
Determination of mercury and nickel by amperometric biosensor prepared with
thermostable lactate dehydrogenase
?brahim TAN, Elif ERHAN, P?nar KARAG?Z, Melek ?ZKAN
Department of Environmental Engineering, Gebze Institute of Technology, 41400 Kocaeli, Turkey
Received 14 December 2010; accepted 16 March 2011
Abstract: Response of biosensor prepared with the thermostable bacterial LDH enzyme was analyzed in the presence of mercury and nickel. For electrode preparation, the enzyme was purified and immobilized on a gold sheet coated by PGA-pyrrole polymeric material. The working electrode was tested at increasing concentration of lactate in the presence of two different concentrations of mercury and nickel. Current response of biosensor decreased from 0.32 μA to 0.09 μA and 4.13 μA to 2.63 μA when 25×10-7 mmol/L mercury and 17×10-5 mmol/L nickel were included in the working solution, respectively. Sensitivity of the electrode decreased from 0.010 2 μA/(mmol·L-1) to 0.0043 μA/(mmol·L-1) in the presence of 25×10-7 mmol/L mercury. On the other hand, the presence of nickel did not result in a decrease in electrode sensitivity. The results pointed out that the prepared biosensor is useful to detect mercury in a sample containing both mercury and nickel together.
Key words: enzyme inhibition; amperometric biosensor; heavy metals
1 Introduction
The presence of heavy metal salts in water sources is dangerous and very important problem for human health and other living organisms. Although there are various methods for analyzing water quality, few enzymatic analytical methods are available for direct determination of heavy metal salts and organics in the environmental samples. Being fast, sensitive and easy usage, biosensor technology is useful for a rapid analysis. Biosensors based on enzyme inhibition have been applied in a wide range of inhibitory substances such as derivatives of insecticides and pesticides, heavy metals and glycoalkaloids. Inhibition of enzyme activity by those substances results in decrease in product concentration [1]. This property of enzymes can be used to develop biosensors, which work in defined standard concentration ranges, for determination of contamination in drinking waters [2-4].
Several enzymes were used for determination of mercury and its compounds. Urease, peroxidase, isocitric dehydrogenase and glucose oxidase are a few of those enzymes [5]. Recent studies on enzyme inhibition-based biosensors were mostly performed with urease or glucose oxidase by immobilizing the enzymes in various conducting materials such as polyvinyl ferrocenium film [6], polyaniline membrane and ferrocene [7] or poly-o-phenylenediamine [5]. There are few reports on inhibition-based biosensors using lactate dehydrogenase enzyme [8].
In this study, an electrochemical biosensor was prepared with a bacterial thermostable lactate dehydrogenase enzyme. L-lactate dehydrogenase catalyzes the interconversion of lactate and pyruvate using NAD+ as a coenzyme. It is usually a tetrameric enzyme composed of 35 kDa subunits [9]. Allosteric L-lactate dehydrogenases (LDH, EC1.1.1.27) are regulated by fructose-1, 6-diphosphate (FDP). NAD+ is reduced to NADH in the reaction between LDH and lactate through which lactate is converted to pyruvate. In a biosensor prepared with lactate dehydrogenase enzymes, the conductive polymeric matrix accepts an electron from NADH and it transfers its electron back to the electrode [10].
In the present study, cloned Clostridium thermocellum LDH was purified and used as bioagent for preparation of the biosensor electrode. The enzyme was previously immobilized on a gold working electrode for measurement of blood lactate [10]. In this study, the electrode was prepared and its response was analyzed in the presence of mercury and nickel.
2 Experimental
2.1 Chemicals and reagents
Lactate, fructose-1, 6-diphosphate (FDP) and sodium 2 [N-cyclohexylamino] ethane sulfonate (CHES) were from Sigma, and all the other chemicals were Merck grade.
2.2 Purification of LDH
C. thermocellum LDH was cloned into pGEX-4T-2 purification vector previously [10]. In this vector, LDH was combined to Gluthatione S-transferase (GST) gene, therefore the enzyme was purified as linked to GST. Recombinant Escherichia coli FMJ39 cells harbouring the plasmid with C. thermocellum LDH gene were grown in Luria Broth containing 100 mg ampicillin per litter for 24 h. IPTG was added into the culture to a final concentration of 0.3 mol/L at the 10th hour of growth. LDH enzyme was purified from crude extracts by using Sepharose 4B beads as described by the manufacturer (Amersham Life Sciences). For the determination of purified enzyme concentration, denaturing SDS- polyacrylamide gel electrophoresis [11] by bradford method [12] was performed. Proteins were visualized with Commasie blue staining of the gel at the end of electrophoresis.
2.3 Enzyme assays
The enzyme was assayed for lactate oxidation to pyruvate at pH 8.5 as described by CONTAG et al [13]. The reaction was monitored spectrophotometrically at 340 nm to determine the rate of NAD+ reduction to NADH. The assay mixture contained 50 mmol/L sodium 2 [N-cyclohexylamino] ethane sulfonate (CHES), 10 mmol/L NAD+, 1 mmol/L fructose-1, 6-diphosphate (FDP) and 50 mg purified enzyme. The substrate, 50 mmol/L sodium lactate, was added last to start the reaction.
Stock solutions of metals or minerals were prepared with their salts and required quantity of them was included in the assay solution. The salts of metals and minerals used in this study were as follows: Al(NO3)3·9H2O, As2O3, Cu(NO3)2·3H2O, Ba(NO3)2, Hg(NO3)2·H2O, Zn(NO3)2·6H2O, Fe(NO3)3, Co(NO3)2·6H2O, Pb(NO3)2, Mn(NO3)2, Ni(NO3)2·6H2O, SeO2, NH4Cl2, NaNO3, NaNO2, KH2PO4. The concentration ranges of metals and inorganic ions tested in this study were selected according to Water Quality Criteria regulated by U.S. Environmental Protection Agency (EPA) and Ministry of Environment and Forestry of Turkey. Enzyme activity was measured in the presence of six to seven different concentrations for each agent. All the activity measurements were performed in duplicate.
2.4 Calculation of IC50 values of metals
Graphs of initial velocity of the enzyme versus lg[inhibitor] were drawn by using Microsoft Excel program and IC50 values were calculated from the equations obtained from the graphs. The half maximal inhibitory concentration, IC50, shows the functional strength of the inhibitor. It is equal to the inhibitor concentration that reduces the enzyme activity by half.
3 Biosensor preparation
3.1 Electrode preparation and immobilization of lactate dehydrogenase
CHI Model 800B electrochemical analyzer was used for electrochemical experiments. A gold working electrode (1 cm2), a platinum wire counter electrode, an Ag/AgCl (3 mol/L NaCl) reference electrode, and a conventional three-electrode electrochemical cell were purchased from CHI instruments.
PGA solution was prepared as described by KORKUT et al [14]. Polymerization was performed at a potential of 1.0 V for 5 min. Gold plate of 1 cm2 was used for preparation of working electrode. The gold plate coated with PGA-PPY polymeric film was immersed into 25% glutaraldehyde solution for 3 h. The immobilization of enzyme onto glutaraldehyde activated PGA-PPY (poly gluteraldehyde-pyrrole) polymeric film was performed as described by OZKAN et al [10]. The enzyme solution containing 2.5 mg LDH was spread evenly and kept for 24 h at 4 °C for coupling.
3.2 Electrochemical measurements
The above mentioned system was used for electrochemical batch measurements performed at 0.2 V. The working buffer (pH 7.5) contained 50 mmol/L CHES buffer, 10 mmol/L NAD+ and 1 mmol/L FDP. Measurements were carried out at room temperature. I-t curves of chronoamperometric measurements were done by increasing concentrations of lactate. All the experiments were performed in duplicate.
4 Results and discussion
The amperometric biosensors prepared with enzymes are very useful for the determination of substrate or inhibitor with a very low concentration. Various enzymes such as urease, invertase, xanthine oxidase, peroxidase, glucose oxidase or alkaline phosphatase have been used for the determination of heavy metals [16-17]. Effect of some heavy metals on the activity of rabbit LDH enzyme in solution or as immobilized on oxygen electrode had been studied before [8].
Microbial enzymes were reported to be more stable than their corresponding plant and animal enzymes, and their production is more convenient and safer [17]. C. thermocellum is a moderately thermophilic and anaerobic bacterium, and especially important for its very active cellulase enzyme systems. In this study, C. thermocellum LDH previously cloned into pGEX-4T-2 purification vector by OZKAN et al [10] was purified and used for biosensor preparation. The activity of C. thermocellum LDH increases with increasing temperature and the highest activity was observed at 50 °C [18]. The electrode prepared with C. thermocellum LDH was found to retain its activity for a week incubation at refrigerator (+5 °C), and the amperometric measurements showed that the electrode sensitivity increased with increasing the temperature to 60 °C [10]. Stability of the LDH enzyme was studied at different temperatures previously. Enzyme did not lose activity at 65 °C when being incubated for 5 min, but its activity decreased by 63% when being incubated at 75 °C for 5 min [19]. Many thermostable enzymes were cloned and purified for biotechnological applications and biosensor development because of their intrinsically stable structural features [20-22]. Stability is important not only for reusability but also for long shelf life of the prepared electrodes. Use of purified enzymes as bioagent is also an important parameter for increasing the sensitivity of the biosensors [23]. The LDH enzyme used in this study was purified as described in experimental part and 0.07 mg enzyme was obtained from 155 mg E. coli cells. A single protein band belonging to the purified enzyme was observed in SDS PAGE Gel (Fig. 1).
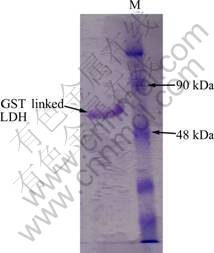
Fig. 1 SDS PAGE gel photograph of purified C.thermocellum LDH (1.63 kDa C. thermocellum LDH-GST band) (M: Marker)
In this study, the activity of LDH enzyme was first measured spectrophotometrically in the presence of different amount of various metals and inorganic minerals. When there was no inhibitor in the assay mixture, the initial velocity was calculated to be 0.18 mmol NADH/(min·mg·L-1). The half inhibitory concentrations (IC50) of the metals were calculated as described in experimental part. The IC50 values for highly inhibitory heavy metals are given in Table 1. Figure 2 shows the equations for the determination of half inhibitory concentrations of Hg2+ and Ni2+. Hg2+, Cd2+, Co2+, Pb2+, Ni2+ and Cu2+ were known to be toxic to different living organisms like yeast, plants and animals or their enzymes [24-26]. In this study, C. thermocellum LDH was found to be highly inhibited by Hg2+, Cd2+ and Pb2+. Percent inhibition values for several other metals or minerals, which are not very toxic to living organisms, were also calculated (Table 2). Inhibition of enzyme activity was no more than 50% when Cu2+, Mn2+, Ba2+ or Al3+ was included in assay mixture at their maximum concentrations. Half inhibitory concentrations of PO4-, NO3-, NH4- were also found to be very high as compared to IC50 values of tested metals. These mineral salts are not strong inhibitors of the enzymes. However, 2.1×10-3 mmol/L NO2- caused 41.3% inhibition of the enzyme activity. Toxicity of nitrite in rats and several aquatic organisms was reported in other studies earlier [27-28].
Table 1 Half inhibitory concentrations of heavy metals for C. thermocellum LDH
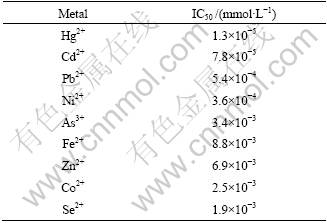
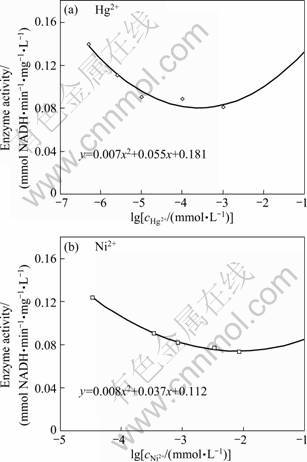
Fig. 2 Equations for determination of IC50 for Hg2+ (a) and for Ni2+ (b)
Amperometric LDH biosensors were prepared previously by immobilization of rabbit or rat lactate dehydrogenases [23, 29] or lactate oxydases [30-31] on different composite films of polypyrrole. However, the response of most of LDH biosensors to heavy metals has not been investigated. In the present study, response of an amperometric biosensor prepared with thermostable purified C. thermocellum LDH to mercury and nickel was tested. The working electrode was prepared as described in experimental section. Relative standard deviation for the electrode was calculated to be 0.32 from eight sequential measurements in the absence of inhibitors. It was observed that, at 22 mmol/L lactate concentration, current decreased from 0.32 ?A to 0.09 ?A when 25×10-7 mmol/L mercury was included in the working solution. The presence of 17×10-5 mmol/L nickel caused a current decrease from 0.41 ?A to 0.26 ?A (Fig. 3). A sharp current decrease observed in the presence of 0.5×10-6 mmol/L Hg2+ and 1.7×10-5 mmol/L Ni2+ indicated the low detection limit of the biosensor. In a study performed by RODRIGUEZ et al [15] the linear range for Hg2+ was determined to be 10-100 ?g/L with a detection limit of 7.2 ?g/L with an amperometric assay based on urease inactivation. In order to woid the problem of irreversable inhibition of enzyme with heavy metals, fresh electrode was used for each test in this study. Irreversable inhibition of rabbit LDH was reported by FENNOUH et al [8]. It was observed that electrode reached steady state valley in less than 10 s. Response time of the electrode can be estimated from the I-t curve shown in Fig. 4.
Enzyme inhibition based biosensors have been widely studied for heavy metal determination. In those studies, response of the biosensor to a specific inhibitory agent was analyzed. However, the presence of a second inhibitory agent found in the sample may cause interference problem and accurate detection of inhibitor can fail. Therefore, the effect of each metal on different responses of the enzyme inhibition-based biosensor should be determined. The antagonistic effect of metals on each other is also an important problem which could lead to inconsistent biosensor responses. There are few studies about the effect of heavy metals on enzyme activity and biosensor performance. GUASCITO et al [5] showed that Cu2+, Cd2+, Co2+ and Ni2+ had lower inhibition effect compared with Hg2+ on glucose oxidase enzyme. However, they also reported that the presence of those metals could interefere with Hg2+ determination with a biosensor prepared with glucose oxidase as bioagent. Sensitivity of an enzyme based biosensor can be defined as ability to detect narrow changes in the concentrations of its substrate. In this study, electrode sensitivity against its substrate (lactate) was determined in the presence of two different concentrations of mercury and nickel. Slope of the lines obtained from the lactate concentration versus current graphics gives sensitivity of the biosensor (Fig. 3). Increase of lactate concentration results in increased current responses of the biosensor. In the present study, the rate of increase, the sensitivity, was found to be different in the presence of mercury and nickel. Electrode sensitivity against lactate was measured as 0.010 2 μA/(mmol·L-1) when no inhibitor is present in the working solution. When 25×10-7 mmol/L mercury was included, sensitivity of the electrode decreased to 0.004 3 μA/(mmol·L-1). Whereas, no decrease was measured in the sensitivity of the electrode when nickel was added in the working solution. This result can be related to the inhibitory concentrations of the metals for LDH enzyme. As shown in Table 1, the half inhibitory concentration of nickel was about 30 times that of mercury. At high concentrations of substrate, the inhibition effect of the less potent heavy metal can be relieved as observed for nickel in Fig. 3(a). This would help the detection of the more potent heavy metal in a sample containing different types of inhibitors.
Table 2 Inhibition of enzyme activity by metals and inorganic ions
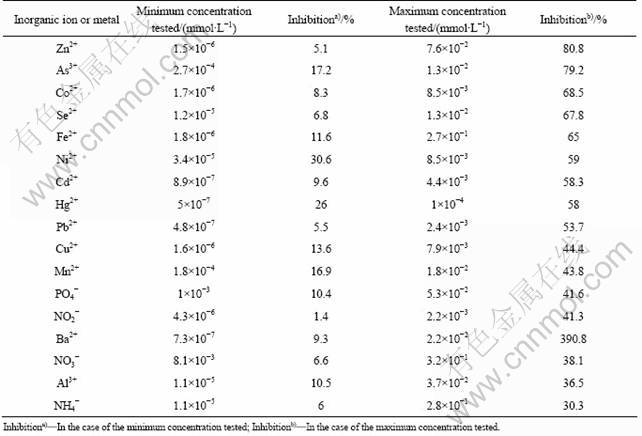
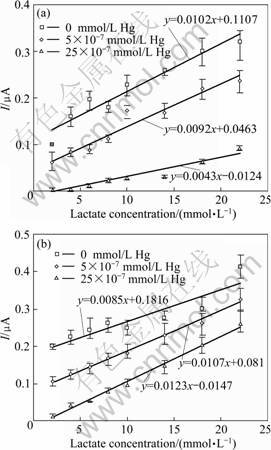
Fig. 3 Effect of mercury (a) and nickel (b) on biosensor response to current
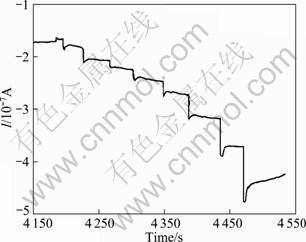
Fig. 4 I-t curve of biosensor obtained in the presence of 1.7×10-5 mmol/L nickel
Various responses of the biosensor such as detection limit, linear range, and sensitivity to the substrate or inhibitors can be evaluated for the identification of the specific inhibitor found in a sample, and concentration determination can be done more precisely with these data. Moreover, responses of more than one working electrodes each prepared with different enzymes (biosensor array) can help solving the interference problem. The possibility of electrochemical biosensor array based on enzyme inhibition was previously mentioned by YOUNG et al [4].
5 Conclusions
1) An amperometric biosensor prepared with a thermostable bacterial LDH enzyme was tested for detection of mercury and nickel in working solution.
2) The biosensor was shown to be able to detect the concentrations of those metals lower than their toxic levels in water samples. Response of the biosensor to increasing concentration of lactate was found to depend on the type of inhibitory metal present in the working solution.
3) Results suggested that the sensitivity of the biosensor to lactate concentrations can be used for the determination of the concentration of mercury in an environmental water sample containing both mercury and nickel together.
Acknowledgements
We thank Dr. ?eyda Korkut ?z?ner for her help in the electrochemical measurements.
References
[1] AmIne A, MohammadI H, BouraIs I, PalleschI G. Enzyme inhibition-based biosensors for food safety and environmental monitoring [J]. Biosensors and Bioelectronics, 2006, 21: 1405-1423.
[2] Cowell D C, Dowman A A, Ashcroft T. The detection and identification of metal and organic pollutants in potable water using enzyme assays suitable for sensor development [J]. Biosensors and Bioelectronics, 1995, 10: 509-516.
[3] Danzer T, Schwedt G. Multivariate evaluation of inhibition studies on an enzyme electrodes system with pesticides and mixtures of mercury and pesticides [J]. Analytica Chimica Acta, 1996, 325: 1-10
[4] Young S J, Hart J P, Dowman A A, Cowell D C. The non-specific inhibition of enzymes by environmental pollutants: A study of a model system towards the development of electrochemical biosensor arrays [J]. Biosensors and Bioelectronics, 2001, 16: 887-894.
[5] GuascIto M R, MalItesta C, Mazzotta E, Turco A. Inhibitive determination of metal ions by an amperometric glucose oxidase biosensor study of the effect of hydrogen peroxide decomposition [J]. Sensors and Actuators B, 2008, 131: 394-402.
[6] Kuralay F, Ozyoruk H, Y?ld?z A. Inhibitive determination of Hg2+ ion by an amperometric urea biosensor using poly (vinylferrocenium) film [J]. Enzyme and Microbial Technology, 2007, 40: 1156-1159.
[7] LIU Jian-xiao, xu Xiang-min, TANG Lin, ZENG Guang-ming. Determination of trace mercury in compost extract by inhibition based glucose oxidase biosensor [J]. Transactions of Nonferrous Metals Society of China, 2009, 19: 235-240.
[8] Fennouh S, Casimiri V, Geloso-Meyer A, Burstein C. Kinetic study of heavy metal salt effects on the activity of L-lactate dehydrogenase in solution or immobilized on an oxygen electrode [J]. Biosensors and Bioelectronics, 1998, 13: 903-909.
[9] Barstow D A, Clarke A R, ChIa W N, WIgley D, Sharman A F, Halbrook C C, AtkInson T, MInton N P. Cloning, expression and complete nucleotide sequence of the Bacillus stearothermophilus L-lactate dehydrogenase gene [J]. Gene, 1986, 46: 47-55.
[10] Ozkan M, Erhan E, Terzi ?, Tan ?, ?z?ner ? K. Thermostable amperometric lactate biosensor with clostridium thermocellum lactate dehydrogenase for measurement of blood lactate [J]. Talanta, 2009, 79: 1412-1417.
[11] LaemmlI U K. Cleavage of structural proteins during the assembly of the head of bacteriophage T4 [J].Nature, 1970, 227: 680-685.
[12] Bradford M M. A rapid and sensitive method for the quantitation of microgram quantities of protein utilizing the principle of protein-dye binding [J]. Analytical Biochemistry, 1976, 72: 248-254.
[13] Contag P R, WIllIams M G, Rogers P. Cloning of lactate dehydrogenase from Clostridium acetobutylicum B463 and expression in Escherichia coli [J]. Applied and Environmental Microbiology, 1990, 56: 3760-3765.
[14] Korkut S. Keskinler B, Erhan E. An amperometric biosensor based on multiwalled carbon nanotube-poly (pyrrole)-horseradish peroxidase nanobiocomposite film for determination of phenol derivatives [J]. Talanta, 2008, 76(5): 1147-1152.
[15] RodrIguez B B, Bolbot J A, TothIll I E. Development of urease and glutamic dehydrogenase amperometric assay for heavy metals screening in polluted samples [J]. Biosensors and Bioelectronics, 2004, 19: 1157-1167.
[16] Shekhovtsova T N, Chernetskaya S V, Belkova N V, Dolmanova I F. The use of immobilised enzymes for the determination of metal ions [J]. Journal of Analytical Chemistry, 1994, 49: 709-714.
[17] Hasan F, Shah A A, Hameed A. Industrial applications of microbial lipases [J]. Enzyme and Microbial Technology, 2006, 39: 235-251.
[18] Assa P, ?zkan M, ?zceng?z G. Thermostability and regulation of Clostridium thermocellum L-lactate dehydrogenase expressed in Escherichia coli [J]. Annals of Microbiology, 2005, 52(3): 193-197.
[19] OZKAN M, YILMAZ E ?, LYND L R, OZCENG?Z G. Cloning and expression of the Clostridium thermocellum L-lactate dehydrogenase gene in Escherichia coli and enzyme characterization [J]. Canadian Journal of Microbiology, 2004, 50(10), 845-851.
[20] Itao T, HIroI T, Amaya T, Kaneko S, ArakI M, Ohsawa T, Yamamura A, Matsumoto K. Preliminary study of a microbeads based histamine detection for food analysis using thermostable recombinant histamine oxidase from arthrobacter crystallopoietes KAIT-B-007 [J]. Talanta, 2009, 77(3): 1185-1190.
[21] Tsuya T, FerrI S, FujIkawa M, Yamaoka H, Sode K. Cloning and functional expression of glucose dehydrogenase complex of Burkholderia cepacia in Escherichia coli [J]. Journal of Biotechnology, 2006, 123: 127-136.
[22] HallIwell C M, SImon E, Toh C S, Bartlett P N, Cass A E G. Immobilisation of lactate dehydrogenase on poly (aniline)–poly (acrylate) and poly (aniline)–poly (vinyl sulphonate) ?lms for use in a lactate biosensor [J]. Analytica Chimica Acta, 2002, 453: 191-200.
[23] LeonIda M D, StarczynowskI D T, Waldman R, AurIan-BlajenI B. Polymeric FAD used as enzyme-friendly mediatorin lactate detection [J]. Analytical and Bioanalytical Chemistry, 2003, 376: 832-837.
[24] Khangarot B S, Das S. Acute toxicity of metals and reference toxicants to a freshwater ostracod, cypris subglobosa Sowerby, 1840 and correlation to EC50 values of other test models [J]. Journal of Hazardous Materials, 2009, 172: 641-649.
[25] Tamas L, Dud?kova J, Durcekova K, Huttova J, MIstr?k I, ZelInova V. The impact of heavy metals on the activity of some enzymes along the barley root [J]. Environmental and Experimental Botany, 2008, 62: 86-91.
[26] Nepov?m A, PodlIpna R, Soudek P, Schroder P, Vanek T. Effects of heavy metals and nitroaromatic compounds on horseradish glutathione S-transferase and peroxidase [J]. Chemosphere, 2004, 57: 1007-1015.
[27] Das P C, Ayyappan S, Jena J K, Das B K. Nitrite toxicity in cirrhinus mrigala (Ham.): Acute toxicity and sub-lethal effect on selected haematological parameters [J]. Aquaculture, 2004, 235: 633-644.
[28] Jensen F B. Uptake, elimination and effects of nitrite and nitrate in freshwater crayfish (Astacus astacus) [J]. Aquatic Toxicology, 1996, 34: 95-104.
[29] Chaubey A, Pande K K, Singh V S, Malhotra B D. Co-immobilization of lactate oxidase and lactate dehydrogenase on conducting polyaniline films [J]. Analytica Chimica Acta, 2000, 407: 97-103.
[30] TrojanowIcz M, KrawczyskIn v e l, Krawczyk T, Geschke O, Cammann K. Biosensors based on oxidases immobilized in various conducting polymers [J]. Sensors and Actuators B, 1995, 28: 191-199.
[31] Gros P, DurlIat H, Comtat M. Use of polypyrrole film containing Fe(CN)63-as pseudo reference electrode: application for amperometric biosensor [J]. Electrochemica Acta, 2000, 46: 643-650.
采用耐热乳酸脱氢酶制备生物传感器来检测汞和镍
?brahim TAN, Elif ERHAN, P?nar KARAG?Z, Melek ?ZKAN
Department of Environmental Engineering, Gebze Institute of Technology, 41400 Kocaeli, Turkey
摘 要:分析了采用耐热细菌LDH酶制备的生物传感器对汞和镍的响应性。将酶纯化、固定在一个涂有PGA-吡咯聚合材料的金箔上作为工作电极,在增加溶液中乳酸浓度的情况下测定生物传感器对两种不同浓度的汞和镍溶液的响应性。当溶液中含25×10-7 mmol/L汞和17×10-5 mmol/L镍时,生物传感器的电流响应分别从0.32 μA下降到0.09 μA和从4.13 μA下降到2.63 μA。在含25×10-7 mmol/L汞溶液中,生物传感器的敏感性从0.010 2 μA/(mmol·L-1) 下降到 0.004 3 μA/(mmol·L-1)。另一方面,镍的存在并没有导致传感器敏感性的降低。结果表明,制备的生物传感器适合用于含汞和镍溶液中的汞检测。
关键词:酶抑制法;生物传感器;重金属
(Edited by YANG Hua)
Corresponding authors: Melek ?ZKAN; Tel: +90-2626053222; Fax: +90-2626053205; E-mail: mozkan@gyte.edu.tr; Elif ERHAN; Tel: +90-26260532217; Fax: +90-2626053205; E-mail: e.erhan@gyte.edu.tr
DOI: 10.1016/S1003-6326(11)61017-0