Trans. Nonferrous Met. Soc. China 25(2015) 497-503
Thermal decomposition of magnesium ammonium phosphate and adsorption properties of its pyrolysis products toward ammonia nitrogen
Yi-qing CHEN1, Jian-jun TANG1, Wen-long LI1, Zhen-hui ZHONG2, Juan YIN1
1. Shenzhen Key Laboratory of Industrial Water Conservation & Municipal Wastewater Resources Technology, Shenzhen Polytechnic, Shenzhen 518055, China;
2. School of Civil Engineering, Guangzhou University, Guangzhou 510006, China
Received 9 June 2014; accepted 29 July 2014
Abstract: High-purity magnesium ammonium phosphate (MAP) was precipitated by controlling pH value of the reaction system of 9.0-9.5. The thermal decomposition behavior of MAP and the adsorption properties of its pyrolysis products toward ammonia-nitrogen were also studied by XRD, SEM, TGA-DTA and FT-IR methods. The results indicated that high-purity MAP was obtained at pH value of 9.0-9.5. Upon heating to 100-120 °C for 120 min, MAP was thermally decomposed, losing water and ammonia concomitantly with a reduction in grain size and crystallinity. The capacity of pyrolysis products for ammonia nitrogen adsorption reached 72.5 mg/g, with a removal rate of up to 95% from an 800 mg/L solution. The characteristic diffraction peaks corresponding to MAP mainly appeared in their XRD patterns after adsorption of ammonia nitrogen. The pyrolysis products of MAP at 100-120 °C could be recycling-used as the chemical treatment regents of ammonia nitrogen in the practical application.
Key words: magnesium ammonium phosphate; magnesium hydrogen phosphate; thermal decomposition; ammonia nitrogen; adsorption properties
1 Introduction
Ammonia nitrogen is a common pollutant that causes eutrophication and environmental damage. In nearly all of China’s contaminated water, ammonia nitrogen is one of the major pollutants at present. The effective and economical treatment of ammonia nitrogen in wastewater is an important objective of environmental research. Frequently used methods for treating ammoniated wastewater include air stripping [1], adsorption and ion exchange [2], biological treatment [3], and chemical sedimentation [4-6]. The latter method is typically simple, using various treatment reagents to produce sediments which are used as fertilizers. Some of these processes have already found application [7,8]. Chemical sedimentation for the removal of ammonia nitrogen from wastewater is based on the following reaction:
HPO42-+Mg2++NH4++6H2O→MgNH4PO4·6H2O+H+(1)
The major limitation for employing the chemical sedimentation of magnesium ammonium phosphate (MAP, MgNH4PO4·6H2O) is the expense of the precipitants (soluble phosphate and magnesium salts), which raises the processing cost. For example, the cost of applying the MAP method to the remediation of landfill leachate was up to 22.9 $/m3 [9].
To solve the cost problems associated with the practical application of MAP chemical sedimentation methods, AHMAD and IDRIS [10] studied the acid regeneration of MAP:
MgNH4PO4·6H2O+H+→MgHPO4·3H2O+NH4++3H2O (2)
HE et al [11] developed a MAP regeneration method under alkaline conditions:
MgNH4PO4·6H2O+NaOH→MgNaPO4(s)+NH3+7H2O (3)
MgNaPO4+NH4++6H2O→MgNH4PO4·6H2O(s)+Na+ (4)
which allowed recycling of the MAP and solved the problem of the precipitating agent cost. However, the acid regeneration method recovered ammonia nitrogen in the form of an ammonium salt from the wastewater, and the recovered solution inevitably contains a small amount of Mg2+ and HPO42- which complicate its discharge. MAP regeneration under alkaline conditions consumes equimolar amount of NaOH, which is economically unsatisfactory, although ammonia nitrogen is recycled in the form of aqueous ammonia from the wastewater.
The use of an ammonia neutralizer generally produces highly concentrated ammonia nitrogen wastewater in the chemical industry, metallurgy, electroplating and other industries. If the ammonia nitrogen pollutant is recovered in the form of aqueous ammonia, it can be directly reused, finally forming ammonia to close the circuit. This process should be regarded as the best solution for ammonia nitrogen wastewater treatment.
The study of BHUIYAN et al [12] showed that MAP can be thermally decomposed into a mixture of MgHPO4, Mg3(PO4)2 and Mg2P2O7. SUGIYAMA et al [13] indicated that the thermal decomposition product of struvite had adsorption capabilities; the adsorption properties of MgHPO4 for NH4+ were better than those of Mg3(PO4)2, whereas Mg2P2O7 hardly adsorbed NH4+. MAP could be employed as an advanced material for the removal/recovery of ammonium. ZHAO et al [14] investigated the formation of MAP from MHP and NH4+ by an exchange reaction under suitable pH conditions. Highly concentrated ammonia solutions could be recovered and the MHP adsorbent could be easily regenerated by heat decomposition of the MAP. This process is an effective method that recycles not only the ammonia but also the MHP. Theoretically, the steam released from the pyrolysis of MAP can generate 13.6% aqueous ammonia (mass fraction), which can fully meet the requirements of industrial processes. It is suggested that the thermal decomposition of MAP under certain conditions can produce highly concentrated aqueous ammonia, but also pyrolysis products that may be used to treat ammonia nitrogen wastewater. This would allow recycling and reduce the costs of the ammonia nitrogen wastewater treatment agent.
The thermal decomposition of MAP can be conducted stepwise, and the temperature determines the composition of the pyrolysis products, thereby affecting their NH4+ adsorption properties. In this work, the thermal decomposition process of MAP and the adsorption properties of its pyrolysis products toward ammonia nitrogen were investigated.
2 Experimental
2.1 Preparation of MAP
Ammonia-containing simulated wastewater (2000 mg/L, 200 mL) was prepared in a 250 mL conical flask. Sufficient Na2HPO4·12H2O and MgCl2·6H2O were added sequentially to achieve a N/P/Mg molar ratio of 1: 1: 1. The solution was stirred with a temperature-controlled magnetic stirrer, and the pH value was adjusted with 5 mol/L NaOH. The resulting precipitates were filtered after 20 min. The filtrate was analyzed for the remaining ammonia nitrogen and total phosphorus content, and the MAP sediments after washing were dried in a thermostatted blast oven at 40 °C for 48 h.
2.2 Thermal decomposition of MAP
The prepared MAP was placed in a tubular resistance furnace and pyrolyzed at 100 °C (unless otherwise stated) for 120 min. The pyrolysis products were finely ground and then sealed for later use.
2.3 Adsorption of ammonia nitogen
Ammonia-containing simulated wastewater (800 mg/L, 200 mL) was prepared with NH4Cl (AR). The MAP pyrolysis product (4 g) was added, and the mixture was magnetically stirred at room temperature for 40 min after adjusting the pH value to ~10 with 5 mol/L NaOH. After the reaction was completed, the mixture was separated using a Hitachi CR22G high-speed refrigerated centrifuge. The supernatant was used to analyze the ammonia nitrogen concentration, and the solid precipitate was treated as described in Section 2.1.
2.4 Testing and characterization
The ammonia nitrogen concentration was analyzed by Nessler’s reagent spectrophotometry. Phosphate concentration was determined by ammonium molybdate spectrophotometry. Atomic absorption spectro- photometry was used to analyze Mg concentration (Shimadzu AA-6300F). Thermogravimetric-differential thermal analysis (TGA-DTA) studies were conducted by a TGA-DTA 6200 analyzer for a sample with mass of 27.5 mg, at a heating rate of 10 °C/min, and a 100 mL/min of air flow. Infrared spectroscopy was analyzed by a Shimadzu FTIR-8400S Fourier transform infrared spectrophotometer, and KBr tablet method was used for measuring absorption spectrum. Powder morphology analysis was conducted by scanning electron microscopy (SEM, JEOL JSM-6700F). A Panalytical Pert Pro X-ray diffractometer was used to obtain X-ray diffraction (XRD) patterns at room temperature, using a Cu Kα source at 40 kV and 40 mA. Particle sizes were determined with a Microtrac S3500 laser particle size analyzer (Microtrac, USA).
3 Results and discussion
3.1 Preparation and characterization of MAP
The recovery of MAP by the chemical sedimentation method (Eq. (1)) illustrates the factors which affect MAP precipitation: the types and ratio of precipitant agents, pH, initial concentration of ammonia nitrogen, etc. To obtain high purity MAP for thermal decomposition and the ammonia adsorption experiments of its pyrolysis products, the initial ammonia nitrogen concentration should be 2000 mg/L, and the precipitation agents Na2HPO4·12H2O and MgCl2·6H2O relative to ammonia should be added at a N/P/Mg molar ratio of 1:1:1. The preparation of MAP involves complex systems such as Mg2+-PO43--NH4+-H+ and the main factor affecting the purity of the MAP is the pH value of the reaction system [15]. Thus, we investigated the effect of pH value of the reaction system on the preparation of MAP.
Figure 1 shows that the rate of MAP precipitation significantly increases with the increase of pH value when the pH value ranges from 6.5 to 9.0. However, the rate of precipitation sharply decreases when pH values are greater than 10.0. The particle sizes (D50) of the precipitates obtained at different pH values were measured using a laser particle size analyzer, and the results are shown in Table 1. The size distribution curve of MAP at pH value of 9.5 is shown in Fig. 2. Clearly, significant changes occur in the particle diameters of the precipitates with changes in pH value.
Theoretical calculation shows that phosphorus mainly exists in the form of H2PO4- when the pH value of the reaction system is less than 8, which is not conducive to the generation of MAP. Furthermore, the phosphorus and magnesium salts easily form the insoluble compound Mg3(PO4)2 when the pH value is greater than 10, as well as Mg(OH)2 when at pH value above 11. Therefore, a reaction system with pH in the range of 8-10 is conducive to the formation of MAP, which is consistent with the experimental results shown in Fig. 1. Because MAP is more easily formed in the pH range of 9.0-9.5, which can be seen from Fig. 1, its particle sizes (30-50 μm) are larger in this range. However, when the pH value exceeds 10, the median diameter (D50) changes and the precipitate includes MAP, Mg3(PO4)2, Mg(OH)2, and other inclusions. Therefore, a pH value in the range of 9.0-9.5 was selected for subsequent MAP preparation experiments.
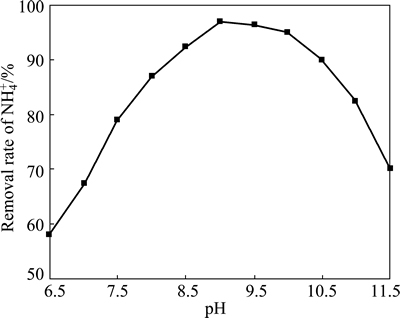
Fig. 1 Relationship between removal rate of NH4+ and pH value
Table 1 Sizes of MAP produced at different pH values

Figure 3 shows the SEM image of the MAP precipitate. The material is rhomboid, uniform, and well-dispersed. The sharp peaks in the XRD patterns in Fig. 4 indicate the precipitate’s high crystallinity, which is consistent with the main absorption peaks and height of MAP standard pattern. The recovered MAP was dissolved in highly concentrated HCl for chemical composition analysis. The N/P/Mg molar ratio is 1.00:1.03: 1.00, which is consistent with the theoretical formula composition and indicative of its high purity.
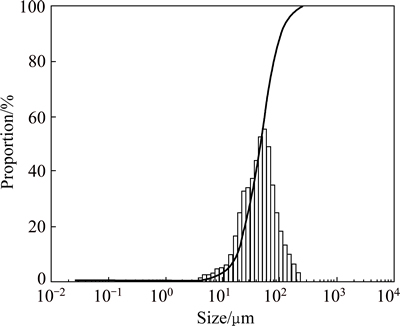
Fig. 2 Size distribution curve of MAP produced at pH 9.5
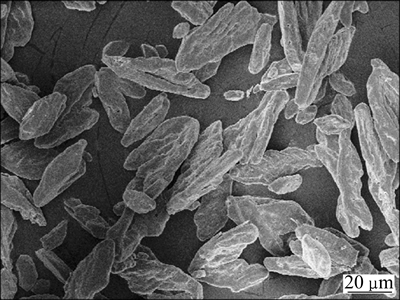
Fig. 3 SEM image of MAP
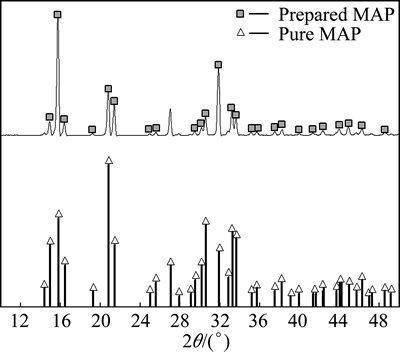

Fig. 4 XRD patterns of MAP
3.2 Thermal decomposition of MAP
The stepwise thermal decomposition of MAP mayinclude the following steps:
MgNH4PO4·6H2O→MgNH4PO4·H2O+5H2O↑ (5)
MgNH4PO4·6H2O→MgHPO4·3H2O+3H2O↑+NH3↑ (6)
3MgNH4PO4·6H2O→Mg2P2O7+MgHPO4+18H2O↑+3NH3↑ (7)
2MgHPO4→Mg2P2O7+H2O↑ (8)
To understand the process of MAP pyrolysis, the TGA-DTA analysis of prepared MAP was carried out. The results are shown in Fig. 5. The DTA curve of MAP displays a distinct endothermic peak at ~105 °C, which may be attributed to the loss of water of crystallization and ammonia gas discharge. From the TGA curve, it can be seen that the mass of the MAP gradually decreases with increasing temperature, and sharply declines between 60 °C and 120 °C, which is caused by the dehydration and the release of ammonia. However, the TGA curve levels off after 120 °C, indicating that the removal of water and ammonia is essentially completed.
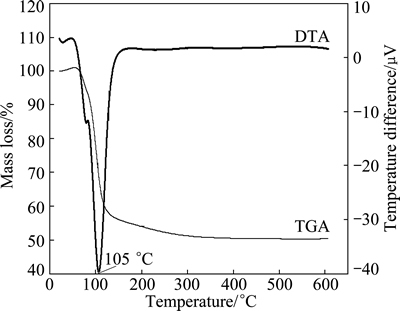
Fig. 5 TGA-DTA curves of MAP
The MAP thermal decomposition steps above manifest that MAP will theoretically lose 51% of its mass if the decomposition product is MgHPO4, or 55% if the product is Mg2P2O7. The mass loss from MAP as shown in the TGA curve is basically consistent with the theoretical calculation.
Figure 6 presents the FT-IR spectra of MAP and its pyrolysis products at different temperatures. With increasing the pyrolysis temperature of MAP, vibrations of the characteristic ammonium bands between 1385 and 1500 cm-1 are the strongest and the relative intensities of absorption peaks for crystal water deformation vibrations at 1640 cm-1 significantly decrease, that is to say, water and ammonia can be released by pyrolyzing MAP, as shown in Eqs. (5)-(7). In addition, phosphate symmetric stretching vibrations at 1070 cm-1 almost maintain the relative intensity; however, when the pyrolysis temperature is raised to 600 °C, P—O—P asymmetric stretching vibrations appear at 890-940 cm-1, namely, P2O74- is generated at this point, as shown in Eq. (8).
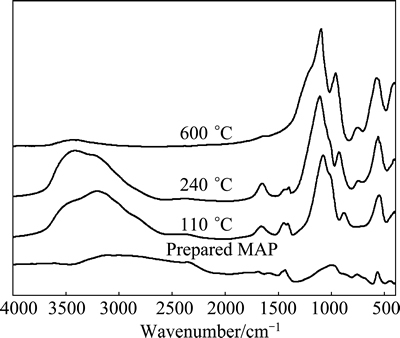
Fig. 6 FT-IR spectra of MAP and its pyrolysis products at different temperatures
Figure 7 presents the XRD patterns of the products obtained from MAP pyrolysis at different temperatures. Under condition of MAP pyrolysis at 100 °C for 120 min, the starting MgNH4PO4·6H2O mainly decomposed into MgHPO4·3H2O due to the low temperature. However, some characteristic diffraction peaks of NH4MgPO4·H2O were also detected. Thus, dehydration and ammonia release were not complete under this condition. Characteristic diffraction peaks of NH4MgPO4·H2O and MgHPO4·3H2O did not appear in the XRD pattern when the pyrolysis temperature was raised to 140 °C, but there were a small amount of diffraction peaks that were characteristic of Mg3(PO4)2·10H2O, as well as miscellaneous others. All the diffraction peaks disappeared when the pyrolysis temperature was raised to 180 °C, indicating the formation of an amorphous, non-crystalline product. Figures 5-7 show that the MAP thermal decomposition temperature should be controlled within the range of 60-180 °C.
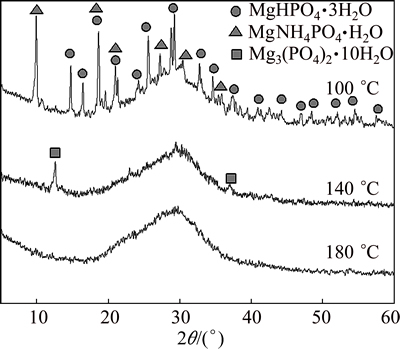
Fig. 7 XRD patterns of pyrolysis products of MAP at different temperatures
It can also be found from experimental results that the degree of crystallinity of the MAP pyrolysis products was greatly reduced, accompanied by significant changes in morphology and reduced particle size. In Fig. 8, the characteristic X-ray diffraction peaks of MgHPO4·3H2O disappeared after the products of MAP pyrolysis at 100 °C were treated with ammonia nitrogen. They were replaced by peaks attributable to MAP, showing that the MgHPO4 in the pyrolysis product could adsorb ammonia nitrogen and regenerate MAP. Thus, the pyrolysis of MAP between 60 and 180 °C releases ammonia and generates solid products that can also be used as treatment agents for ammonia nitrogen wastewater.
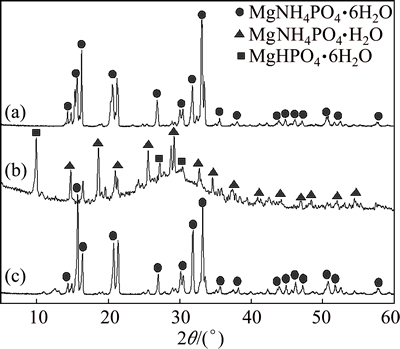
Fig. 8 XRD patterns of MAP (a), MAP pyrolysis products (b), and MAP pyrolysis products after absorption of ammonia nitrogen (c)
3.3 Adsorption of ammonia nitrogen
Table 2 and Fig. 9 reveal the effects of pyrolysis temperature on the adsorption properties of the MAP pyrolysis products for ammonia nitrogen. The pyrolysis products formed at 60 °C demonstrated an adsorption capacity for ammonia nitrogen of 34.1 mg/g, and the removal rate of ammonia nitrogen with an initial concentration of 800 mg/L reached 56%. Thus, the MAP pyrolyzed at 60 °C released a part of its ammonia nitrogen and formed MgHPO4. The adsorption capacity for ammonia nitrogen of the pyrolysis products generated at 120 °C significantly increased to 72.5 mg/g, and the removal rate of ammonia nitrogen exceeded 95%. However, if the pyrolysis temperature was further increased, the adsorption capacities of the pyrolysis products significantly declined. For example, the pyrolysis products generated at 250 °C hardly absorbed ammonia nitrogen.
Table 2 Absorption capacity of MAP pyrolysis products for ammonia nitrogen at different temperatures
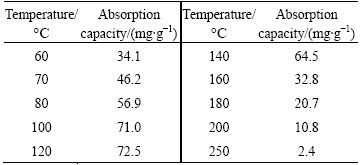
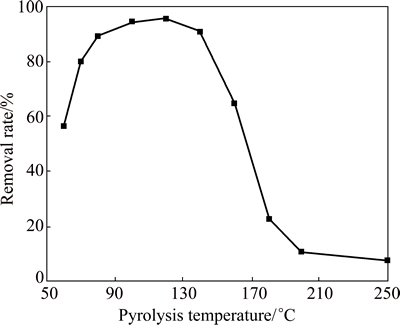
Fig. 9 Influence of pyrolysis temperature on removal rate of ammonia nitrogen by MAP pyrolysis products
The experimental results in Table 2 and Fig. 9 are consistent with the TGA-DTA data in Fig. 5, the FT-IR patterns in Fig. 6 and the XRD results in Fig. 7. Ammonia was released and MgHPO4 that could adsorb ammonia nitrogen would be produced upon MAP pyrolysis at low temperatures, whereas at a pyrolysis temperature of 200 °C, ammonia nitrogen was released, but the pyrolysis product was Mg2P2O7. The latter hardly absorbed ammonia nitrogen. Therefore, MAP should be pyrolyzed between 100 and 120 °C to be used as a practical treatment agent for ammonia nitrogen.
The XRD patterns for the three samples in Fig. 10 show only the characteristic diffraction peaks of MAP after repeating pyrolysis and ammonia nitrogen adsorption treatment cycles. This confirms that by controlling a certain temperature (100-120 °C) of MAP pyrolysis, the pyrolysis products are fully recyclable for reusing as processing agents for ammonia nitrogen.
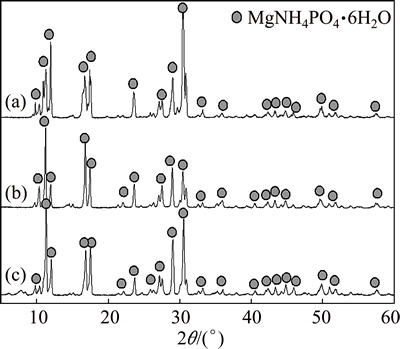
Fig. 10 XRD patterns of MAP powders before use (a), after one cycle (b) and five cycles (c)
4 Conclusions
1) High-purity MAP can be obtained by controlling the reaction system pH value at 9.0-9.5. The MAP precipitates have relatively uniform particle sizes in the range of 30-50 μm.
2) The grain size and crystallinity of the pyrolysis products simultaneously decrease when MAP is pyrolyzed between 100 and 200 °C for 120 min. In contrast, the adsorption properties for ammonia nitrogen are improved. The adsorption capacity reaches 72.5 mg/g for the sorbent pyrolyzed at 120 °C, and the removal rate of ammonia nitrogen at an MAP initial concentration of 800 mg/L is as high as 95%.
3) MAP pyrolyzed under appropriate conditions not only releases ammonia but yields MAP-based pyrolysis products that can be recycled for reusing in the further chemical treatment of ammonia nitrogen. This practical method is expected to solve the shortcomings of traditional MAP-based chemical sedimentation methods in the treatment of ammonia nitrogen wastewater. Theoretically, the ammonia nitrogen pollutant is recovered as aqueous ammonia which is a potential resource. This approach is squarely in line with the concept of a circular economy that China makes efforts to promote.
References
[1] CHEUNG K C, CHU L M, WONG M H. Ammonia stripping as a pretreatment for landfill leachate [J]. Water, Air, and Soil Pollution, 1997, 94: 209-221.
[2] LI P, WANG Q H, ZENG T L, XIE W M. Organic matter and ammonia removal and adsorption ability of three kinds of carriers [J]. Technology of Water Treatment, 2012, 38: 17-20. (in Chinese)
[3] OBAJA D, MACE S, MATA-ALVAREZ J. Biological nutrient removal by a sequencing batch reactor (SBR) using an internal organic carbon source in digested piggery wastewater [J]. Bioresource Technology, 2005, 96: 7-14.
[4] STRATFUL I, SCRIMSHAW M D, LESTER J N. Conditions influencing the precipitation of magnesium ammonium phosphate [J]. Water Research, 2001, 35: 4191-4199.
[5] ZOU A H, SUN T C, SONG C Y, XING Y, GUO S H. Recovery of magnesium ammonium phosphate from ammonia nitrogen wastewater by chemical precipitation [J]. Journal of University of Science and Technology Beijing, 2007, 29: 562-564. (in Chinese)
[6] YU R T, REN H Q, WANG Y R, DING L L, GENG J J, XU K, ZHANG Y. A kinetic study of struvite precipitation recycling technology with NaOH/Mg(OH)2 addition [J]. Bioresource Technology, 2013, 143: 519-524.
[7] UYSAL A, KURU B. Magnesium ammonium phosphate production from wastewater through Box-Behnken design and its effect on nutrient element uptake in plants [J]. Clean-Soil Air Water, 2013, 41: 447-454.
[8] LATIFIAN M, HOLST O, LIU J. Nitrogen and phosphorus removal from urine by sequential struvite formation and recycling process [J]. Clean-Soil, Air, Water, 2013, 42: 1157-1161.
[9] GUNAY A, KARADAG D, TOSUN I, OZTURK M. Use of magnesit as a magnesium source for ammonium removal from leachate [J]. Journal of Hazardous Materials, 2008, 156: 619-623.
[10] AHMAD A A, IDRIS A. Release and recovery of phosphorus from wastewater treatment sludge via struvite precipitation [J]. Desalination and Water Treatment, 2014, 52: 5696-5703.
[11] HE S L, ZHANG Y, YANG M, DU W L, HARADA H. Repeated use of MAP decomposition residues for the removal of high ammonium concentration from landfill leachate [J]. Chemosphere, 2007, 66: 2233-2238.
[12] BHUIYAN M I H, MAVINIC D S, KOCH F A. Thermal decomposition of struvite and its phase transition [J]. Chemosphere, 2008, 70: 1347-1356.
[13] SUGIYAMA S, YOKOYAMA M, ISHIZUKA H, SOTOWA K I, TOMIDA T, SHIGEMOTO N. Removal of aqueous ammonium with magnesium phosphate obtained from ammonium elimination of magnesium ammonium phosphate [J]. Journal of Colloid and Interface Science, 2005, 292: 133-138.
[14] ZHAO T, ZHOU K G, WANG H, LIU R Y. Study on optimal conditions of absorbing ammonia-nitrogen in wastewater with MHP [J]. Technology of Water Treatment, 2008, 34: 69-72. (in Chinese)
[15] JIANG K, ZHOU K G, PENG J L. Thermodynamics of solid-liquid equilibrium in NH4+-Mg2+-PO43-H+-H2O system [J]. Journal of Central South University: Science and Technology, 2009, 40: 1178-1182. (in Chinese).
磷酸铵镁的热分解行为及热分解产物的氨氮吸附性能
陈益清1,唐建军1,李文龙1,钟振辉2,尹 娟1
1. 深圳职业技术学院 深圳市工业节水与城市污水资源化技术重点实验室,深圳 518055;
2. 广州大学 土木工程学院,广州 510006
摘 要:通过控制反应体系的pH值为9.0~9.5,制得高纯度的磷酸铵镁(MAP);利用XRD、SEM、TGA-DTA及FT-IR等技术,研究MAP的热分解行为及热解产物对氨氮的吸附性能。结果表明,在pH值为9.0~9.5的条件下可制得高纯度的MAP。将MAP在100~120 °C下热解120 min,可将氨及水脱除,热解固体产物粒径变小,结晶度降低,对氨氮的吸附量达72.5 mg/g,对起始浓度为800 mg/L溶液的氨氮去除率达95%以上。吸附氨氮后,其XRD谱中主要出现MAP的特征衍射峰。循环使用结果表明,将MAP在100~120 °C条件下发生热解,热解固体产物可循环用于氨氮的处理药剂。
关键词:磷酸铵镁;磷酸氢镁;热分解;氨氮;吸附性能
(Edited by Wei-ping CHEN)
Foundation item: Project (ZDSY20120619093952884) supported by Shenzhen Strategic New Industry Development, China
Corresponding author: Jian-jun TANG; Tel: +86-755-26731648; E-mail: tangjj7384@sina.com
DOI: 10.1016/S1003-6326(15)63630-5