
Bioleaching of chalcopyrite by pure and mixed culture
ZHANG Yan-sheng(张雁生), QIN Wen-qing(覃文庆), WANG Jun(王 军), ZHEN Shi-jie(甄世杰),
YANG Cong-ren(杨聪仁), ZHANG Jian-wen(张建文), NAI Shao-shi(赖绍师), QIU Guan-zhou(邱冠周)
Key Laboratory of Biometallurgy of Ministry of Education, School of Minerals Processing and Bioengineering,
Central South University, Changsha 410083, China
Received 20 September 2008; accepted 5 November 2008
Abstract: The bioleaching of chalcopyrite in shake flasks was investigated by using pure Acidithiobacillus ferrooxidans and mixed culture isolated from the acid mine drainage in Yushui and Dabaoshan Copper Mine in China, marked as YS and DB, respectively. The mixed culture consisted mainly of Acidithiobacillus ferrooxidans, Acidithiobacillus thiooxidans, and Leptospirillum spp. (Leptospirillum ferriphilum and Leptospirillum ferrooxians). The results show that the mixed culture is more efficient than the pure Acidithiobacillus ferrooxidans because of the presence of the sulfur-oxidizing cultures that positively increase the dissolution rate and the recovery of copper from chalcopyrite. The pH value decreases with the decrease of chalcopyrite leaching rate, because of the formation of jarosite as a passivation layer on the mineral surface during bioleaching. In the bioleaching using the mixed culture, low pH is got from the sulfur oxidizing inhibiting, the formation of jarosite. The copper extraction reaches 46.27% in mixed culture and 30.37% in pure Acidithiobacillus ferrooxidans after leaching for 75 d.
Key words: chalcopyrite; bioleaching; Acidithiobacillus ferrooxidans; mixed culture
1 Introduction
As an environmentally benign technology with wide applications, biohydrometallurgical process is characterized by low cost for recovering metals from low-grade refractory ores and has received growing attentions due to the increasingly stringent environmental protection regulations[1-3]. Therefore, it is an economical method to recover metals from minerals, especially low-grade ores, overburden, and waste from current mining operations which require moderate capital investment and operating cost[4]. Bioleaching is generally more environmentally friendly than conventional metal recovery processes such as concentration and smelting. Those physicochemical metal extraction processes generate sulfur dioxide, a toxic emission that is increasingly the target of regulatory legislation[5-6].
Microorganisms are important in the bioleaching of the sulfide ores in heap or dump processing commercial application. The most important bacteria are iron- and sulfur-oxidizing Acidithiobacillus ferrooxidans, sulfur-oxidizing Acidithiobacillus thiooxidans and Acidithiobacillus caldus, and iron-oxidizing Leptospirillum spp (Leptospirillum ferriphilum and Leptospirillum ferrooxians)[7-9]. In the processes the temperature is 35-45 ℃. It has been recognized that the dominant microbes were Leptospirillum species and A. caldus in the bioleaching processes operated at 45-50 ℃ [10]. Some research demonstrated that the mixed culture was more efficient than the pure culture alone. And the bacteria have the best adaptability to the ore from which the bacteria were isolated.
Chalcopyrite is the most abundant and refractory copper sulfide. And the bioleaching of chalcopyrite is the main industry target. The slow dissolution rate of chalcopyrite is the main factor hindering commercial application, because the polysulphides, elemental sulfur and iron-hydroxy precipitate layer formed on the surface of chalcopyrite restrict the flow of bacteria, nutrients, oxidants, and reaction products to and from the chalcopyrite surface[4-5, 11].
In this study, the bioleaching of chalcopyrite by pure Acidithiobacillus ferrooxidans and two mixed cultures isolated from acid mine drainage of Yushui and Dabaoshan Copper Mine, marked as YS and DB, respectively, is investigated to determine the culture adaptability to chalcopyrite. The chalcopyrite surface after leaching for several days and the leaching residues are characterized by scanning electron microscopy (SEM), energy dispersive X-ray analysis(EDXA). The bioleaching mechanisms of chalcopyrite by the culture and the role of jarosite are discussed.
2 Experimental
2.1 Culture media and microorganism
The bacteria used in the experiment were A. ferrooxidans type strain ATCC23270 purchased from ATCC and the two mixed culture isolated from the acid mine drainage in Yushui and Dabaoshan Copper Mine in China, which consisted mainly of Acidithiobacillus ferrooxidans, Acidithiobacillus thiooxidans, and Leptospirillum spp. (Leptospirillum ferriphilum and Leptospirillum ferrooxians).
A. ferrooxidans was grown in medium 9K with an initial pH of 2.0. The mixed cultures were maintained in salt medium with sulfur and Fe2+ as the energy source, and adjusted to pH value 2.0 using the sulfuric acid. The 9K medium contained 3 g/L (NH4)2SO4, 0.1 g/L KCl, 0.5 g/L K2HPO4, 0.5 g/L MgSO4·7H2O, 0.01 g/L Ca(NO3)2 and 44.7 g/L FeSO4· 7H2O.
2.2 Mineral
The mineral samples used in the experiments were obtained from Yushui Copper Mine in China, which contained about 96% chalcopyrite and 3.5% secondary cupric sulfide and 0.5% galena. Chemical analyses showed that the ore contained 31.84% Cu, 26.74% Fe, and 31.99% S. The mineral was ground to the particle size of <0.074 mm over 90%. Thin polished slabs of chalcopyrite were prepared by attaching the mineral to the glass slides, cutting thin sections, and then polishing the exposed faces. Several blocks were produced from the slab. The mineral blocks were then washed with acetone and ethanol.
2.3 Bioleaching experiments
The bacterial leaching experiments were carried out in 250 mL Erlenmeyer flasks that were shaken in an air-conditional shaker. The temperature and rotation were constantly maintained at (30±1) ℃ and 160 r/min, respectively. The bacterial activity was monitored by the oxidation rate of Fe2+ and sulfur element. The progress of bioleaching was monitored through the measurement of the concentration of Fe2+, Cu2+, and pH value. The concentration of ferrous irons in the solution was determined by titration with potassium dichromate (K2Cr2O7). The concentration of Cu2+ in the solution was measured using an atomic adsorption spectophometer. In order to determine the mineral solubility, the specific surface area of both the original mineral sample and the leaching residuum was measured. The structural morphology of polished mineral blocks was examined by scanning electron microscopy. The pH value in the leaching solution was measured with a pH-meter (SJ-4A) and was kept constant (pH 2.0) throughout the leaching process using a solution of H2SO4 (4.0 mol/L). Solid samples were also collected, filtered, dried in air and analyzed using SEM and EDXA.
3 Results and discussion
3.1 Activity of bioleaching bacteria
The bacteria were cultured in salts media with energy sources of Fe2+ and S, respectively. The oxidation rate of Fe2+ and the pH value of solution are shown in Figs.1 and 2, respectively. The oxidation rate of Fe2+and S indicates that the oxidation activity of mixed culture is higher than that of the pure culture. Over 95% Fe2+ is oxidized in less than 30 h by mixed culture of Yushui and in 45 h by mixed culture of Dabaoshan, while about 85 h by the pure Acidithiobacillus ferrooxidans. The results in Fig.2 indicate that the mixed culture oxidizes element sulfur more actively than pure Acidithiobacillus ferrooxidans. The final pH value of the media is 1.68, 1.32 and 1.2, respectively after being cultured for 120 h. It is demonstrated that the iron and sulfur oxidation activity of mixed culture is higher than that of the pure culture.
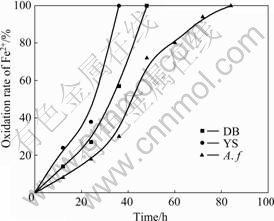
Fig.1 Oxidation rate of Fe2+ by pure culture Acidithiobacillus ferrooxidans and mixed culture of YS and DB in 9K solution at pH 2 and 30 ℃
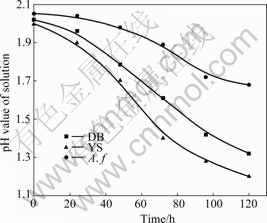
Fig.2 Changes in pH of solution when pure and mixed bacteria were cultured in salts media with element sulfur as energy sources at 30 ℃
3.2 Bioleaching of chalcopyrite in presence and absence of bacteria
The copper extraction of the chalcopyrite leached by mixed culture of YS and in abiotic control is shown in Fig.3. Chalcopyrite is oxidized more deeply by mixed culture than in the abiotic control. The copper leaching rates are 46.27% and 11.5% respectively after being leached for 75 d. The dissolution of chalcopyrite is accelerated by the mixed culture. Therefore, the dissolution rate of chalcopyrite in bioleaching is much higher than that in abiotic control.
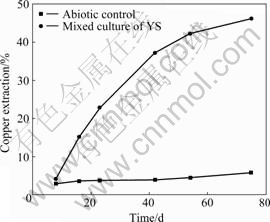
Fig.3 Copper extraction of chalcopyrite leached in mixed culture YS and in abiotic control at initial pH 2 and 30 ℃
3.3 Bioleaching of chalcopyrite by Acidithiobacillus ferrooxidans and mixed culture
The comparison of leaching chalcopyrite at 30 ℃ by pure Acidithiobacillus ferrooxidans and mixed cultures of Yushui and Dabaoshan is shown in Fig.4. The mixed culture oxidizes chalcopyrite more actively than pure culture of Acidithiobacillus ferrooxidans and abiotic controls. As anticipated, the pure culture of Acidithiobacillus ferrooxidans is ineffective in leaching chalcopyrite, with copper extraction of 30.37%, which is lower than that of the mixed culture. Copper extraction after 75 d of incubation reaches 30.37% in pure culture of A. ferrooxidans, 44.68% in mixed culture of Dabaoshan, and 46.27% in mixed culture of Yushui. The presence of sulfur-oxidizing bacteria in the mixed culture significantly increases the dissolution of chalcopyrite. The copper release rate of the pure and mixed culture is accelerated initially but decreased significantly after 40 d of incubation. Fig.5 shows the changes in pH of the solution during leaching of chalcopyrite. The pH value increases in the initial bioleaching, and decreases after leaching for 25 d. The pH value in abiotic control keeps at the level of 1.8-2.0.
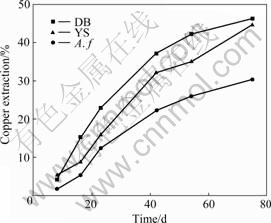
Fig.4 Copper extraction of chalcopyrite leached by A. ferrooxidans and mixed culture at initial pH 2 and 30 ℃
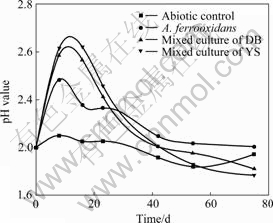
Fig.5 Changes of pH value during leaching of chalcopyrite by A. ferrooxidans and mixed culture at initial pH 2 and 30 ℃
3.4 SEM image of polished chalcopyrite blocks
The SEM images of chalcopyrite surface un-leached and leached with bacteria are shown in Fig.6. The SEM image of the polished chalcopyrite block surface prior to leaching is shown in Fig.6(a). The surface is smooth and clear. The surface of the chalcopyrite leached for 75 d in the abiotic control is not heavily etched as shown in Fig.6(b). Many small stripes are present on the surface, which exhibits insignificant dissolution character. The surface morphology exposed to bacteria is different from that to the abiotic control. As illustrated in Figs.6(c), (d) and (e), the chalcopyrite surfaces leached by the culture show remarkable surface cauterization, especially in the presence of the mixed culture of Yushui (Fig.6(e)). Unlike the small stripes on the abiotic control samples surface, larger consecutive alveolates are observed on the sample surfaces (Fig.6(f)). In addition, large amounts of deposits in the form of porous flocs are seen on the chalcopyrite surfaces after 75 d leaching (Figs.6(d) and (e)). It is illustrated that the bacteria have the best adaptability to the ore obtained from the same place where the bacteria are isolated.
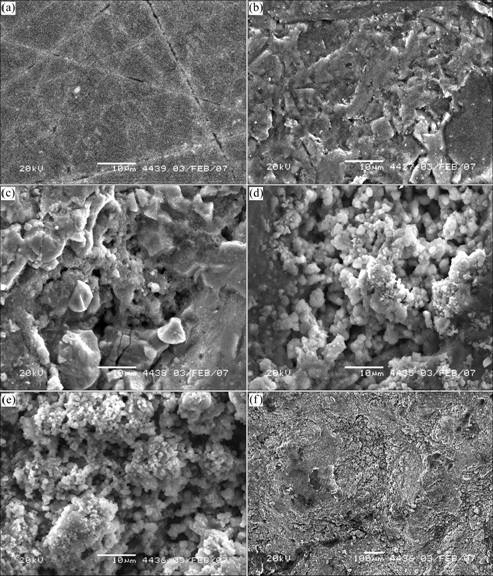
Fig.6 SEM images of chalcopyrite surface (All reactions were at pH 2.0 and 30 ℃): (a) Un-leached; (b) After 75 d reaction in abiotic control; (c) In Acidithiobacillus ferrooxidans pure culture; (d) In mixed culture of DB; (e, f) In mixed culture of YS
3.5 EDXA analysis of chalcopyrite and leaching residues
The chalcopyrite and leaching residues were examined by area analysis of EDXA. The contents of three elements Cu, Fe and S in chalcopyrite and leaching residues are listed in Table 1. The results indicate that S and Fe in the leaching residues are the majority. It is possible that the polysulphides, elemental sulfur layer and the iron-hydroxy precipitate layer are formed on the chalcopyrite surface. The mass fraction of copper decreases from 34.13% to 31.22% and the mole fraction of Cu decreases from 25.34% to 21.98%, indicating that copper is dissolved.
Table 1 Composition of chalcopyrite and leaching residues analysed by EDXA

3.6 Discussion
Chalcopyrite as acid-soluble metal sulfides is probably dissolved by the ferric ions and proton attack [4, 12-14]. Ferric iron oxidizes chalcopyrite to copper and ferrous ions in solution, and elemental sulfur. This reaction is illustrated as follows:
CuFeS2+4Fe3+→Cu2++2S0+5Fe2+ (1)
The ferrous ions formed in the bioleaching reaction are rapidly oxidized in the presence of iron-oxidizing bacteria by the following reaction:
Fe2++2H++0.5O2
2Fe3++H2O (2)
The elemental sulfur formed in the reaction (1) can be oxidized to sulfate in the presence of sulfur-oxidizing bacteria as the following reaction:
2S0+3O2+2H2O
2SO42-+4H+ (3)
Chalcopyrite can be dissolved directly in acid by the following possible reaction[13, 15-16]:
CuFeS2+2H++0.5O2→Cu2++2S+Fe2++H2O (4)
During the leaching processing, ferric ions readily precipitate as basic sulfates such as jarosite by the reaction as follows:
3Fe3++2SO42-+6H2O+K+→KFe(SO4)2(OH)6+6H+ (5)
The acid dissolution (reaction (4)) and ferrous iron oxidation (reaction (2)) processes are acid-consuming reactions, which lead to the increase of culture pH in bioleaching systems. The sulfur oxidization (reaction (3)) and the formation of jarosite (reaction (5)) processes are acid-producing reactions, which contribute to the pH decrease in bioleaching systems. Ferrous iron oxidation is the primary reason for the pH increase in pure A. ferrooxidans and the mixed culture containing A. Ferrooxidans and L. ferriphilum as shown in Fig 5. The more rapid increase of pH in the mixed culture than in the pure culture results from the A. ferrooxidans and L. ferriphilum accelerating the oxidation of ferrous iron to ferric iron. The primary decrease of the pH value relates to the formation of jarosite (reaction (5)). Jarosite forms a passivation layer on the mineral surface to inhibit the dissolution of chalcopyrite. Therefore, the decrease of chalcopyrite leaching rate is accompanied with the decrease of pH value of the solution at the same time after leaching about 25 d.
It is shown in Fig.4 that the copper extraction increases with the decrease of pH value, especially in the mixed culture, indicating that the mixed culture is more efficient than the pure culture. The presence of the sulfur-oxidizing bacteria A. thiooxidans in the mixed culture increases the dissolution rate and the recovery of copper from chalcopyrite through reducing the accumulation of sulfur by transforming it into sulfuric acid[14, 17]. The intermediary sulfur may form a layer on the metal sulfide surface, negatively influencing leaching kinetics.
In the leaching of chalcopyrite, jarosite readily forms in the high Fe3+ concentration at pH 2.0-2.6 and 30 ℃, and the most important factor influencing the formation is pH[18-19]. In pure A. ferrooxidans, jarosite precipitation forms a passivation layer on the mineral surface[5], which strongly inhibits ferric iron reduction followed by a subsequent reduction in the copper leaching rate. While in the mixed culture, the low pH value hinders the formation of jarosite precipitates because of the presence of iron- and sulfur-oxidizing culture. The leaching rate of chalcopyrite is influenced little by the passivation effect of jarosite and does not decrease in the latter leaching period.
4 Conclusions
1) The iron- and sulfur-oxidizing activity of mixed culture is higher than that of the pure A. ferrooxidans.
2) The mixed culture is more efficient than the pure Acidithiobacillus ferrooxidans and positively increases the dissolution rate and the recovery of copper from chalcopyrite because of the presence of sulfur-oxidizing culture in the mixed culture, by which the sulfur is oxidized into sulfuric acid to inhibit the formation of jarosite on the chalcopyrite surface.
3) The mixed bacteria of YS have the best adaptability to the bioleaching of chalcopyrite. The copper extraction achieves 46.27% in mixed culture of YS and 30.37% in pure Acidithiobacillus ferrooxidans.
References
[1] SMITH R W, MISRA M. Recent developments in the bioprocessing of minerals [J]. Mineral Processing and Extractive Metallurgy Review, 1993, 12: 37-60.
[2] BARTLETT R W. Biooxidation heap pretreatment of sulfide refractory gold ore [J]. Mineral, 1996, 16: 89-124.
[3] PRADHAN N, NATHSARMA K C, SRINIVASA RAO K, SUKLA L B, MISHRA B K. Heap bioleaching of chalcopyrite: A review [J]. Minerals Engineering, 2008, 21: 355-365.
[4] WATLING H R. The bioleaching of sulphide minerals with emphasis on copper sulphides—A review [J]. Hydrometallurgy, 2006, 84: 81-108.
[5] STOTT M B, WATLING H R, FRANZMANN P D, SUTTON D. The role of iron-hydroxy precipitates in the passivation of chalcopyrite during bioleaching [J]. Minerals Engineering, 2000, 13(10): 1117-1127.
[6] OLSON G J, BRIERLEY J A, BRIERLEY C L. Bioleaching review (part B): Processing in bioleaching: applications of microbial processes by the minerals industries [J]. Applied Microbiology and Biotechnology, 2003, 63: 249-257.
[7] CORAM N J, RAWLINGS D E. Molecular relationship between two groups of the genus Leptospirillum and the finding that Leptospirillum ferriphilum sp. nov. dominates South African commercial biooxidation tanks that operate at 40 ℃ [J]. Applied and Environmental Microbiology, 2003, 68(2): 838-845.
[8] OKIBE N, GERICKE M, HALLBERG K B, JOHNSON D B. Enumeration and characterization of acidophilic microorganisms isolated from a pilot plant stirred-tank bioleaching operation [J]. Applied and Environmental Microbiology, 2003, 69(4): 1936-1943.
[9] RAWLINGS D E. Characteristics and adaptability of iron- and sulfur-oxidizing microorganisms used for the recovery of metals from minerals and their concentrates [J]. Microbial Cell Factories, 2005, 4: 13.
[10] RAWLINGS D E, CORAM N J, GARDNER M N, DEANE S M. Thiobacillus caldus and Leptospirillum ferrooxians are widely distributed in continuous-flow biooxidation tanks used to treat a variety of metal-containing ores and concentrates [C]// Biohydrometrallurgy and the Environment toward the Mining of the 21st Century, Part A. Amsterdam: Elsevier, 1999: 773-778.
[11] PARKER A, KLAUBER C, KOUGIANOS A, WATLING H R, BRONSWIJK W. An X-ray photoelectron spectroscopy study of the mechanism of oxidative dissolution of chalcopyrite [J]. Hydrometallurgy, 2003, 71: 265-276.
[12] SCHIPPERS A, SAND W. Bacterial leaching of metal sulfide proceeds by two indirect mechanisms via thiosulfate or via polysulfides and sulfur [J]. Applied and Environmental Microbiology, 1999, 65: 319-321.
[13] PADILLA R, PAVEZ P, RUIZ M C. Kinetics of copper dissolution from sulfidized chalcopyrite at high pressures in H2SO4-O2 [J]. Hydrometallurgy, 2008, 91: 113-120.
[14] BEVILAQUA D, LEITE A L L C, GARCIA J O, TUOVINEN O H. Oxidation of chalcopyrite by Acidithiobacillus ferrooxidans and Acidithiobacillus thiooxidans in shake flasks [J]. Process Biochemistry, 2002, 38: 587-592.
[15] C?RDOBA E M, MU?OZ J A, BL?ZQUEZ M L, GONZ?LEZ F, BALLESTER A. Leaching of chalcopyrite with ferric ion (Part I): General aspects [J]. Hydrometallurgy, 2008, 93: 81-87.
[16] ROHWERDER T, GEHRKE T, KINZLER K, SAND W. Bioleaching review (part A): Progress in bioleaching: fundamentals and mechanisms of bacterial metal sulfide oxidation [J]. Applied Microbiology and Biotechnology, 2003, 63: 239-248.
[17] SEMENZA M, VIERA M, CURUTCHET G., DONATI E. The role of Acidithiobacillus caldus in the bioleaching of metal sulfides [J]. Latin American Applied Research, 2002, 32(4): 303-306.
[18] KINNUNEN P H M, HEIMAL S, RIEKKOLA-VANHANEN M L, PUHAKKA J A. Chalcopyrite concentrate leaching with biologically produced ferric sulphate [J]. Bioresource Technology, 2006, 97: 1727-1734.
[19] FOUCHERA S, BRUNETA F B, D′HUGUESA P, CLARENSB M, GODONC J J, MORIN D. Evolution of the bacterial population during the batch bioleaching of a cobaltiferous pyrite in a suspended solids bubble column and comparison with a mechanically agitated reactor [J]. Hydrometallurgy, 2003, 71: 5-12.
Foundation item: Project(50621063) supported by the National Natural Science Foundation of China; Project(2004CD619205) supported by the National Basic Research Program of China; Project(2007AA060902) supported by the High-tech Research and Development Program of China
Corresponding author: QIN Wen-qing; Tel: +86-731-8830346; E-mail: qingwenqing1@126.com
(Edited by YUAN Sai-qian)