J. Cent. South Univ. Technol. (2008) 15: 214-217
DOI: 10.1007/s11771-008-0041-8

Synthesis and electrochemical properties of SnO2-polyaniline composite
HE Ze-qiang(何则强)1, 2, XIONG Li-zhi(熊利芝)1,2, LIU Wen-ping(刘文萍)1,
WU Xian-ming(吴显明)1, CHEN Shang(陈 上)1, HUANG Ke-long(黄可龙)2
(1. College of Chemistry and Chemical Engineering, Jishou University, Jishou 416000, China;
2. School of Chemistry and Chemical Engineering, Central South University, Changsha 410083, China)
Abstract: The SnO2-polyaniline(SnO2-PAn) composite was prepared by microemulsion polymerization method using aniline, ammonium peroxodisulfate and SnO2 as starting materials. The SnO2-PAn composite was characterized by X-ray diffractometer, scanning electron microscope and electrochemical techniques. The results show that PAn in the composites is amorphous. PAn formed in the reaction is deposited preferentially on the SnO2 particles, giving a SnO2-PAn composite, in which SnO2 is coated with PAn. SnO2-PAn composite shows a reversible capacity of 657.6 mA?h/g and the capacity loss per cycle is only 0.092% after 80 cycles, suggesting that SnO2-PAn composite is a promising anode material for lithium ion batteries.
Key words: lithium ion battery; synthesis; electrochemical properties; microemulsion polymerization method; SnO2; polyaniline
1 Introduction
Recently, tin oxide and tin-based composite oxide electrodes have been investigated as possible anode electrodes for the fourth generation lithium-ion batteries due to the desirable material properties of high capacity on both a gravimetric and a volumetric basis and the low potential of Li ion intercalation[1-5]. However, there exist large volume expansion and contraction problems associated with the Li+ insertion and removal reactions, respectively. Thus, mechanical stresses, related to the volume charges, induce a rapid decay in mechanical stability. The electrode suffers from cracking and crumbling (pulverization), as well as from consequent loss of electrode interparticle contact, resulting in loss of capacity[6-8]. Tin or tin oxide was combined with graphite and carbon in an attempt to reduce capacity fade. The approach has been to prevent the tin from becoming electrically disconnected through the use of a conductive matrix[9-13]. Recently, the conducting polymer has been studied as an additive to improve the performances of cathode and anode materials in lithium ion batteries[14-16]. The conducting polymer composites exhibit good charge-discharge properties, indicating clearly that the conducting polymer can work as a conducting matrix for the particles of electrode. ZHANG et al[17] reported the nano-tin-polyaniline lithium ion composite anode and SCHNITZLER et al[18] reported the synthesis and characterization of new organic/inorganic hybrid materials formed from the mixed oxide (Ti,Sn)O2 nanoparticles and polyaniline (PAn). However, using polyaniline as an additive for a tin-oxide based anode material in lithium-ion batteries has not been explored. In this work, SnO2-polyaniline (SnO2-PAn) composite was synthesized using a microemulsion polymerization method. The electrochemical properties of SnO2-PAn composite as an anode material for lithium-ion batteries were examined.
2 Experimental
0.5 g sodium dodecyl benzene sulfonate (C18H29- SO3Na) was dissolved in 100 mL deionized water, in which pH value was adjusted to about 1-2 by dropping 0.1 mol/L HCl. 22.5 g aniline was slowly added dropwise into the solution under strong stirring. The solution was ultrasonically treated for 30 min, and then a specific mass of SnO2 powder (the molar ratio of SnO2 to aniline (n(SnO2)?n(aniline)) was 2?5) and 10 mL n-butyl alcohol (C4H9OH) was added under strong stirring[19]. The stirring was lasted for 45 min to obtain a micro emulsion liquid, and the solution was cooled with ice-water. A certain amount of ammonium peroxydisulfate (APS, (NH4)2S2O8) was dissolved in 100
mL deionized water in terms of n(aniline)?n(APS)=1?1
and added dropwise to the micro emulsion in 1 h to form green polyaniline. The reaction temperature was kept at 10 ℃. After 6 h, the resulting green dispersion was centrifuged. The resulting SnO2-PAn composite was washed thoroughly by deionized water, ethanol and acetone for several times, and then dried at 60 ℃ under vacuum.
Phase identification and surface morphology studies of the samples were carried out by an X-ray diffractometer (XRD; Rigaku D/MAX-gA) with Cu Kα radiation and scanning electron microscope (SEM, JSM 5600LV).
A slurry containing 80% SnO2-PAn composite, 10% PVDF (polyvinylidene fluoride) and 10% acetylene black (mass fraction) was made using N-methylprrolidinone(NMP) as the solvent. The electrodes with an area of 1 cm2 were prepared by coating the slurry (about 100 μm in thickness) on copper foils, followed by drying in vacuum at 60 ℃ for 12 h. Electrochemical tests were performed using a conventional coin-type cell, employing lithium foil as a counter electrode and 1.0 mol/L LiPF6 in ethylene carbonate/dimethyl carbonate (EC/DMC) (with volume ratio of EC to DMC was 1?1) as the electrolyte. The assembly was carried out in an Ar-filled glove box. The electrochemical tests were carried out with an electrochemical work station(CHI660B, Shanghai, China).
3 Results and discussion
Fig.1 shows the XRD patterns of SnO2, PAn and SnO2-PAn composite.
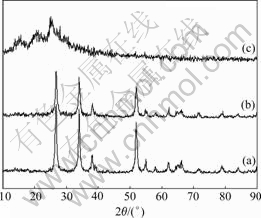
Fig.1 XRD patterns of samples: (a) SnO2; (b) SnO2-PAn; (c) PAn
The XRD pattern of SnO2 agrees well with JCPDS 41-1445, suggesting that the prepared powder is pure SnO2 with single crystalline of tetragonal (p42/mnm) structure. The XRD diffraction peaks of SnO2 are somewhat wide, indicating that the particle size of SnO2 is small. The XRD pattern of SnO2-PAn composite assembles well with that of SnO2, in which the XRD peaks of PAn cannot be found, suggesting that the PAn in the composites is amorphous. The PAn formed in the reaction is deposited preferentially on the SnO2 particles, giving a SnO2-PAn composite, in which SnO2 is coated with the PAn[20].
Fig.2 shows the SEM images of SnO2 and SnO2-PAn composite. It can be seen from Fig.2(a) that the SnO2 powder is composed of spherical particles with size of 15-20 nm. Due to the high activity of nano- particles, there are some agglomerates. After coating, the porous nanosized PAn particles are uniformly coated on the surface of the SnO2 particles, as seen in Fig.2(b). The layer of conductive PAn particles may improve the contact of Sn particles in the electrode reaction process and enhance the cycling performance of nano-SnO2[21]. In order to verify the improvement of PAn coating layer, the conductivities of nano-SnO2, PAn and SnO2-PAn composite were tested. The results show that the conductivity of nano-SnO2, PAn and SnO2-PAn composite are 2.28×10-4, 0.11 and 0.24 S/cm, respectively, suggesting that the conductivity of SnO2 is improved by coating with PAn.
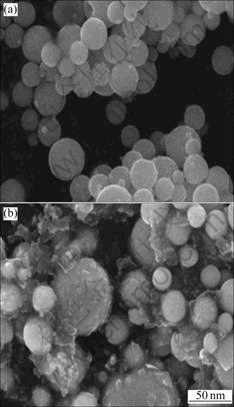
Fig.2 SEM images of samples: (a) SnO2; (b) SnO2-PAn composite
The first discharge-charge curves of nano-SnO2 and SnO2-PAn composite are shown in Fig.3. It can be seen that the dischrge-charge curves of nano-SnO2 and SnO2-PAn composite are very similar, indicating that they have the same mechanism of lithium storage[22-24]. Two obvious plateaus appear on the first discharge curve. The first one is at 0.9 V, which corresponds to the reaction between SnO2 and Li, leading to the dissociation of SnO2 and the formation of solid electrolyte interface (SEI) film on the surface of electrode. The second appears at 0-0.5 V, which is associated with the alloying reactions between Sn and Li. The only plateau at 0-0.5 V during charging corresponds to the de-alloying reactions of LixSn (0≤x≤4.4), i.e, the deinsertion process of lithium ion.
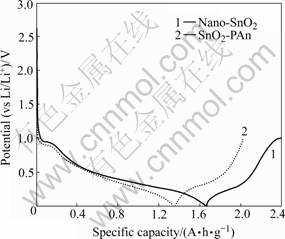
Fig.3 The first discharge-charge curves of different anodes
By comparing the discharge-charge curves of SnO2 and SnO2-PAn composite, it is found that SnO2-PAn composite has lower capacity than nano-SnO2. This may be caused by the decrease of active material in the SnO2-PAn electrode due to the introduction of PAn.
The cycling performance of nano-SnO2 and SnO2-PAn composite is shown in Fig.4. Cycling at 0.1C (70 mA/g), the initial specific capacity of nano-SnO2 is 702.1 mA?h/g, 577.5 mA?h/g of that is retained after 80
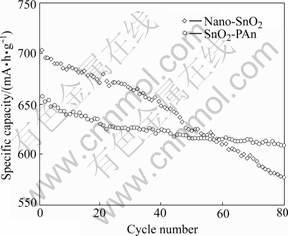
Fig.4 Cyclability of different anodes
cycles, and the capacity loss per cycle is 0.22%. Under the same conditions, the specific capacity of SnO2-PAn composite is 657.6 mA?h/g, 609.3 mA?h/g of that can be retained after 80 cycles, the capacity loss per cycle is only 0.092%. It is obvious that SnO2-PAn composite shows better cycling performance than nano-SnO2, which is due to the improvement of electrical contact between SnO2 particles after being coated with PAn[21].
Fig.5 shows the AC impedance diagrams of nano-SnO2 and SnO2-PAn anodes after the 80th cycle operated in the voltage range from 0 to 1.0 V. The frequency range is changed from 1×10-3 to 1×106 Hz and current rate is 0.1C (70 mA/g). A typical semicircle and an inclined line are seen in both the figures of nano-SnO2 and SnO2-PAn. The high frequency arc is attributed to the charge transfer reaction at the interface of electrolyte and electrode[25]. The inclined line corresponds to Warburg impedance related to the diffusion of lithium ion in the anode[26]. By comparing the AC impedance diagram of SnO2 and SnO2-PAn composite, it is easily found that SnO2-PAn composite shows lower charge transfer resistance, indicating that the conductive PAn coated on the surface of SnO2 may improve the electrochemical kinetics of SnO2 and accelerate the diffusion rate of lithium ion.
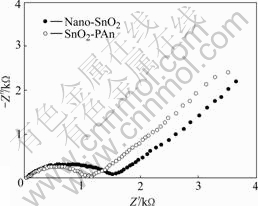
Fig.5 AC impedance diagrams of different anodes
4 Conclusions
1) The PAn formed in the reaction is deposited preferentially on the SnO2 particles to obtain a SnO2-PAn composite, in which SnO2 is coated with amorphous PAn.
2) The conductive PAn can improve the electro- chemical kinetics of SnO2 and accelerate the diffusion rate of lithium ion and thus improve the electrochemical properties of SnO2.
3) SnO2-PAn composite may be a promising anode material for lithium ion batteries with high specific capacity and good cycling stability.
References
[1] IDOTA Y, MATSUFUJI A, MAEKAWA Y, NIYASAKA T. Tin-based amorphous oxide: A high-capacity lithium-ion-storage material[J]. Science, 1997, 276(5317): 1395-1397.
[2] COURTNEY A, DAHN J R. Electrochemical and in situ X-ray diffraction studies of the reaction of lithium with tin oxide composites[J]. J Electrochem Soc, 1997, 144(6): 2045-2052.
[3] COURTNEY A, DAHN J R. Key factors controlling the reversibility of the reaction of lithium with SnO2 and Sn2BPO6 glass[J]. J Electrochem Soc, 1997, 144(9): 2943-2948.
[4] HE Ze-qiang, LI Xin-hai, XIONG Li-zhi, WU Xian-ming, XIAO Zhuo-bing, MA Ming-you. Synthesis and electrochemical properties of tin oxide-based composite by rheological technique[J]. Materials Chemistry & Physics, 2005, 93(2/3): 516-520.
[5] HE Ze-qiang, XIONG Li-zhi, XIAO Zhuo-bing, MA Ming-you, WU Xian-ming. Preparation and electrochemical properties of nano-SnO by sol-gel technique[J]. Chinese Journal of Inorganic Chemistry, 2006, 22(2): 253-257. (in Chinese)
[6] YANG J, WINTER M, BESENHARD J O. Small particle size multiphase Li-alloy anodes for lithium-ion-batteries[J]. Solid State Ionics, 1996, 90(4): 281-287.
[7] BESENHARD J O, YANG J, WINTER M. Will advanced lithium- alloy anodes have a chance in lithium-ion batteries?[J]. J Power Sources, 1997, 68(1): 87-90.
[8] WINTER M, BESENHARD J O, SPAHR M E, NOVAK P. Insertion electrode materials for rechargeable lithium batteries[J]. Adv Mater, 1998, 10(10): 725-763.
[9] LEE J Y, ZHANG R, LIU Z. Lithium intercalation and deinter- calation reactions in synthetic graphite containing a high dispersion of SnO [J]. Electrochem Solid State Lett, 2000, 3(4): 167-170.
[10] READ J, FOSTER D, WOLFENSTINE J, BEHL W. SnO2-carbon composites for lithium-ion battery anodes[J]. J Power Sources, 2001, 96(2): 277-281.
[11] MA Ming-you, HE Ze-qiang, XIONG Li-zhi, LI Xin-hai, XIAO Zhuo-bing, WU Xian-bing, LIU Wen-ping. Preparation and electrochemical properties of SnO2-graphite composites by homogeneous precipitation technique[J]. The Chinese Journal of Nonferrous Metals, 2005, 15(5): 793-798. (in Chinese)
[12] QI Zhi, WU Feng. Nanosized SnO2/graphite composite as negative electrode materials for lithium ion batteries [J]. Chinese Journal of Inorganic Chemistry, 2005, 21(2): 257-260. (in Chinese)
[13] BALAN L, SCHNEIDER R, WILLMANN P, BIUAUD D. Tin-graphite materials prepared by reduction of SnCl4 in organic medium: Synthesis, characterization and electrochemical lithiation[J]. J Power Sources, 2006, 161(1): 587-593.
[14] GUO Z P, WANG J Z, LIU H K, DOU S X. Study of silicon/ polypyrrole composite as anode materials for Li-ion batteries[J]. J Power Sources, 2005, 146(1/2): 448-451.
[15] PASQUIER A, ORSINI F, GOZDZ A S, TARASCON J M. Electrochemical behaviour of LiMn2O4–PPy composite cathodes in the 4-V region[J]. J Power Sources, 1999, 81/82(4): 607-611.
[16] VEERARAGHAVAN B, PAUL J, HALA B, POPOV B. Study of polypyrrole graphite composite as anode material for secondary lithium-ion batteries[J]. J Power Sources, 2002, 109(2): 377-387.
[17] ZHANG Xiang-wu. WANG Chun-sheng, APPLEBY A J, LITTL F E. Improvement in electrochemical properties of nano-tin-polyaniline lithium-ion composite anodes by control of electrode microstructure[J]. J Power Sources, 2003, 109(1): 136-141.
[18] SCHNITZLER D C, MERUVIA M S, HUMMELGEN I A, ZARBIN A J G. Preparation and characterization of novel hybrid materials formed from (Ti, Sn)O2 nanoparticles and polyaniline[J]. Chem Mater, 2003, 15(24): 4658-4665.
[19] HE Ze-qiang, XIONG Li-zhi, MA Ming-you, XIAO Zhuo-bing, WU Xian-ming. Synthesis and characterization of nanometer SnO2 by non-hydrolytic sol-gel approach [J]. Chinese Journal of Inorganic Chemistry, 2005, 21(11): 1691-1696. (in Chinese)
[20] SHENG Yu, CHEN Jian-ding, ZHU De-qin. In-situ chemical synthesis and characterization of conducting polyaniline/manganese dioxide composites[J]. Acta Materiae Compositae Sinica, 2004, 21(4): 1-7. (in Chinese)
[21] FU L J, LIU H, ZHANG H P, LI C, ZHANG T, WU Y P, HOLZE R, WU H Q. Synthesis and electrochemical performance of novel core/shell structured nanocomposites[J]. Electrochemistry Communications, 2006, 8(1): 1-4.
[22] HE Ze-qiang, LI Xin-hai, WU Xian-ming, HOU Zhao-hui, LIU En-hui, DENG Ling-feng, HU Chuang-yue, TIAN Hui-peng. Preparation and electrochemical properties of nanosized tin dioxide electrode material by sol-gel process[J]. Trans Nonferrous Met Soc China, 2003, 13(4): 998-1002.
[23] HE Ze-qiang, LI Xin-hai, XIONG Li-zhi, LIU En-hui, HOU Zhao-hui, WU Xian-ming, DENG Ling-feng. Soft chemical synthesis and electrochemical properties of tin oxide-based materials as anodes for lithium ion batteries[J]. J Cent South Univ Technol, 2004, 11(2): 142-146.
[24] HE Ze-qiang, XIONG Li-zhi, XIAO Zhuo-bing, MA Ming-you, WU Xian-ming. Electrochemical properties of novel calcium stannate anode for lithium ion batteries[J]. Trans Nonferrous Met Soc China, 2005, 15(6): 1420-1424.
[25] MACDONALD J R. Impedance spectroscopy[M]. New York: John Wiley & Sons, 1987: 69.
[26] AURBACH D, EIN-ELI Y, CHUSID O, CARMELI Y, BABAI M, YAMIN H. The correlation between the surface chemistry and the performance of Li-carbon intercalation anodes for rechargeable ‘Rocking-Chair’ type batteries[J]. J Electrochem Soc, 1994, 141(3): 603-610.
(Edited by CHEN Wei-ping)
Foundation item: Project(20376086) supported by the National Natural Science Foundation of China; Project(2005037700) supported by the Postdoctoral Science Foundation of China; Project(07A058) supported by the Scientific Research Fund of Hunan Provincial Education Department; Project(07JJ3014) supported by Hunan Provincial Natural Science Foundation of China
Received date: 2007-06-28; Accepted date: 2007-09-13
Corresponding author: HE Ze-qiang, PhD; Tel: +86-13787930478; E-mail: csuhzq@163.com