
Rapid manufacturing of SiC molds with micro-sized holes using abrasive water jet
Bo-Sung SHIN1, Kang-Su PARK1, Yeon-Kyoung BAHK2, Sun-Ki PARK2, Jung-Han LEE2,
Jeung-Sang GO3, Myung-Chang KANG4, Chae-Moon LEE5
1. ERC/NSDM, Pusan National University, Busan, 609-735, Korea;
2. School of Mechanical Engineering, Pusan National University, Busan, 609-735, Korea;
3. Microhole Company, Busan, 609-735, Korea;
4. NCRC, Pusan National University, Busan, 609-735, Korea;
5. Samsung Electro-Mechanics, Busan, 609-735, Korea;
Received 2 March 2009; accepted 30 May 2009
Abstract: Silicon carbide (SiC) is highly wear resistant with good mechanical properties, including high temperature strength, excellent chemical resistance, and high thermal conductivity and thermal shock resistance. SiC molds, which can be produced with diverse microstructural features, are now widely used in glass molding owing to their excellent characteristics, and also have potential applicability in IT industries. SiC molds are traditionally fabricated by silicon micromachining or dicing. The fabrication cost of silicon micromachining is very high, however, because several expensive masks are needed. Furthermore, the fabrication time is very long. Meanwhile, it is difficult to make micro-patterned molds with arbitrary shapes using dicing saws. Abrasive water jet (AWJ) is widely applied to cut and drill very brittle, soft and fibrous materials. It offers high energy density, the absence of a heat affected zone(HAZ), high performance, and an environment friendly process. In spite of these advantages, micro-hole drilling via conventional AWJ processing suffers from notable shortcomings. We proposed a new abrasive supplying method of AWJ. The proposed method reduces frosting phenomena, and provides micro-machining of AWJ. The characteristics of a hole machined was investigated by the proposed AWJ process according to the ratio of water and abrasives. With the optimal experimental conditions, 3×3 array SiC molds with the diameter of 700 μm and depth of 900 μm were successfully manufactured.
Key words: rapid manufacturing; abrasives water jet (AWJ); silicon carbide (SiC); molds; micro-sized hole
1 Introduction
Abrasive water jet (AWJ) machining is commonly used for material cutting, drilling, slotting, and profiling for soft material, multi-layered materials, and advanced materials that are difficult to machine [1-5]. Conventional AWJ offers some advantages. First, the machining thickness by AWJ is thicker than that yielded by a laser. Second, it is possible to cut the material with high reflectivity. Third, brittle materials such as glass and stone can be machined more effectively. Conventional AWJ is a non-contact high-energy density process similar to laser machining, but the kinetic energy, i.e. the machining energy, of abrasives transferred from a high pressure water jet is very low, at about 3% [6].
To improve the momentum transfer efficiency of AWJ machining, an abrasive suspension method was recently developed. This method provides excellent micromachining results. Researches on micro water jet cutting with pre-mixed abrasives, which are injected at ultrasonic speed through a focusing nozzle using an intensifier, have been reported by Miller [6]. He achieved a minimum cutting width of less than several tens of micrometers via a pre-mixed aluminum oxide of several hundreds of nanometers. The micro water jet can save significant amounts of water and abrasive, and also reduce the required pressure compared to a conventional water jet. For example, when a 70 μm consumption is reduced from 300-400 MPa to 70 MPa, from 10 L/h to 1 L/h, and from 2 kg/h to 0.1 kg/h, respectively. However, the maximum cutting speed for 50 μm and 3.0mm titanium specimens is only 400 mm/min and 15 mm/min, respectively. Thus, cutting speed remains limited, and there is a technical limitation for increasing the cutting speed. In addition, PALLEDA [7] reported experimental results on material removal rate (MRR) where the stand-off distance (SOD) and processing pressure were varied for 4 mm thick, brittle glass material. According to the report, increasing the SOD leads a slight increment in MRR, but a reduction of the SOD occurs beyond a certain range. CHEN et al [28]. conducted 64 iterations of experiments by varying the process pressure in a range of 138-345 MPa, transfer speed of 15-50 mm/min, abrasive consumption of 0.575-0.910 kg/min, and a SOD of 2-6 mm in order to determine the optimal AWJ processing conditions on 12.7 mm and 25.4 mm thick alumina ceramics. According to the results, maximum processing depth of approximately 21 mm could be achieved with a water jet orifice diameter of 0.41 mm and a mixing nozzle diameter of 1.27 mm using a garnet abrasive with mesh number 80 for abrasive size at a water jet impact angle of 80?-85?.
In the present study, water jet pressure, the SOD between the nozzle end and work piece, and abrasive mass flow rate, major process parameters in the conventional AWJ method, are studied. Second, a theoretical analysis on abrasive and water jet is conducted to determine the limitations and identify key issues regarding precision machining via conventional AWJ. Finally, to produce micro-sized holes using the proposed AWJ process, which is called the micro-AWJ process, the abrasive flow rate is investigated experimentally and the possibility of producing a SiC mold with micro-sized holes is verified.
2 Theoretical
As shown in Fig.1, the high pressure water that passes the intensifier is injected via the intensifier nozzle in the mixing chamber according to on/off valve operation. When the high pressurized water passes the orifice, abrasives are mixed. Mixture of water and abrasive is accelerated to machine the work piece. Here, the speed of the abrasives should be the same as the velocity of the water in the focusing nozzle. Using the momentum transfer equation, the velocity of abrasive particles (Va) could be determined from Eq.(1) [9].
(1)
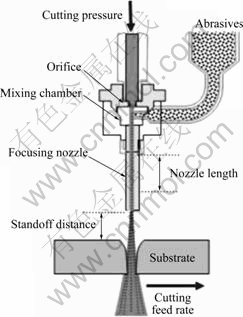
Fig.1 Schematics illustrating AWJ processing
where Vj, ma, mw, and k are the speed at orifice end, the abrasive flow rate, the water flow rate, and the momentum transfer efficiency between water and abrasives, respectively. Under the assumption that water is incompressible and frictional loss is ignored in the system, Vj could be written as Eq.(2) according to Bernoulli’s equation.
(2)
where p and ρw denote the pressure and the density of water, respectively. The speed of water was calculated to be 600-660 m/s when the cutting pressure was 180-220 MPa. Under these conditions, the speed of the abrasives was roughly 180 m/s. However, dry AWJ machining requires air suction while the abrasives pass the orifice of the nozzle. Therefore, frosting phenomena from spreading of the abrasives are unavoidable. This phenomenon is the major cause of failure at the work piece surface. In this study, water was used instead of air to minimize this type of failure. In addition, a micro-hole machining method on the surface of brittle material was proposed. Abrasives were supplied only with water and the speed of the abrasives (
) can be derived from Eq.(3) due to momentum transfer at the mixing nozzle.
(3)
where
is the additional amount of water that is required during supply of the abrasives. If this value becomes larger, the speed of the abrasive particles will be decreased. Therefore, the best machining performance can be achieved when the ratio is optimal.
3 Experimental
A water jet was developed for the experiment. The maximum available pressure was 400 MPa, but the pressure was set at 250 MPa for the experiments. The diameter of the nozzle and orifice is 0.5 and 0.1 mm, respectively. The work piece for the experiment was SiC material, a hard molding material that presents challenges in mechanical machining. SiC material has superior mechanical properties at high temperature, and exhibits additional chemical stability. SiC is widely used in precision glass molding. The properties of SiC obtained from ACM Co., Ltd and used in the experiments are shown in Table 1 [10]. The purity of the SiC is 99.99%-99.999%, and the actual operating temperature is higher than 1 800 ℃ [7]. The abrasive material for this study is alumina with particle size of 90 μm shown in Table 2.
Table 1 Mechanical properties of work piece material, SiC
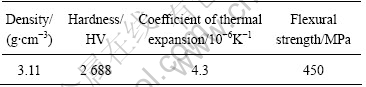
Table 2 Mechanical properties of abrasive material, alumina (Al2O3)
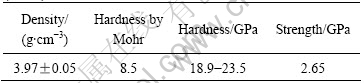
4 Results and discussion
Fig.2 shows high speed camera images taken at the end of the focusing nozzle of a conventional AWJ, where abrasives, air, and water are mixed, and at the end of the nozzle of the proposed AWJ, where abrasives are mixed only with water. As shown in the image, the conventional water jet yielded a wide diameter jet of water, air, and abrasives at a certain distance from the nozzle end. In contrast, the proposed water jet showed a significant reduction of water jet diameter due to the absence of air. This could greatly mitigate the frosting phenomena, and micro-hole machining on hard metal could be realized. In this experiment, the cutting pressure, the orifice diameter, the nozzle diameter, the SOD to the work piece, and the machining time were set at 250 MPa, 0.1 mm, 0.5 mm, 1.2 mm, and 5 s, respectively. With these controlled parameters, the mixing ratios of water and abrasives were varied as 10?0, 10?1, 10?2, 10?3, and 10?4. Drilled cross-sections of SiC with different mixing ratios are shown in Fig.3. It was found that with the increase of mixing ratios the drilled depths increased from 750 μm to 1 200 μm. Diameters of the micro-holes increased from 550 μm to 1 000 μm.
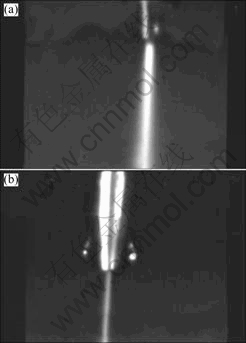
Fig.2 High-speed camera images of water-jet: (a) conventional AWJ; (b) proposed AWJ
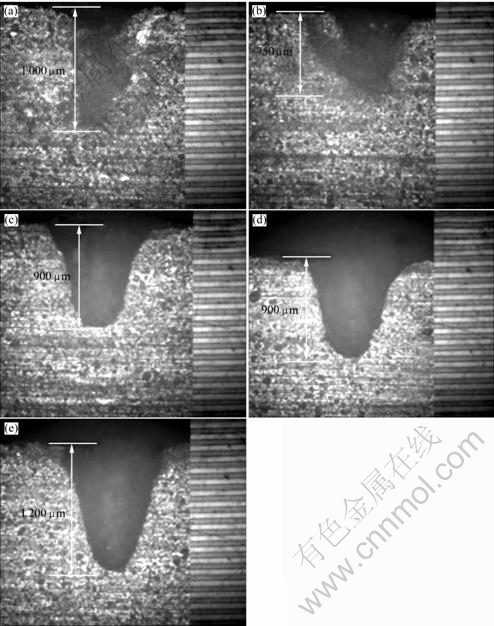
Fig.3 Cross sections of micro-holes with different ratios of water to abrasives: (a) 10?0; (b) 10?1; (c) 10?2; (d) 10?3; (e) 10?4
As shown in Fig.4, a mixing ratio of 10?0 referred drilling only with pure water. This yielded narrow drilling diameter and increased depth, but the drilled side face became excessively rough. As such, this is unacceptable for micro-hole drilling. For a mixing ratio of 10?1, shallow drilling depth with large hole diameter was achieved, which is also unsuitable for micro-hole drilling. When the mixing ratio was changed from 10?2 to 10?3, the drilled depth was maintained at nearly the same level, but the diameter of the micro-hole was slightly increased from 700 μm to 750 μm. For a mixing ratio of 10?4, deeper drilling depth along with a significant increase in hole diameter (up to 1 000 μm) were achieved, which is also inappropriate for micro-hole machining. According to the experimental results, the optimal drilling conditions were obtained at a mixing ratio of 10?2-10?3. As shown in Fig.5, a 3×3 array with a hole diameter of 700 μm and depth of 900 μm was successfully drilled on the surface of the SiC mold.
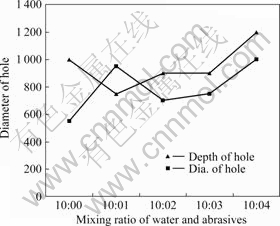
Fig.4 Depth and diameter of micro-hole with mixing ratio of water and abrasives
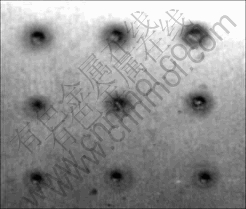
Fig.5 SiC mold with 3×3 holes with depth of 900 μm and diameter of 700 μm
5 Conclusions
Micro-hole drilling was successfully achieved on the surface of SiC, a hard material that is not readily machined by conventional AWJ. From theoretical and experimental results, the following conclusions have been drawn. First, a new abrasive supply method was proposed, where abrasives are mixed only with water, in order to address the frosting phenomena during micro-hole machining. Second, the hole diameter and the drilling depth were increased along with a higher mixing ratio of abrasive and water. Third, the optimal range of the mixing ratio was found to range from 10?2 to 10?3. Finally, a 3×3 array of micro-holes was successfully produced on the surface of a SiC mold using optimal processing conditions, demonstrating the feasibility of rapid manufacturing of SiC patterns with micro-holes. In future research, various SiC molds with micro-hole patterns should be fabricated, and the proposed process should also be applied to micro-glass forming processes.
Acknowledgement
This work was supported by a grant-in-aid for the National Core Research Center Program from the Ministry of Education Science & Technology and the Korea Science & Engineering Foundation (No. R15-2006-022- 01001). The authors would like to thank director S T KIM of ACM Co. Ltd. for assistance with SiC specimens for this study.
References
[1] KHAN A A, HAQUE M M, Performance of different abrasive materials during abrasive water jet machining of glass [J]. J Mater Process Technol, 2007, 191: 404-407.
[2] PARK K S, BAHK Y K, GO J S, SHIN B S. A study on the frosting phenomena of abrasives waterjet microcutting for multi-layered materials [J]. Trans of Korean Society of Machine Tool Engineering, 2007, 16(5): 183-190.
[3] SHANMUGAM D K, WANG J, LIU H. Minimisation of kerf tapers in abrasive waterjet machining of alumina ceramics using a compensation technique [J]. International Journal of Machine Tools & Manufacture, 2008, 48: 1527-1534.
[4] SHANMUGAM D K, NGUYEN T, WANG J. A study of delamination on graphite/epoxy composites in abrasive waterjet machining [J]. Composites: Part A, 2008, 39: 923-929.
[5] WANG J. A new model for predicting the depth of cut in abrasive waterjet contouring of alumina ceramics [J]. J Mater Process Technol, 2009, 209: 2314-2320.
[6] MILLER D S. Micromachining with abrasive waterjets [J]. J Mater Proces Technol, 2004, 149: 37-42.
[7] PALLEDA M. A study on taper angles and material removal rates of drilled holes in the abrasive waterjet machining process [J]. J Mater Process Technol, 2007, 189: 292-295.
[8] CHEN L, SIORES E, WONG W C K. Optimising abrasive waterjet cutting of ceramic materials [J]. J Mater Process Technol, 1998, 74: 251-254.
[9] WANG Jun. Abrasive waterjet machining of engineering materials [M]. Trans Tech Publications, 2003: 62-63.
Corresponding author: Bo-Sung SHIN; Tel: +82-51-510-2787; E-mail: bosung@pusan.ac.kr
(Edited by CHEN Ai-hua)