substrate.
REFERENCES
[1]Lee D H, Wei N, Murthy N N, et al. Reversible O2 binding to a dinuclear copper(I) complex with linked tris(2-pyridylmethyl)amine units: kinetic-thermodynamic comparisons with monoclear analogues [J]. J Am Chem Soc, 1995, 117(50): 12498-12513.
[2]Tolman W B. Making and breaking the dioxygen O—O bond: new insights from studies of synthetic copper complexes [J]. Acc Chem Res, 1997, 30(6): 227-237.
[3]Itoh S, Taki M, Nakao H, et al. Aliphatic hydroxylation by a bis(μ-oxo)dicopper(III) complex [J]. Angew Chem Int Ed Engl, 2000, 39(2): 398-400.
[4]Reglier M, Jorand C, Waegell B. Binuclear copper complex model of Tyrosinase [J]. J Chem Soc Chem Commun, 1990: 1752-1755.
[5]Wilcox D E, Porras A G, Hwang Y T, et al. Substrate analogue binding to the coupled binuclear copper active site in Tyrosinase [J]. J Am Chem Soc, 1985, 107(13): 4015-4027.
[6]Reglier M, Amadei E, Tadayoni R, et al. Pyridine nucleus hydroxylation with copper oxygenase models [J]. J Chem Soc Chem Commun, 1989: 447-450.
[7]Sorrel T N. Synthetic models for binuclear copper proteins [J]. Tetrahedron, 1989, 45(1): 3-68.
[8]Takehira K, Shimizu M, Watanabe Y, et al. A novel oxygenation of 2,3,6-trimethylphenol to trimethyl-p-benzoquinone by dioxygen with copper(Ⅱ) chloride/amine hydrochloride catalyst [J]. Tetrahedron Letters, 1989, 30(48): 6691-6692.
[9]Rogic M M, Demmin T R. Cleavage of carbon-carbon bonds. Copper(Ⅱ)-induced oxygenolysis of o-benzoquinones, catechols, and phenols. On the question of nonenzymatic oxidation of aromatics and activation of molecular oxygen [J]. J Am Chem Soc, 1978, 100(17): 5472-5487.
[10]Tan D M, Li H H, Wang B, et al. A novel domino reaction of 1,1′-bi-2-naphthol catalyzed by copper(Ⅱ)-amine complexes [J]. Chinese J Chem, 2001, 19(1): 91-96.
[11]SONG Ji-guo, SONG Hua-can, YANG Qi-qin, et al. Electroreduction of Cu(Ⅱ) in Cu(p-CH3C6H4SO3)2+DMSO [J]. Acta Phys -Chim Sin, 2003, 19(3): 283-285.
[12]LIU Peng, YANG Qi-qin, LIU Guan-kun. Electroreduction of Zn2+ and Ni2+ and Cu2+ in urea-chlorides melt [J]. Trans Nonferrous Met Soc China, 1996, 6(2): 41-44.
[13]Kapila V P, Kapila B, Kumar S. Chemistry of substituted sulphuric acids: Part XX - Transition metal p-tolylsulphates and their complexes [J]. Indian J Chem, 1991, 30A(10): 908-911.
[14]Ahuwalia V K, Tehim A K. Synthesis of some 1,3,6-trioxygenated isopentenylated xanthones [J]. Tetrahedron, 1984, 40(17): 3303-3312.
(Edited by LONG Huai-zhong)
Foundation item: Projects (01105500; 2003B12006; 013024) supported by the Key Projects of Guangdong Province; project (2003Z2-D0081) supported by the Key Project of Science and Technology of Guangdong Province
Received date: 2004-04-19; Accepted date: 2004-09-21
Correspondence: SHEN Pei-kang, Professor; Tel: +86-20-84113369; Fax: +86-20-84113369; E-mail: stdp32@zsu.edu.cn